Mechanotransduction: Two views of the same stimulus
The ability of sensory neurons to detect and respond to mechanical force using a process known as mechanotransduction allows us to interpret and navigate the physical world around us. Although a number of different proteins have been linked to mechanotransduction, how they interact with each other or with other signaling pathways in a single sensory neuron is still not well understood.
The study of mechanotransduction has focused primarily upon ion channels that span cell membranes and open in response to mechanical perturbation. In most cases, the channel protein itself is the mechanosensor, responding either to changes in the physical properties of the cell membrane or the tension in a molecular anchor within the cell (Ranade et al., 2015). This promotes the false impression that each type of channel operates independently. However, individual sensory neurons normally express a variety of ion channels and other proteins that allow them to respond to many different signals, such as touch, chemicals, and both hot and cold temperatures (Geffeney and Goodman, 2012; Abraira and Ginty, 2013).
Recent studies have begun to unravel the complex relationship between mechanosensory channels and their environment inside a single neuron. For example, some channels have been shown to interact with members of a superfamily of membrane proteins called the G-protein coupled receptors (GPCRs). The binding of an external signal molecule (ligand) to a GPCR stimulates signaling pathways inside the cell that are involved in a large variety of processes. Furthermore, some of the components in these pathways can interact with mechanosensory channels in response to pain or inflammation (Geppetti et al., 2015; Veldhuis et al., 2015).
All GPCRs contain a seven transmembrane domain embedded within the cell membrane, along with one or more domains inside the cell that trigger the downstream signaling pathways. Members of a subgroup known as the adhesion GPCRs also contain an unusual extracellular domain (ECD) that is thought to interact with components of the extracellular matrix, a scaffold-like structure that surrounds cells to provide structural support (Langenhan et al., 2016)
The ECD is linked to the transmembrane domains by another domain that allows some adhesion GPCRs to cut themselves into two pieces. It has been assumed that this “autoproteolysis” step, which splits the ECD away from the rest of the protein, is essential to activate adhesion GPCRs. Recent studies suggest that some adhesion GPCRs may detect mechanosensory information through the ECD when it is tethered to the extracellular matrix (Petersen et al., 2015; Scholz et al., 2015).
In 2015, a team of researchers led by Robert Kittel and Tobias Langenham at the University of Würzberg reported that an adhesion GPCR called dCIRL may influence the activity of the NOMPC mechanosensory channel in the chordotonal organ of fruit fly larvae (Scholz et al., 2015). However, it was not clear whether the two proteins directly interact with each other. Now, in eLife, Kittel, Langenham and co-workers – including Nicole Scholz as first author – report that dCIRL may modulate the activity of NOMPC by stimulating signaling pathways inside the neuron (Scholz et al., 2017).
The chordotonal organ plays crucial roles in a range of mechanosensory processes in fruit fly larvae, and Scholz et al. found that dCIRL (also known as latrophillin) and NOMPC co-localize to the same structures within the neurons in this organ. Mechanical stimuli trigger weaker responses in mutant larvae that are unable to produce dCIRL than they do in normal larvae. In addition, changing the length of the ECD modified the response of dCIRL to mechanical stimuli consistent with an essential role for the ECD in transducing the signal. Together, these results suggest that dCIRL interacts with unidentified ligands outside of the cell to modulate the activity of NOMPC and adjust how strongly a neuron responds to a mechanical stimulus.
Unlike the adhesion GPCRs studied in other animals, dCIRL does not require autoproteolysis to be correctly localized or activated in neurons. Moreover, dCIRL also appears to use different signaling pathways because it decreases the level of a signal molecule called cAMP in cells, whereas the adhesion GPCRs in other animals have the opposite effect (Müller et al., 2015). By using different signaling pathways, the various members of this GPCR subgroup may play different roles in different cell types or species.
The findings of Scholz et al. provide a potential process by which multiple signaling events (such as the complementary mechanical inputs from NOMPC and dCIRL) can interact within a single sensory neuron to precisely modulate the neuron's response to a mechanical perturbation (Figure 1). The diversity of the adhesion GPCR proteins across different cell types and species supports the hypothesis that the way adhesion GPCRs modulate mechanotransduction may also depend upon the type of mechanical signal they receive.
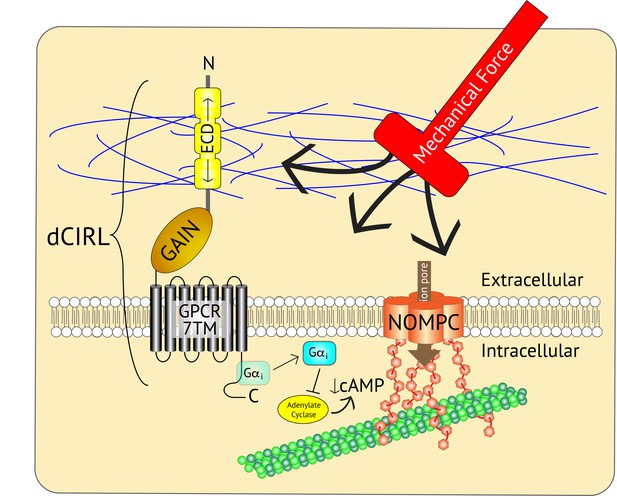
Adjusting the mechanosensory response of sensory neurons.
The ion channel NOMPC (orange) is a transmembrane protein that is anchored to the neuron’s cytoskeleton (green circles), allowing it to detect mechanical force. This stimulates the pore of the channel (brown arrow) to open, leading to the generation of an electrical signal within the neuron. At the same time, the extracellular domain (ECD) of another transmembrane protein, the adhesion GPCR dCIRL, detects the mechanical force differently as a result of being anchored to the extracellular matrix (blue strings). Activation of dCIRL decreases the level of cAMP in the cell, possibly due to the activation of a G protein (Gαi) that inhibits the enzyme that makes cAMP (known as adenylate cyclase). Unidentified signaling components downstream of cAMP alter NOMPC activity to modulate the strength of the overall response. In this manner, both extracellular and intracellular views of the same stimulus are combined to precisely adjust the neuronal response. GAIN, GPCR autoproteolytic domain. 7TM: seven transmembrane domain.
References
-
Adhesion G protein-coupled receptors in nervous system development and diseaseNature Reviews Neuroscience 17:550–561.https://doi.org/10.1038/nrn.2016.86
Article and author information
Author details
Publication history
Copyright
© 2017, Johnson
This article is distributed under the terms of the Creative Commons Attribution License, which permits unrestricted use and redistribution provided that the original author and source are credited.
Metrics
-
- 2,131
- views
-
- 213
- downloads
-
- 5
- citations
Views, downloads and citations are aggregated across all versions of this paper published by eLife.
Download links
Downloads (link to download the article as PDF)
Open citations (links to open the citations from this article in various online reference manager services)
Cite this article (links to download the citations from this article in formats compatible with various reference manager tools)
Further reading
-
- Neuroscience
Dynamic interactions between large-scale brain networks underpin human cognitive processes, but their electrophysiological mechanisms remain elusive. The triple network model, encompassing the salience network (SN), default mode network (DMN), and frontoparietal network (FPN), provides a framework for understanding these interactions. We analyzed intracranial electroencephalography (EEG) recordings from 177 participants across four diverse episodic memory experiments, each involving encoding as well as recall phases. Phase transfer entropy analysis revealed consistently higher directed information flow from the anterior insula (AI), a key SN node, to both DMN and FPN nodes. This directed influence was significantly stronger during memory tasks compared to resting state, highlighting the AI’s task-specific role in coordinating large-scale network interactions. This pattern persisted across externally driven memory encoding and internally governed free recall. Control analyses using the inferior frontal gyrus (IFG) showed an inverse pattern, with DMN and FPN exerting higher influence on IFG, underscoring the AI’s unique role. We observed task-specific suppression of high-gamma power in the posterior cingulate cortex/precuneus node of the DMN during memory encoding, but not recall. Crucially, these results were replicated across all four experiments spanning verbal and spatial memory domains with high Bayes replication factors. Our findings advance understanding of how coordinated neural network interactions support memory processes, highlighting the AI’s critical role in orchestrating large-scale brain network dynamics during both memory encoding and retrieval. By elucidating the electrophysiological basis of triple network interactions in episodic memory, our study provides insights into neural circuit dynamics underlying memory function and offer a framework for investigating network disruptions in memory-related disorders.
-
- Neuroscience
Astrocytes derive from different lineages and play a critical role in neuropathic pain after spinal cord injury (SCI). Whether selectively eliminating these main origins of astrocytes in lumbar enlargement could attenuate SCI-induced neuropathic pain remains unclear. Through transgenic mice injected with an adeno-associated virus vector and diphtheria toxin, astrocytes in lumbar enlargement were lineage traced, targeted, and selectively eliminated. Pain-related behaviors were measured with an electronic von Frey apparatus and a cold/hot plate after SCI. RNA sequencing, bioinformatics analysis, molecular experiment, and immunohistochemistry were used to explore the potential mechanisms after astrocyte elimination. Lineage tracing revealed that the resident astrocytes but not ependymal cells were the main origins of astrocytes-induced neuropathic pain. SCI-induced mice to obtain significant pain symptoms and astrocyte activation in lumbar enlargement. Selective resident astrocyte elimination in lumbar enlargement could attenuate neuropathic pain and activate microglia. Interestingly, the type I interferons (IFNs) signal was significantly activated after astrocytes elimination, and the most activated Gene Ontology terms and pathways were associated with the type I IFNs signal which was mainly activated in microglia and further verified in vitro and in vivo. Furthermore, different concentrations of interferon and Stimulator of interferon genes (STING) agonist could activate the type I IFNs signal in microglia. These results elucidate that selectively eliminating resident astrocytes attenuated neuropathic pain associated with type I IFNs signal activation in microglia. Targeting type I IFNs signals is proven to be an effective strategy for neuropathic pain treatment after SCI.