Mcm10 promotes rapid isomerization of CMG-DNA for replisome bypass of lagging strand DNA blocks
Figures
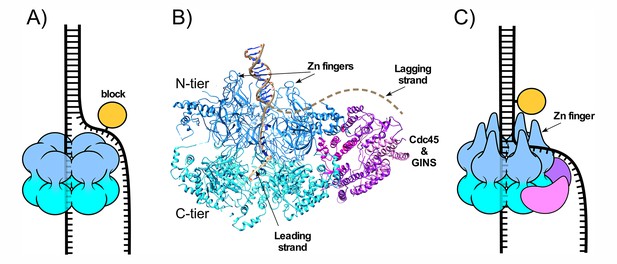
CMG-fork structure and isomerization needed for bypass of lagging strand blocks.
(A) Illustration of a hexameric helicase in the steric exclusion mode, encircling the tracking strand and excluding the non-tracking strand from the central channel. (B) CMG-forked DNA structure (PDB ID# 5U8S). Duplex DNA penetrates the zinc fingers defining the start of the central channel in the N-tier and the unwinding point is internal to the central channel (Georgescu et al., 2017). The Mcm2-7 subunits are shown in two shades of blue to highlight the N- and C-tiers. The lagging strand tail is present but not visible, indicating mobility, and is suggested to exit the central channel though a gap between two zinc fingers (dashed line) (see also panel C). (C) Illustration of CMG encountering a lagging strand block in the modified steric exclusion mode, partially encircling both strands of the duplex DNA as shown in (B). To bypass the block, CMG-DNA must isomerize to the classic steric exclusion mode in (A) or by opening of the Mcm2-7 ring during bypass (illustrated in Figure 8 of Langston and O'Donnell, 2017).
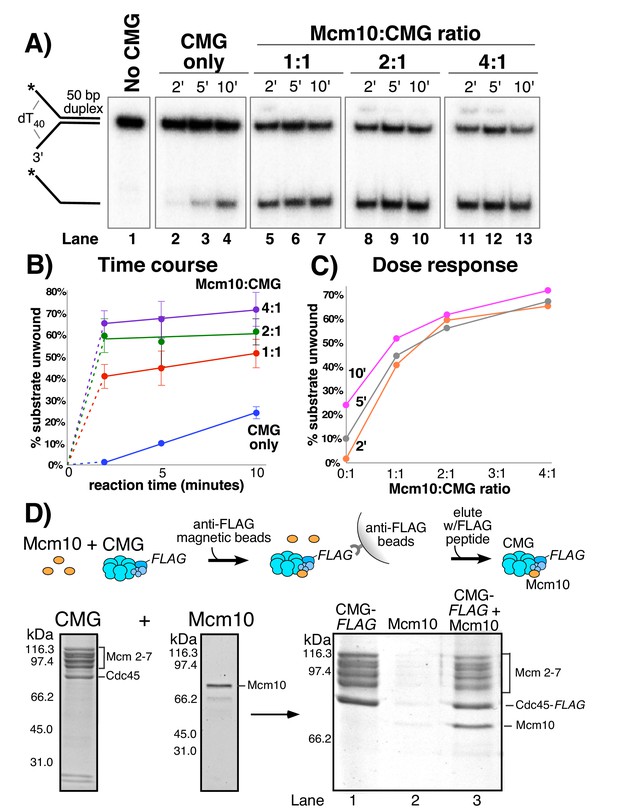
Mcm10 binds CMG and stimulates its helicase activity.
(A) Titration of Mcm10 in a CMG unwinding assay. Reactions contained 25 nM CMG with either: no Mcm10 (lanes 2–4), 25 nM Mcm10 (1:1, lanes 5–7), 50 nM Mcm10 (2:1, lanes 8–10) or 100 nM Mcm10 (4:1, lanes 11–13). See Materials and methods for details. Also see Figure 2—figure supplements 1–2. (B) Quantification of the data from (A). Values are the average of three independent experiments and the error bars show the standard deviation. (C) The same data displayed as a dose-response curve of unwinding as a function of the Mcm10:CMG ratio in the reaction at the three different time points. (D) Isolation of CMG-Mcm10 complex using CMG-Flag and anti-Flag beads. CMG and a 3-fold molar excess of Mcm10 were mixed, attached to beads, then washed twice and eluted with Flag peptide. The PAGE gel shows elution from the anti-Flag beads that were loaded with either CMG-flag alone (lane 1), Mcm10 alone (lane 2), or the CMG-flag +Mcm10 mixture (lane 3). The protein bands are identified to the right. A densitometric scan of the bands in the gel of the CMG-Mcm10 complex indicates a molar ratio of 1.8 Mcm10 per Mcm2-7 complex; the Cdc45 is typically in excess due to the Flag tag (i.e. any dissociated Cdc45 will bind the beads). The GINS subunits ran off the gel (see Figure 2—figure supplements 3–4 for GINS stoichiometry). Also see Figure 2—figure supplement 5 for helicase activity of purified CMG-Mcm10 complex.
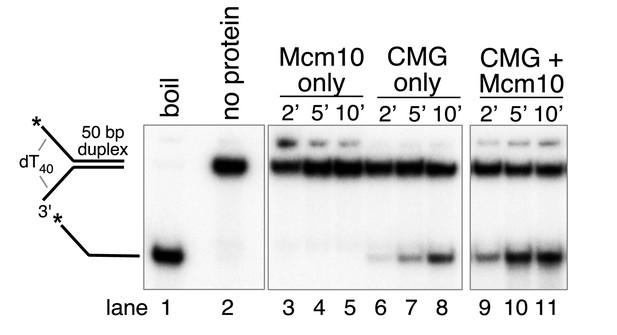
Mcm10 does not have helicase activity.
Helicase assays were performed as in Figure 2 using Mcm10 only (lanes 3–5), CMG only (lanes 6–8) or CMG + Mcm10 (lanes 9–11).
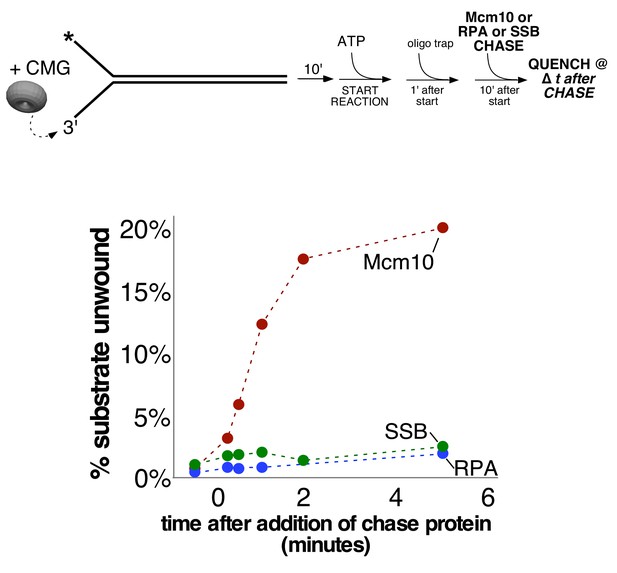
Neither yeast RPA nor E. coli SSB stimulates CMG helicase.
As shown in the schematic at the top, CMG (20 nM) was pre-incubated with the 160-mer duplex substrate from Figure 3B (0.5 nM) for 10’ followed by addition of ATP (1 mM) to start the CMG unwinding reaction. 1’ after adding ATP, unlabeled trap oligo (20 nM) was added to the reaction to prevent re-annealing of any unwound strands. 10’ after addition of ATP, either Mcm10 (red), yeast RPA (blue), or E. coli SSB (green) (‘chase’ protein, 80 nM) was added to the reaction to determine whether it stimulates CMG unwinding. 30’ prior to addition of the chase protein, an aliquot of each reaction was withdrawn to determine the extent of CMG unwinding prior to addition of the chase protein. Time points were also taken 15’, 30’, 1’, 2’ and 5’ after addition of the chase protein, analyzed by gel electrophoresis and quantified as previously described.
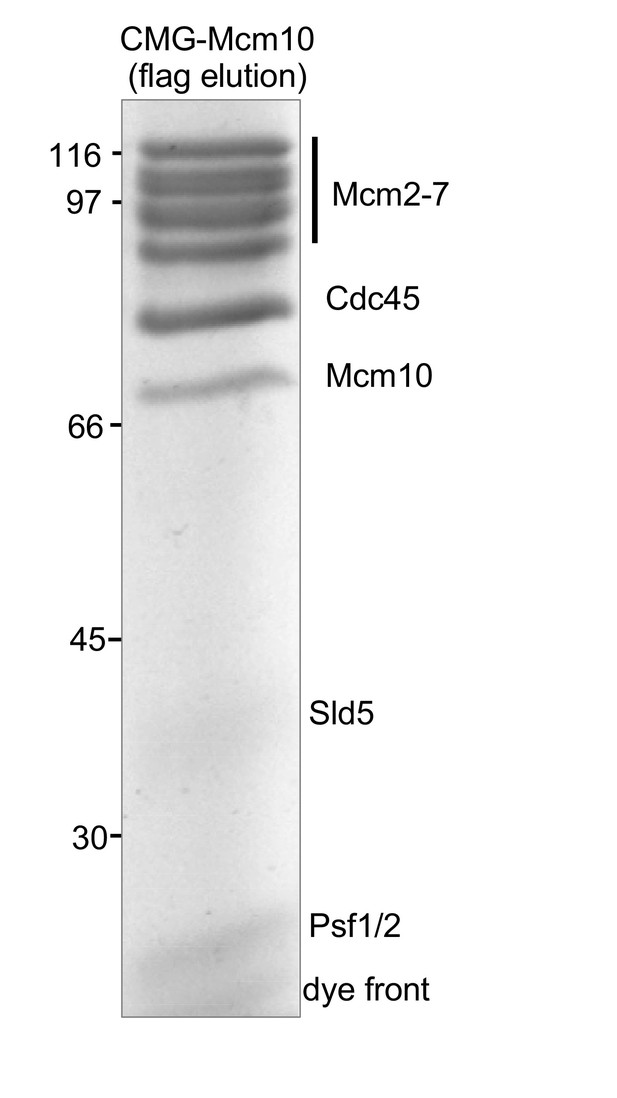
SDS PAGE of flag bead eluted CMG-Mcm10.
To determine the stoichiometry of GINS in the CMG-Mcm10 complex, a Flag purification of CMG-Mcm10 was analyzed in a 10% PAGE gel followed by densitometric analysis. The result indicates the following stoichiometry: 1.0 Mcm2-7, 1.2 Mcm10, 0.87 Sld5, and 2.6 Psf1 + Psf2 (they co-migrate for an average of 1.3 each). The Psf3 runs at the dye front and could not be quantitated. FLAG columns capture some free Cdc45 and thus overestimate the Cdc45-FLAG subunit; MonoQ analysis removes free Cdc45 (see Figure 2—figure supplement 4).
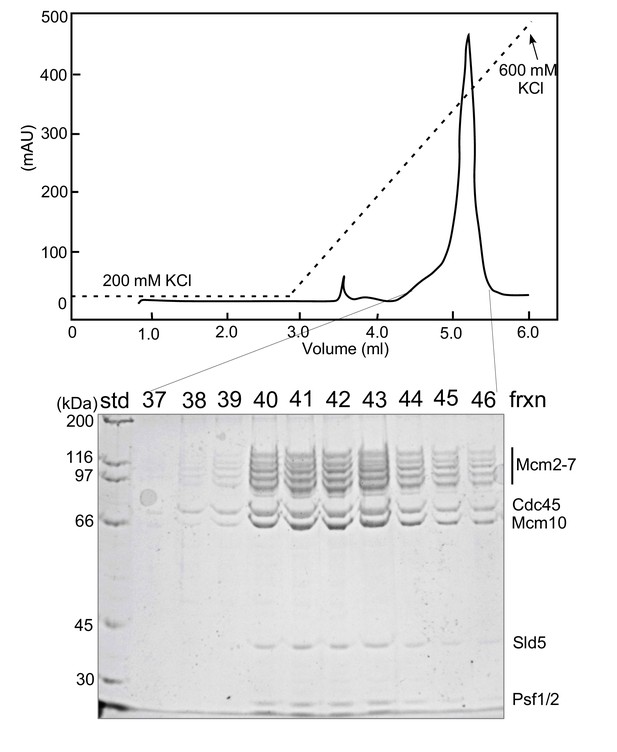
MonoQ reconstitution of CMG/Mcm10 complex.
To form a CMG-Mcm10 complex, 600 μg purified CMG (765 pmol) was mixed with 250 μg purified Mcm10 (3.1 nmol). The mixture was incubated on ice for 30’ and spun in a microcentrifuge at 15,000 rpm for 3’ at 4°C to remove any precipitated proteins. The CMG/Mcm10 mixture was injected at 0.1 ml/min onto a 100 μl monoQ column that had previously been equilibrated in a buffer consisting of 20 mM Tris-Cl pH7.5, 200 mM KCl, 2 mM DTT and 2 mM MgCl2. The column was washed and then eluted in the same buffer using a 2.5 ml gradient from 200 mM to 600 mM KCl, collecting 0.1 ml fractions. Peak fractions were analyzed in an 8% SDS-polyacrylamide gel stained with Denville Blue. A densitometric scan of the gel showed a molar ratio of 1.0 Mcm2-7: 1.59 Cdc45: 2.0 Mcm10: 1.4 Sld5: Psf1 and Psf2 at 1.05 each (Psf1/2 co-migrate, and together give a stoichiometry of 1.0:2.1 for Mcm2-7: Psf1 +Psf2); Psf3 runs at the dye front.
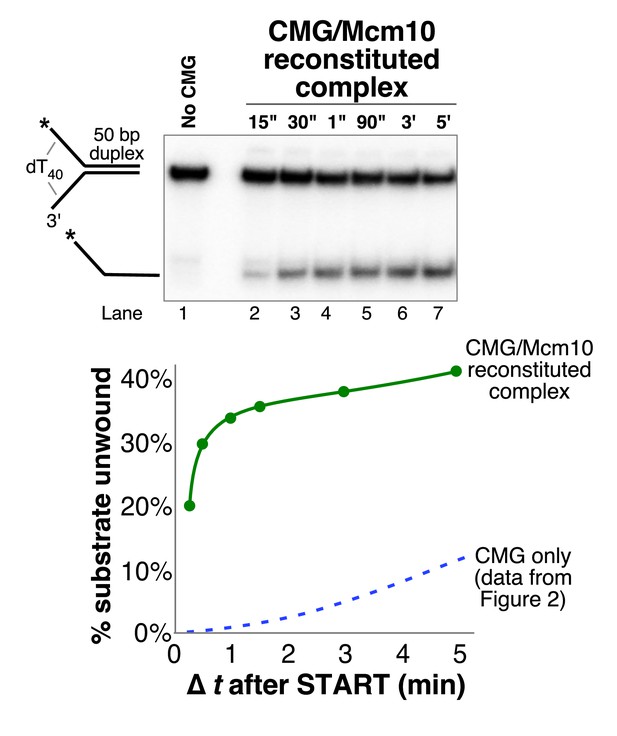
The CMG-Mcm10 complex reconstituted on MonoQ is functional.
30 nM (final concentration) of reconstituted CMG-Mcm10 complex (Figure 2—figure supplement 4) was preincubated with the 50 bp duplex fork substrate (0.5 nM) for 10’ in the absence of ATP in buffer containing 20 mM Tris Acetate pH 7.6, 5 mM DTT, 0.1 mM EDTA, 10 mM MgSO4, 30 mM KCl, 40 μg/ml BSA. The reaction was started by addition of 1 mM ATP and 40’ later unlabeled 50duplex LAG oligo was added (to 50 nM) as a trap for unwound DNA. The total reaction volume was 78 μl and at the indicated times, 11 μl aliquots were removed, stopped with buffer containing 20 mM EDTA and 0.1% SDS (final concentrations), and flash frozen in liquid nitrogen. Frozen reaction products were thawed quickly in room temperature water and separated on 10% native PAGE minigels. Gels were washed in distilled water, mounted on Whatman 3 MM paper, wrapped in plastic and exposed to a storage phosphor screen that was scanned on a Typhoon 9400 laser imager (GE Healthcare). The scanned gel was analyzed using ImageQuant TL v2005 software to obtain the quantitations shown in the graph below the gel.
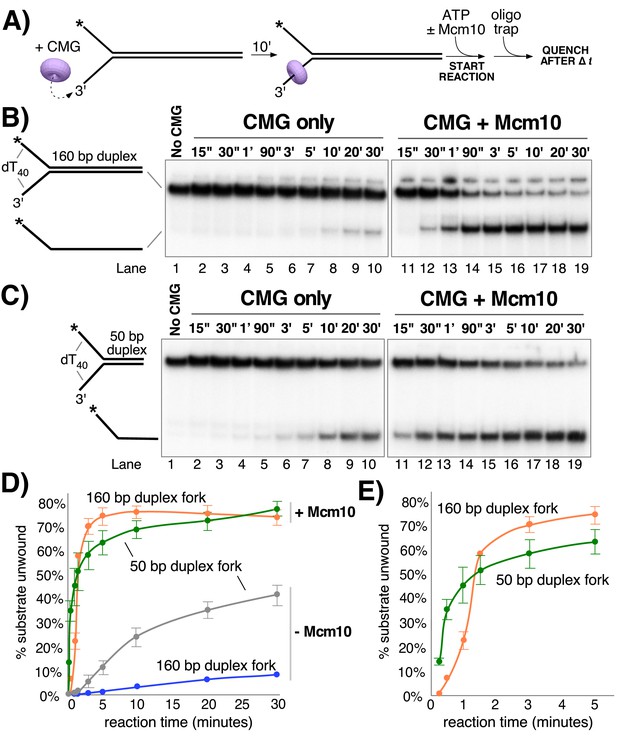
Mcm10 enhances the processivity of CMG unwinding.
(A) Scheme of the reaction. CMG was pre-incubated with the substrates for 10’ before addition of ATP ± a 2-fold excess of Mcm10 over CMG. (B) Native PAGE analysis of CMG unwinding in the absence (lanes 2–10) or presence (lanes 11–19) of Mcm10 using either a 160 bp duplex fork (panel B) or a 50 bp duplex fork (panel C) as indicated by the schematics to the left of the gels. (D) Quantification of the data in the gels. (E) The first 5’ of reactions with Mcm10 are shown to better illustrate the effect of Mcm10 on unwinding by CMG. The values are averages of three independent experiments and the error bars show the standard deviation. Also see Figure 3—figure supplement 1.
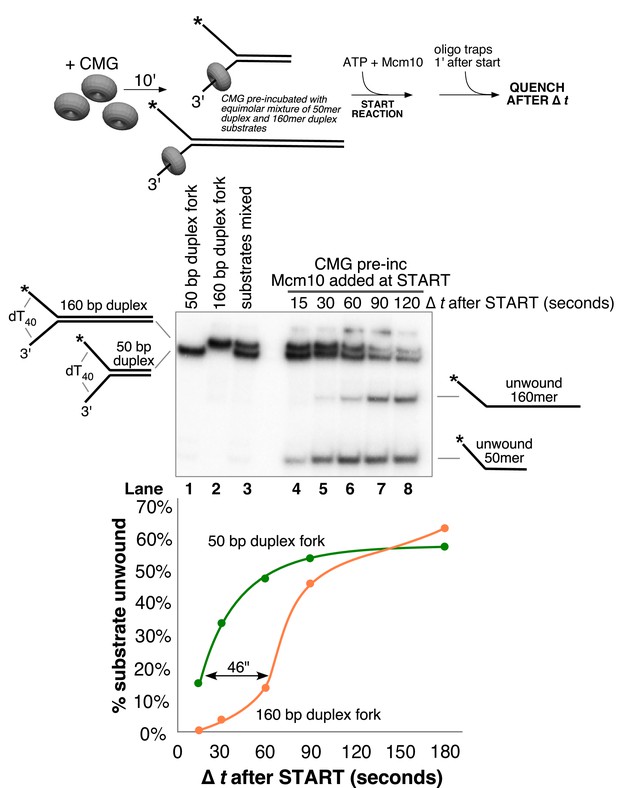
Delayed replication of longer duplex allows estimation of unwinding rate.
A helicase assay identical to those in Figure 3 was performed except that both the 50 bp duplex and 160 bp duplex for substrates were added to the reaction at 0.5 nM each substrate and pre-incubated together with 20 nM CMG in the absence of ATP as shown in the scheme above the gel. The reaction was started by the addition of ATP (1 nM) and Mcm10 (40 nM) and unlabeled traps for each substrate (25 nM) were added 1’ later to prevent re-annealing of unwound DNA. The total reaction volume was 91 μl and at the indicated times, 11 μl aliquots were removed, stopped with buffer containing 20 mM EDTA and 0.1% SDS (final concentrations), and flash frozen in liquid nitrogen. The positions of the two substrates are indicated in lanes 1–3 of the gel showing that they migrate at distinguishable positions. Unwinding of each substrate was calculated as [unwound/(unwound + unreacted substrate)] for each substrate separately and the values are shown in the graph below the gel.
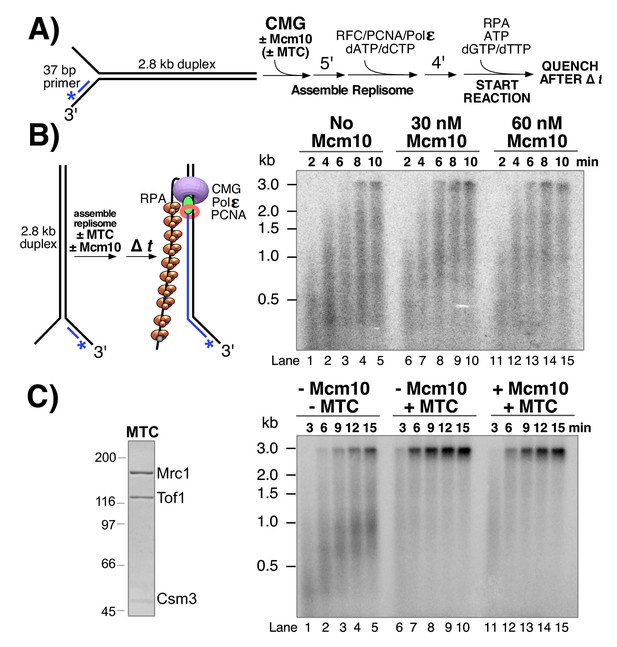
Mcm10 stimulates CMG-dependent leading strand replication in the absence of Mrc1-Tof1-Csm3.
(A) Reaction scheme. See Materials and methods for details. (B) Alkaline agarose gel of leading strand products synthesized by the core leading strand replisome with the indicated amounts of Mcm10. (C) Purified MTC complex is shown to the left. Numbers to the left of the SDS PAGE gel show the migration of molecular weight markers (kDa). Time courses, to the right, are the core leading strand replisome with or without Mcm10 and Mrc1-Tof1-Csm3 complex (MTC) as indicated above the gel.
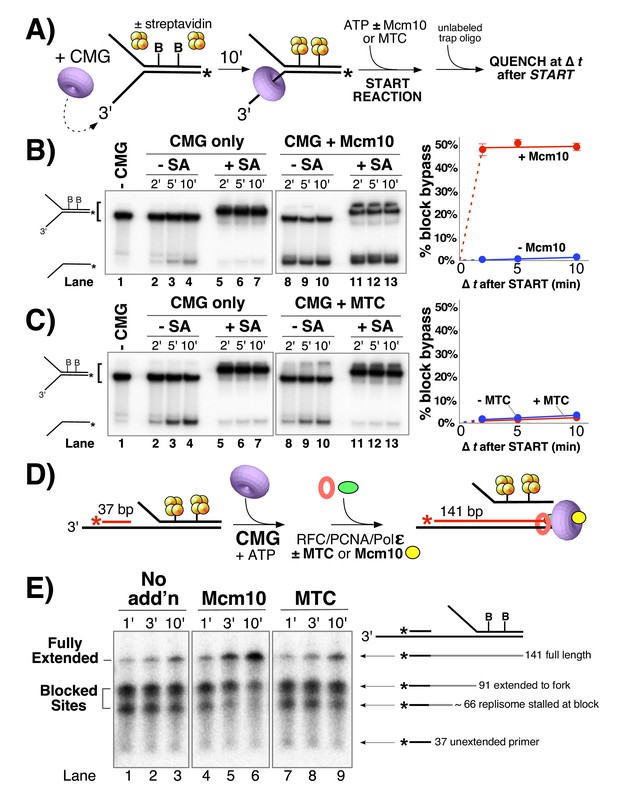
Mcm10, but not MTC complex, enables CMG and the replisome to bypass a lagging strand block.
(A) Illustration of the helicase reaction scheme. See Materials and methods for details. (B) Unwinding assays on dual biotinylated forks with or without streptavidin and with or without Mcm10, as indicated. (C) Same as in panel B except reactions contained or omitted MTC complex instead of Mcm10. (D) Illustration of the replisome reaction scheme. Reactions contained a synthetic minifork with 32P-5’ end-labeled primer and a dual biotin-streptavidin (SA) block on the lagging strand template. CMG and ATP were added for 3 min to allow CMG to bind DNA and translocate to the SA blocks, then Pol ε, RFC and PCNA were added (±MTC) along with all four dNTPs to assemble and extend the primer up to the blocked CMG, followed by addition of Mcm10 (or no protein). See Materials and methods for details. (E) Timed aliquots after ± Mcm10 addition were analyzed in a 10% urea PAGE gel. The primer, two stalled products and the full-length product are indicated to the right of the gel. Also see Figure 5—figure supplement 1.
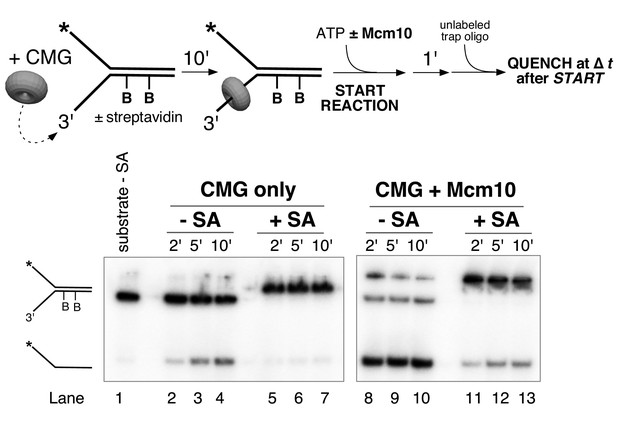
A leading strand block inhibits CMG unwinding even in the presence of Mcm10.
Helicase reactions were performed as in Figure 5B except that the substrate contained a dual biotin-streptavidin block on the leading strand (50duplex LEAD2 dual biotin); the lagging strand (50duplex LAG2) was radiolabeled. Oligo sequences are in Table 1.
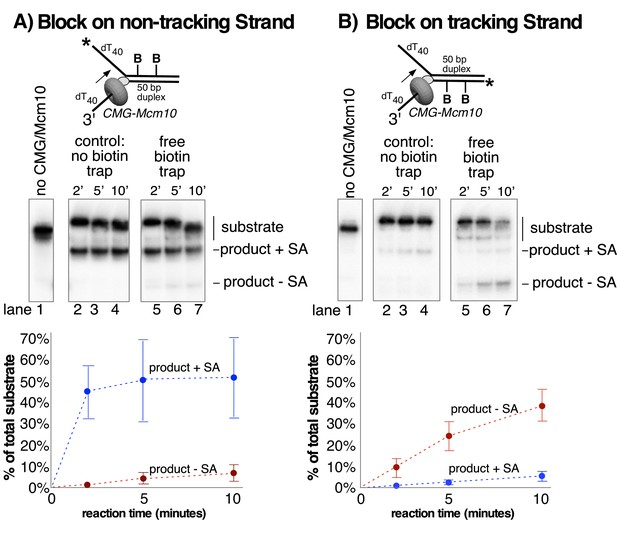
Lagging strand blocks are not displaced from DNA during unwinding by CMG-Mcm10.
(A) The helicase reaction in Figure 5B (CMG + Mcm10) was repeated with the radiolabel on the biotinylated lagging strand and in the presence of free biotin as a trap for displaced streptavidin (See Materials and methods for details). Unwound strands are shifted in the gel when bound to streptavidin (product +SA) compared to unbound (product – SA). Lanes 2–4 are a control in the absence of biotin trap showing that all of the unwound products migrate in the gel shifted position. The biotin trap is present in the experiment in lanes 5–7. A shift in migration of the unwound products indicates displacement of streptavidin by CMG-Mcm10, but the result shows that almost all the unwound DNA retained the streptavidin. (B) The experiment in (A) was repeated with a substrate in which the dual biotin blocks were on the radiolabeled leading strand. Also see Figure 6—figure supplement 1.
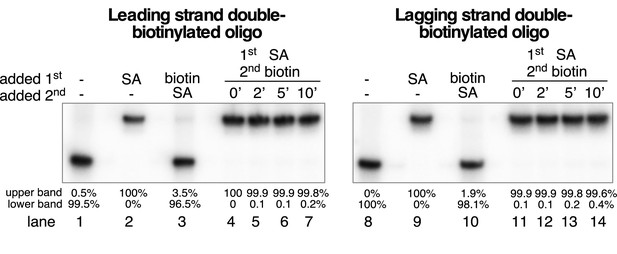
Free biotin prevents streptavidin binding to the biotinylated oligos but does not displace pre-bound streptavidin.
To test the effectiveness of the free biotin trap used in Figure 6, the radiolabeled, dual-biotinylated oligos (0.5 nM) were incubated with streptavidin (4 μg/ml) and biotin (1.5 μM) was added before (lanes 3 and 10) or after (lanes 4–7 and 11–14) streptavidin incubation. Lanes 1/8 and lanes 2/9 are controls showing the migration of the substrates in the absence and presence of streptavidin, respectively, with no biotin addition. Lanes 3 and 10 demonstrate the effectiveness of the free biotin trap in preventing streptavidin binding to the substrates. Lanes 4–7 and 11–14 show that when the substrates are pre-incubated with streptavidin for 5’ at 30° C, addition of the biotin trap does not disrupt the pre-bound streptavidin over the 10’ time course used in the helicase assays in Figure 6. Below the gels is quantitation of the upper and lower bands in each lane of the gel by densitometry as described previously.
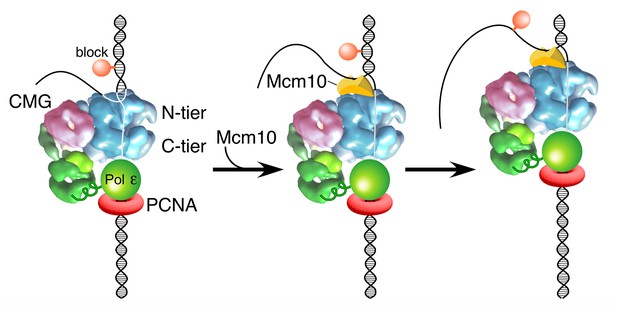
Model of Mcm10 function.
Proposed model of Mcm10 mediated isomerization of CMG-DNA at a replication fork. Left, CMG encounters an impediment on the DNA but cannot pass it because it surrounds dsDNA in the N-terminal tier of Mcm2-7 (as in Figure 1C); middle, Mcm10 binds to the forked junction and to the N-terminal tier of CMG, releasing CMG’s grip on the duplex DNA and placing CMG in a steric exclusion mode encircling ssDNA (as in Figure 1A); right, with the lagging strand freed from the interior of the Mcm2-7 ring, CMG can bypass the impediment on the DNA and proceed. Alternative isomerization reactions that could also accomplish bypass of a bulky block are discussed in the text.
Tables
Oligonucleotides Used in this Study.
All oligonucleotides used in this study were ordered from IDT with the indicated modifications.
Oligo name | Sequence (5’ to 3’) | Modification(s) |
---|---|---|
50dupex LAG | TTTTTTTTTTTTTTTTTTTTTTTTTTTTTTTTTTTTTTTTGACGCTGCCGAATTCTGGCTTGCTAGGACATTACAGGATCGTTCGGTCTC | None |
50duplex LAG dual biotin | TTTTTTTTTTTTTTTTTTTTTTTTTTTTTTTTTTTTTTTTGACGCTGCCGAATTCTGGCTTGCTAGGACATTACAGGATCGTTCGGTCTC | Two biotin-modified thymidine residues in BOLD |
50duplex LEAD | GAGACCGAACGATCCTGTAATGTCCTAGCAAGCCAGAATTCGGCAGCGTCTTTTTTTTTTTTTTTTTTTTTTTTTTTTTTTTTT*T*T*T*T*T*T | The six dT residues at the 3’ end are connected by phosphorothioate bonds (*) |
50duplex LAG2 | TTTTTTTTTTTTTTTTTTTTTTTTTTTTTTTTTTTTTTTTGACGCTGCCGAATTCTGGATTGCTAGGACATTACAGGATCGTTCGGTCTC | None |
50duplex LEAD2 dual biotin | GAGACCGAACGATCCTGTAATGTCCTAGCAATCCAGAATTCGGCAGCGTCTTTTTTTTTTTTTTTTTTTTTTTTTTTTTTTTTT*T*T*T*T*T*T | Two biotin-modified thymidine residues in BOLD; the six dT residues at the 3’ end are connected by phosphorothioate bonds (*) |
160mer duplex LEAD | AGAGAGTAGAGTTGAGTTGTGATGTGTAGAGTTGTTGTAGAGAAGAGTTGTGAAGTGTTGAGTAGAGAAGAGAAGAGAAGTGTTGTGATGTGTTGAGTAGTGTAGAGTTGAGAAGTAGAGATGTGTTGAGATGAGAAGAGTTGTAGTTGAGTTGAAGTGGTTTTTTTTTTTTTTTTTTTTTTTTTTTTTTTTTTT*T*T*T*T*T | The five dT residues at the 3’ end are connected by phosphorothioate bonds (*) |
160mer duplex LAG | TTTTTTTTTTTTTTTTTTTTTTTTTTTTTTTTTTTTTTTTCCACTTCAACTCAACTACAACTCTTCTCATCTCAACACATCTCTACTTCTCAACTCTACACTACTCAACACATCACAACACTTCTCTTCTCTTCTCTACTCAACACTTCACAACTCTTCTCTACAACAACTCTACACATCACAACTCAACTCTACTCTCT | None |
Blocked Fork LEAD | ACCGGAGACCGAACGATCCTGTAATGTCCTAGCAAGCCAGAATTCGGCAGCGTCTTTTTTTTTTTTTTTTTTTTTTTTTTTTTTTTTTTTTTTTGAGGAAAGAATGTTGGTGAGGGTTGGGAAGTGGAAGGATGGGCTCGAGAGGTTTTTTTTTTTTTTTTTTTTTTTTTTTTT*T*T*T*T*T | The five dT residues at the 3’ end are connected by phosphorothioate bonds (*) |
Blocked Fork LAG | TTTTTTTTTTTTTTTTTTTTTTTTTTTTTTTTTTTTTTTTTTTTTTTTTTGACGC TGCCGAATTCTGGCTTGCTAGGACATTACAGGATCGTTCG*G*T*C*T*C | Two biotin-modified thymidine residues in BOLD; the five dT residues at the 3’ end are connected by phosphorothioate bonds (*) |
Blocked Fork Primer | CCTCTCGAGCCCATCCTTCCACTTCCCAACCCTCACC | None |
C2 | CCTCTCGAGCCCATCCTTCCACTTCCCAACCCTCACC | None |
Additional files
-
Transparent reporting form
- https://doi.org/10.7554/eLife.29118.018