Distinct cellular and molecular mechanisms for β3 adrenergic receptor-induced beige adipocyte formation
Abstract
Beige/brite adipocytes are induced within white adipose tissues (WAT) and, when activated, consume glucose and fatty acids to produce heat. Classically, two stimuli have been used to trigger a beiging response: cold temperatures and β3-adrenergic receptor (Adrb3) agonists. These two beiging triggers have been used interchangeably but whether these two stimuli may induce beiging differently at cellular and molecular levels remains unclear. Here, we found that cold-induced beige adipocyte formation requires Adrb1, not Adrb3, activation. Adrb1 activation stimulates WAT resident perivascular (Acta2+) cells to form cold-induced beige adipocytes. In contrast, Adrb3 activation stimulates mature white adipocytes to convert into beige adipocytes. Necessity tests, using mature adipocyte-specific Prdm16 deletion strategies, demonstrated that adipocytes are required and are predominant source to generate Adrb3-induced, but not cold-induced, beige adipocytes. Collectively, we identify that cold temperatures and Adrb3 agonists activate distinct cellular populations that express different β-adrenergic receptors to induce beige adipogenesis.
https://doi.org/10.7554/eLife.30329.001eLife digest
Excess accumulation of a type of fat called white fat is associated with obesity and metabolic problems. White fat cells store energy. White fat tissue also contains some beige fat cells, which burn fats and sugars to produce heat. Cold temperatures trigger the production and activity of beige fat cells, which allows the body to stay warm. People with obesity tend to have less beige fat and more white fat. This has led scientists to test whether treatments that increase the number of beige fat cells a person has could reduce fat mass and improve metabolism.
To develop treatments that increase beige fat, scientists must first understand where it comes from and how cold and other factors stimulate its growth. Recent studies have shown that smooth muscle cells, which surround blood vessel walls, make cold-induced beige fat cells. A widely used drug that turns on the β3 adrenergic receptor, which is found in the cell membrane, also boosts the creation of beige fat cells. Yet, it was not clear exactly how cold or this drug triggers the production of beige fat.
Now, Jiang et al. show that drugs that target β3 adrenergic receptors cause white fat cells in mice to change into beige fat cells. The experiments also showed that cold turns on a different receptor called the β1 adrenergic receptor on smooth muscle cells causing them to make beige fat cells. This shows that there is more than one source for beige fat cells in the body and that different strategies for increasing beige fat cell numbers do not work the same way.
More studies are needed to learn whether beige fat cells produced after exposure to cold or drugs behave in the same way and have similar affects on metabolism. This could help scientists determine if one of these strategies could make a better treatment for obesity or other metabolic disorders.
https://doi.org/10.7554/eLife.30329.002Introduction
The ability to defend core body temperature in response to cold environments is, in part, mediated by non-shivering thermogenesis, through the recruitment and activation of brown and beige/brite (brown-in-white) adipose tissues (Cannon and Nedergaard, 2004). Beige adipocytes are activated in white adipose tissue (WAT) by cold exposure or by beta3-adrenergic receptor (Adrb3) agonists via the sympathetic nervous system (Young et al., 1984). Activation of beige adipocyte thermogenesis requires the induction of numerous thermogenic and mitochondrial genes such as uncoupling protein 1 (Ucp1) (Cannon and Nedergaard, 2004). To perform thermogenic function, beige adipocytes, like classical brown adipocytes, take up substantial amounts of glucose and free fatty acids and convert these molecules into heat rather than ATP. This uncoupling ability is clinically desirable because it has the potential to lower body fat percentage, reduce blood sugars and increase metabolic rate (Sidossis and Kajimura, 2015; Blondin et al., 2014; Blondin et al., 2017). Using radiolabeled glucose uptake and positron electron tomography (PET) imaging, brown/beige fat has been identified in cold-exposed adult humans (Cypess et al., 2009; van Marken Lichtenbelt et al., 2009). These imaging studies have indicated that glucose uptake occurs often in the supraclavicular region. Furthermore, supraclavicular WAT biopsies from cold-exposed humans have shown the presence and activation of brown/beige fat, which appears to resemble rodent beige fat rather than rodent brown fat (Shinoda et al., 2015; Wu et al., 2012). In addition to cold exposure, Adrb3 agonists also stimulate and promote beige adipocyte formation and activation (Himms-Hagen et al., 1994). However, clinically used non-selective Adrb3 agonists have led to off target side-effects such as tachycardia and hypertension, which precludes the use of these Adrb3 agonists as beiging agents in the clinic (Arch, 2011). Yet, recent efforts by Cypess and colleagues have identified a more selective Adrb3 agonist, mirabergon (MB), which is clinically used to treat overactive bladder conditions, as a potential human ‘beiger’ (Cypess et al., 2015).
Numerous studies have proposed various origins of beige adipocytes. For example, the notion that white adipocytes can transdifferentiate into beige adipocytes has been a conventional notion (Barbatelli et al., 2010; Smorlesi et al., 2012). On the other hand, several recent perivascular genetic fate-mapping studies have indicated that cold-induced beige adipocytes are generated from blood vessel-derived progenitors within WAT. For instance, perivascular smooth muscle cells that express Myh11, Pdgfrb and Acta2 have been shown to be a progenitor cell pool for cold-induced beige adipocytes (Berry et al., 2016; Long et al., 2014; Shao et al., 2016). Also, platelet-derived growth factor receptor α (Pdgfra) positive fibroblasts can form some Adrb3-induced beige adipocytes within the perigonadal adipose tissue (PGW) but not within the subcutaneous inguinal adipose (IGW) WAT depot (Lee et al., 2012). Yet, Pdgfra+ cells do not appear to serve as a cold-induced beige progenitor pool (Lee et al., 2015; Berry et al., 2016). Thus, it is unclear if smooth muscle cells are the major contributing cellular source for Adrb3-induced beige adipocytes.
Mechanistically, studies have also suggested that both cold and Adrb3 stimulate brown adipocyte activation and beige adipocyte recruitment similarly through the activation of Adrb (Ramseyer and Granneman, 2016). Norepinephrine signaling, via Adrb3, not only stimulates thermogenic action in mature brown adipocytes but also recruits and activates beige adipocytes within WAT (Grujic et al., 1997). Further, norepinephrine signaling through Adrb3-cAMP activation upregulates thermogenic genes such as Ucp1 potentiating the beiging process (Cao et al., 2001). However, cellular expression studies have indicated that Adrb3 expression is restricted to mature white and brown adipocytes and is not expressed within the stromal vascular fraction (SVF), the proposed site of both white and beige progenitors (Collins et al., 1994; Berry et al., 2016). In contrast to Adrb3, Adrb1 is expressed in the SVF, not in mature adipocytes, and is thought to mediate the proliferation and differentiation of classical brown adipocyte progenitors (Bronnikov et al., 1992). Thermogenically, Adrb1 null mice are unable to defend their body temperature in response to the cold (Ueta et al., 2012); whereas, transgenic overexpression of Adrb1 showed robust cold-induced beiging potential within WAT (Soloveva et al., 1997). Overall, several studies suggest mechanistic differences between cold- and Adrb3-induced beige adipocyte formation (Vosselman et al., 2012). But whether these two receptors mediate or recruit beige adipocytes similarly remains unknown.
Here, we show that smooth muscle/mural cells (Acta2 and Myh11) do not fate-map into Adrb3-induced beige adipocytes rather most Adrb3-induced beige adipocytes emanate from pre-existing white adipocytes. White adipocyte beiging necessity tests demonstrate the requirement of white adipocytes to form a significant percentage of beige adipocytes. Moreover, we find that that cold-induced beiging requires Adrb1 activation but not Adrb3 signaling. These data suggest that several cellular sources exist for beige adipocyte formation, which could provide mechanistic insight and clinical utility into enhancing beige adipocyte formation, function and perdurance.
Results
Difference between cold- and Adrb3-induced beiging
To begin to explore possible differences between cold-induced and Adrb3-induced beiging, we evaluated the relative appearance of in vivo beige adipogenesis. C57BL/6J-inbred mice were randomized to room temperature (23°C), cold (6.5°C) or CL316,243 (CL, 1 mg/Kg), a Adrb3 selective agonist, for 1, 3, or 7 days. After 1 day of cold exposure, beige adipocyte appearance was minimal within the subcutaneous inguinal adipose depot (IGW), the predominant beiging WAT. Conversely, 1 day of CL treatment produced some Ucp1+ multilocular beige adipocytes and after 3 days of CL many Ucp1+ beige adipocytes could be observed. In contrast, after 3 days of cold exposure, a small number of Ucp1+ beige adipocytes were present. By day 7, both beiging agents produced many Ucp1+ beige adipocytes throughout the IGW depot (Figure 1A and Figure 1—figure supplement 1). These findings were also confirmed by quantitative real time-PCR analysis of beige and thermogenic gene expression (Figure 1—figure supplement 1).
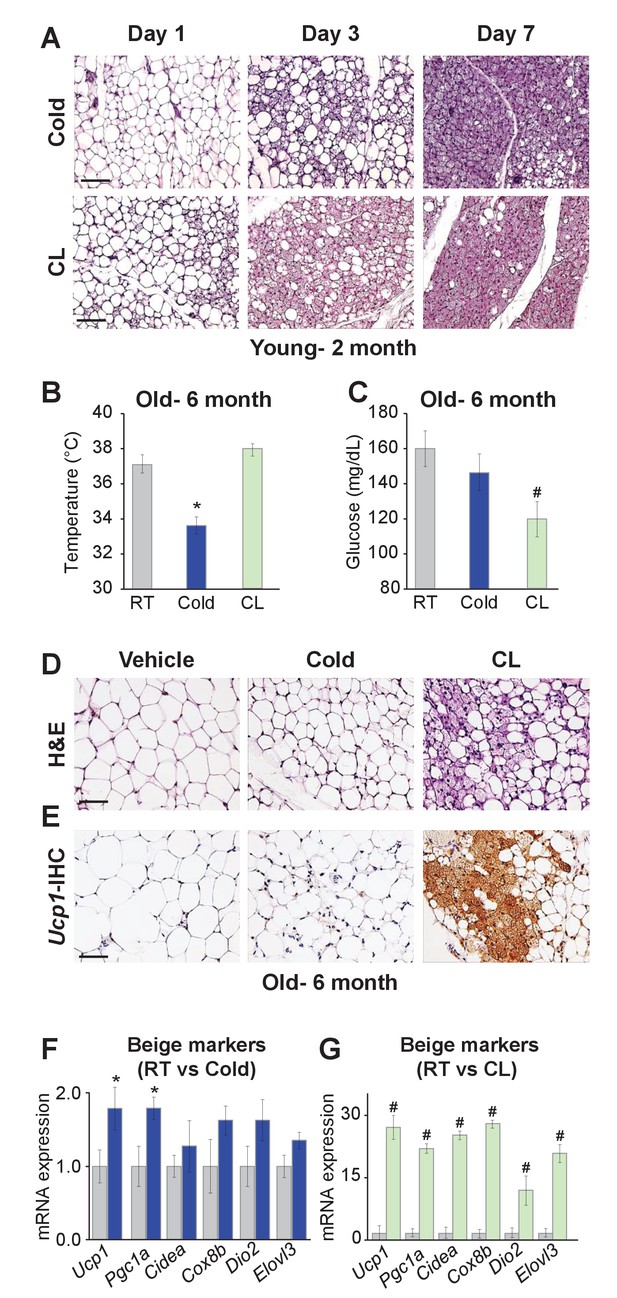
Differences between cold exposure and Adrb3 agonists-induced beiging.
(A) Representative H&E-stained images of sections from IGW depots from two-month-old male mice were cold exposed (6.5°C) or treated with CL (1 mg/Kg/mouse/day) for one, three or seven days (n= 6/group). (B) Rectal temperatures from six-month-old male mice maintained at room temperature, cold exposed or treated with CL for seven days (n= 10/group). (C) Sera glucose from mice described in (B). (D) Representative H&E-stained images of IGW depot sections from mice described in (B). (E) Representative Ucp1 immunohistochemistry (IHC)-stained images of IGW depot sections from mice described in (B). (F) mRNA expression of beige and thermogenic genes from mice described in (B) maintained at room temperature or cold exposed for seven days. (G) mRNA expression of beige and thermogenic genes from mice described in (B) maintained at room temperature or treated with CL for seven days. *p<0.05 unpaired t-test, two tailed: Cold exposed compared to room temperature mice. #p<0.05 unpaired t-test, two tailed: CL-treated compared to vehicle room temperature-treated mice. Scale bar = 100 μm.
To continue to test differences between cold- and Adrb3-induced beiging, we aged (six months) C57BL/6J inbred male mice. Aged mice have been shown to be defective in cold-induced beige adipocyte formation attributed to a cellular aging senescence-like phenotype of beige progenitors (Rogers et al., 2012; Berry et al., 2017). Mice were then randomized to room temperature, cold or CL for seven days. Cold exposure was unable to induce beiging or a thermogenic program, as previously reported (Figure 1B–F) (Berry et al., 2017). Strikingly, CL induced robust beiging, glucose consumption, and thermogenic gene expression compared to room temperature controls (Figure 1B–G).
Smooth muscle cells do not generate Adrb3- induced beige adipocytes
To continue to explore potential difference between cold-induced and Adrb3-induced beige adipocytes, we attempted to probe whether perivascular mural cells that express Acta2, which function as a major cellular source for cold-induced beige adipocytes, also served as a progenitor source for Adrb3 activation. Recent studies have used the Acta2Cre-ERT2; Rosa26RRFP as beige fate-mapping model (Figure 2A) (Berry et al., 2016; Wendling et al., 2009). Notably, neither the Acta2-driven reporter nor endogenous Acta2 is expressed in beige or brown adipocytes or white adipocytes (Berry et al., 2016). To induce recombination, one dose of tamoxifen (TM, 50 mg/Kg) for two consecutive days was administered to two-month-old Acta2Cre-ERT2; Rosa26RRFP male mice (Figure 2A). After a two-week TM washout period, mice were randomized to vehicle, CL, or mirabegron (MB, 1 mg/Kg), a clinically used Adrb3 agonist, for seven days (Figure 2A). Histological assessment revealed both CL and MB induced beige adipocyte formation, similarly, in the IGW and PGW WAT depots (Figure 2—figure supplement 1). Nevertheless, beige adipocytes induced by CL- or MB- did not originate from an Acta2-RFP perivascular source (Figure 2B and Figure 2—figure supplement 2). Acta2-RFP expression was restricted to the vasculature under both Adrb3 agonist treatments, similar to vehicle sections (Figure 2B and Figure 2—figure supplement 2). We next tested if chronic cold or CL treatment could provoke Acta2+ mural cells to form beige adipocytes. We cold exposed or CL treated TM-induced Acta2-RFP mice for 14 days. Fate mapping studies demonstrated that Acta2+ cells formed beige adipocytes after 14 days of cold exposure, comparable to seven days of cold treatment (Figure 2—figure supplement 3) (Berry et al., 2016), However, CL-induced Ucp1+ beige adipocytes were RFP negative after 14 days of treatment (Figure 2—figure supplement 3). We then examined if age impacts Acta2-RFP fate mapping into Adrb3-induced beige adipocytes. We administered TM to P30, P90 and P180 Acta2Cre-ERT2; RFP mice and then randomized mice to vehicle or CL for seven days, 2 weeks post TM. Histological assessment demonstrated that Adrb3-induced Ucp1+ beige adipocytes were Acta2-RFP negative (Figure 2—figure supplement 4). Taken together, these data suggest that Acta2+ mural cells do not serve as a progenitor cell source for Adrb3-induced beige adipocytes.
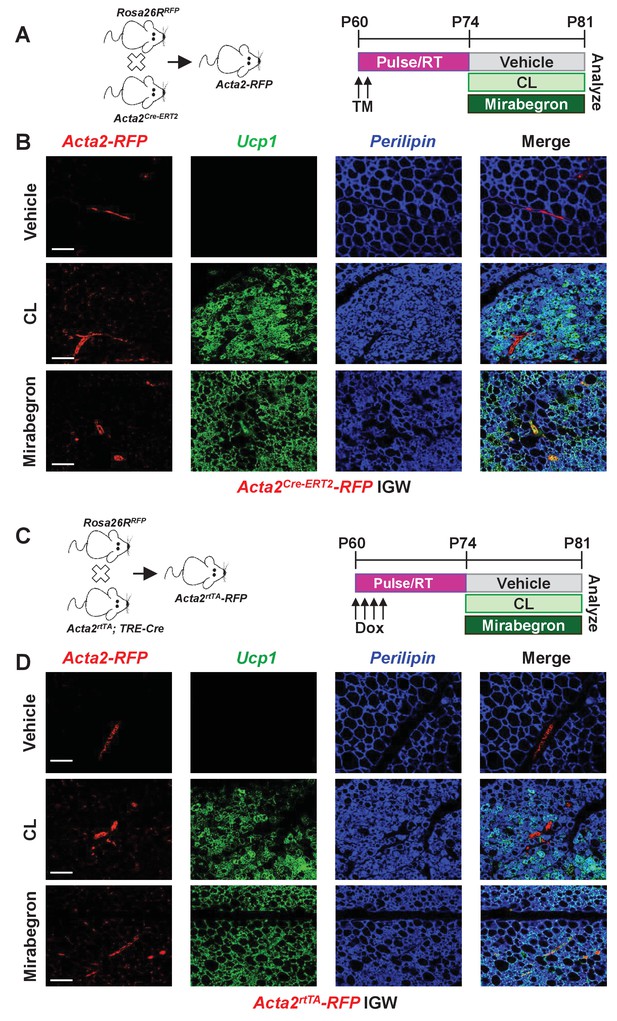
Smooth muscle cells are not a source of Adrb3-induced beiging.
(A) Illustration of mice and experimental regime: two-month-old Acta2Cre-ERT2; RosaR26RFP male mice were administered one dose of TM for two consecutive days. Two weeks post TM, mice were randomized to vehicle, CL or mirabegron for seven days. (B) Representative images of fate mapping analysis of Acta2Cre-ERT2-RFP+ cells into Ucp1 beige adipocytes within IGW depots from mice described in (A). (C) Illustration of mice and experimental regime: two-month-old Acta2rtTA; TRE-Cre; RosaR26RFP male mice were administered doxycycline (Dox) for four consecutive days. Two weeks post Dox, mice were randomized to vehicle, CL or mirabegron for seven days. (D) Representative images of fate mapping analysis of Acta2rtTA-RFP+ cells into Ucp1 beige adipocytes within IGW depots from mice described in (C). Scale bar = 200 µm.
This negative tracing by Acta2-RFP into Adrb3-induced beige adipocytes may be, in part, due to undesired biological actions of TM on white adipose tissues thereby masking or altering fate mapping capabilities (Ye et al., 2015). To overcome possible TM-tracing issues, we employed a doxycycline (Dox, 0.5 mg/L) inducible Acta2rtTA; TRE-Cre; Rosa26RRFP mouse model (Figure 2C) (Berry et al., 2016). Acta2rtTA-RFP was restricted to the vasculature and did not generate CL- or MB-induced beige adipocytes, confirming our TM-induced Acta2Cre-ERT2; Rosa26RRFP fate-mapping studies (Figure 2D and Figure 2—figure supplement 5).
To support our Adrb3 Acta2 beiging fate-mapping studies, we performed two necessity strategies: (1) a cell-killing strategy (diphtheria toxin fragment A; DTA) (Ivanova et al., 2005) and (2) a blockade of adipocyte differentiation (Pparg deletion) (Figure 2—figure supplement 6) (Berry et al., 2016; Jiang et al., 2014). A potential concern of these methods is that these deletions will occur in other Acta2+ compartments which could alter beiging potential (Wendling et al., 2009). To minimize off-target affects, we conducted these studies in a temporally regulated fashion and proximate to Adrb3 agonist administration (Figure 2—figure supplement 6). Both strategies (DTA, Ppargfl/fl) did not appear to alter Adrb3-induced beige adipocyte formation or the physiological responses to CL treatment (Figure 2—figure supplement 6), indicating that Acta2+ cells are not a primary source of Adrb3-induced beige adipocytes.
Other perivascular sources such as Myh11+ cells have been shown to fate map into cold-induced beige adipocytes (Long et al., 2014). So, we employed the Myh11Cre-ERT2 combined with RosaR26RRFP to generate Myh11Cre-ERT2; R26RRFP fate mapping mice. We randomized TM-induced mice to vehicle or CL for seven days and found that CL-induced Ucp1+ beige adipocytes that were RFP negative (Figure 2—figure supplement 7). To further test if Myh11+ cells could commit to the Adrb3-induced beige progenitor lineage, we administered TM to two-month-old Myh11Cre-ERT2; R26RRFP mice and administered vehicle or CL one month post TM for seven days. We found that no new Adrb3-induced beige adipocytes emanated from an Myh11+ smooth muscle cell under this longer time interval (Figure 2—figure supplement 8). Collectively, our fate mapping and necessity tests demonstrate that smooth muscle/mural cells, which are required for cold-induced beiging, are not a cellular source of Adrb3-induced beige adipocytes.
Pdgfra+ cells are a minor subset of progenitors for Adrb3-induced beige adipocytes
Adrb3 agonists can stimulate perivascular Pdgfra+ fibroblasts within visceral PGW, but not IGW depots, to form beige adipocytes (Lee et al., 2012). Yet, PdgfraCre-ERT2; RFP cells do not appear to generate cold-induced beige adipocytes (Berry et al., 2016; Lee et al., 2015). As a potential difference between cold- and Adrb3-induced beiging, we re-visited if Pdgfra+ cells are a source of Adrb3-induced beige adipocytes by employing the PdgfraCre-ERT2; RFP model (Figure 3—figure supplement 1). TM induced PdgfraCre-ERT2; Rosa26RRFP two-month-old male mice were randomized to vehicle, CL or MB (Figure 3—figure supplement 1). Fate-mapping revealed that roughly 2% of subcutaneous IGW multilocular Ucp1+ beige adipocytes were RFP positive (Figure 3A). Conversely, ~10–15% of perigonadal multilocular Ucp1+ beige adipocytes were RFP positive (Figure 3—figure supplement 1). However, many Ucp1 antibody positive beige adipocytes were RFP negative, suggesting that Pdgfra+ cells are not a major source used to generate Adrb3-induced beige adipocytes. One potential caveat of inducible systems is recombination efficiency (Feil et al., 1997). To examine recombination efficiency, we TM pulsed two-month-old PdgfraCre-ERT2; Rosa26RRFP mice. SV cells were isolated from subcutaneous adipose depots (inguinal and periscapular) and subjected to flow cytometric analysis, 24 hr after the last TM injection. We found that Pdgfra-RFP+ cells were 100% positive for Pdgfra antibody staining (Figure 3B). Conversely, ~70% of Pdgfra antibody+ cells were RFP+ indicating a high correspondence between reporter and endogenous Pdgfra expression (Figure 3C,D). We also performed quantitative real-time PCR analysis of isolated Pdgfra-RFP+ cells and found that these cells did not express a smooth muscle signature (Figure 3E). Immunohistochemistry also demonstrated that Pdgfra-RFP+ cells did not overlap with Acta2+ cells, as previously reported (Figure 3—figure supplement 1) (Lee et al., 2012).
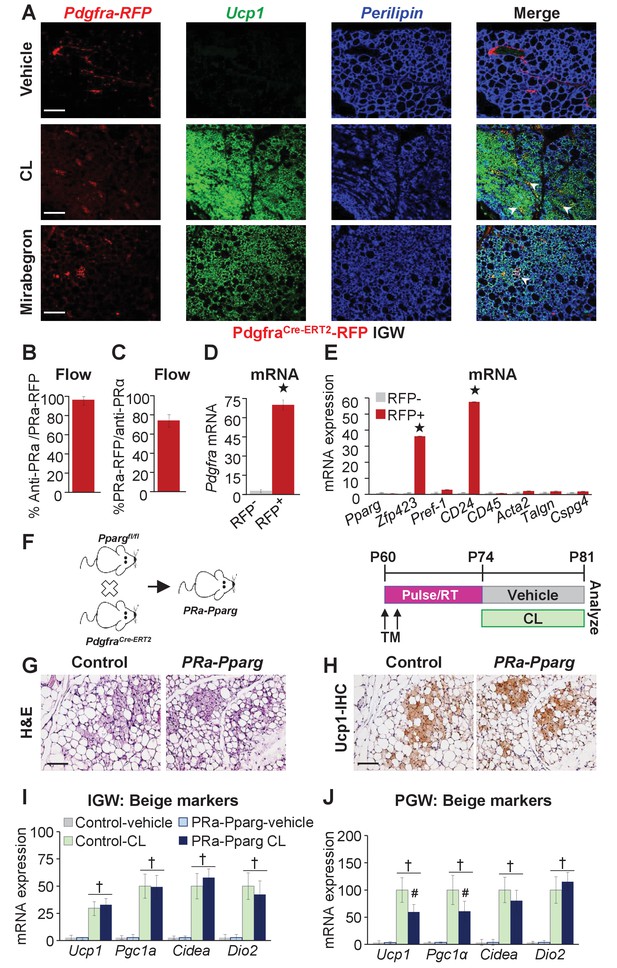
Pdgfra+ cells are not required for Adrb3-induced beiging.
(A) Representative images of Pdgfra-RFP fate mapping immunostaining of IGW depots from two-month-old male PdgfrαCre-ERT2; RosaR26RFP mice. Mice were administered one dose of tamoxifen (TM) for two consecutive days and after a two-week TM washout period mouse were treated with vehicle, CL or mirabegron for seven days. White arrowheads indicate RFP+ and Ucp1 +beige adipocytes. (B) TM-pulsed Pdgfra-RFP+ cells were FACS isolated from the WAT SV compartment and antibody stained for Pdgfra and analyzed by flow cytometry (n= 6/group). (C) SV cells were isolated from TM-pulsed Pdgfra-RFP+ mice. Cells were immunostained for Pdgfra and examined for Pdgfra-RFP positivity by flow cytometry (n= 6/group). (D) Cells described in (B) were FACS isolated and examined for mRNA expression of Pdgfra. ★p<0.001 RFP+ compared to RFP- cells (n= 6/group). (E) Cells described in (B) were FACS isolated and mRNA expression of denoted genes were examined. ★p<0.001 RFP+ compared to RFP- cells (n= 6/group). (F) Illustration (left) and experimental procedure (right) used to generate TM-induced two-month-old PdgfraCre-ERT2; Ppargfl/fl (PRa-Pparg) male mice (n= 8/group). (G) Representative H&E-staining images of IGW depot sections from mice described in (F). (H) Representative Ucp1 IHC staining of IGW depot sections from mice described in (F). (I) mRNA expression of beige and thermogenic markers from IGW depots from mice described in (F) (n= 6/group in triplicate). (J) mRNA expression of beige and thermogenic markers from PGW depots from mice described in (F). †p<0.05 unpaired t-test, two tailed: PRa-Pparg CL treated compared to control CL treated. Scale bar = 200 µm.
To support our Pdgfra fate-mapping studies, we incorporated a Ppargfl/fl allele with the PdgfraCre-ERT2 mouse model (denoted PRa-Pparg) to block adipogenic action in Pdgfra+ cells. Two-month-old PRa-Pparg male mice were TM-induced two weeks prior to vehicle or CL treatment for seven days (Figure 3F). We found that Adrb3-induced beige adipocyte formation was unaltered in response to blocking adipogenesis in Pdgfra+ cells (Figure 3G–I and Figure 3—figure supplement 2). However, in PGW depots, some beige genes were dampened (Figure 3J). Together, it appears, under our conditions, that Pdgfra+ cells are a minor WAT depot-specific subset of progenitors for Adrb3-induced beiging.
Pparg-marked perivascular progenitors do not generate Adrb3-induced beige adipocytes
To globally examine if Adrb3-induced beige adipocytes were generated from a common white and beige adipose progenitor cell source, we employed the AdipoTrak system (PpargtTA; TRE-H2BGFP), which marks the entire adipose lineage (Jiang et al., 2014; Tang et al., 2008). We Dox suppressed the AdipoTrak system from conception until postnatal day 60 (P60) (Figure 3—figure supplement 3). Under these conditions, PpargtTA activity is suppressed and prevents nucleosome incorporation of H2BGFP. Dox removal, allows PpargtTA to become active and H2BGFP is incorporated into proliferating nucleosomes of the progenitor compartment but not into post-mitotic cells such as existing white and beige adipocytes. GFP will be detected in the nuclei of adipocytes if they differentiate from a proliferating GFP-marked progenitor source. Reactivation of the AdipoTrak system after Dox suppression occurs predominantly around the vasculature, and these GFP-labeled progenitors can then be traced into a fraction of cold-induced beige progenitors (Berry et al., 2016). However, after CL administration, histological staining revealed that <2% of beige adipocytes were GFP+ (Figure 3—figure supplement 3). These data suggest that the proliferating perivascular Pparg+ adipose progenitors do not generate Adrb3-induced beige adipocytes.
Pre-existing white adipocytes generate many new Adrb3-induced beige adipocytes
Transdifferentiation or interconversion of white adipocytes to beige adipocytes has long been considered the standard notion for the beiging phenomena (Barbatelli et al., 2010); however, recent studies have challenged this view (Berry et al., 2016; Wang et al., 2013; Vishvanath et al., 2016). Yet, our data thus far have not identified a progenitor cell source for Adrb3-induced beiging. Therefore, we further probed if existing white adipocytes could generate Adrb3-induced beige adipocytes by generating AdiponectinCre-ERT2; Rosa26RRFP (Adpn-RFP) mice. Adpn-RFP marks 100% of pre-existing white and beige adipocytes but does not mark the adipose SV compartment or newly generating adipocytes (Figure 4A and Figure 4—figure supplement 1) (Berry et al., 2016; Sassmann et al., 2010). TM-induced Adpn-RFP mice were randomized to vehicle, CL, or MB treatment for seven days, two-weeks post TM administration (Figure 4B). Beige adipocytes generated by CL or MB were formed from an Adpn-RFP+ source (Figure 4C,D). That is ~75% of all Adrb3-induced Ucp1+ beige adipocytes were RFP+, suggesting that white adipocytes could interconvert to beige adipocytes (Figure 4E). These data were confirmed by longer pulse-chase experiments in which we TM induced two-month-old AdiponectinCre-ERT2; Rosa26RRFP mice and then treated them with CL two months later. Fate mapping assessment demonstrated that ~70% of Ucp1+ beige adipocytes were RFP+, suggesting that pre-existing white adipocytes are a major source of Adrb3-induced beige adipocytes (Figure 4—figure supplement 1).
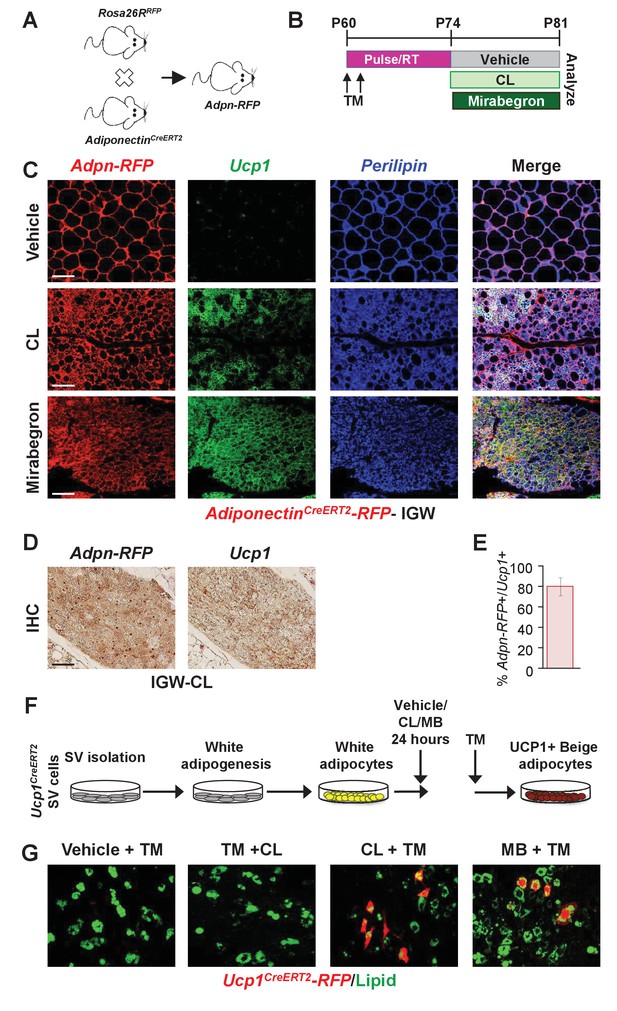
Pre-existing white adipocytes are the major source of Adrb3-induced beiging.
(A) Genetic schema of mice used to generate two-month-old AdiponectinCre-ERT2; RFP (Adpn-RFP) male mice (n = 6/group). (B) Experimental paradigm of TM induction and subsequent treatment with vehicle, CL or mirabegron of Adpn-RFP mice. (C) Representative images of Adpn-RFP fate mapping and Ucp1 immunostaining of IGW depot sections from mice described in (B). Scale bar = 200 µm. (D) Representative images of RFP and Ucp1 IHC of IGW depot sections from mice described in (B). Scale bar = 100 µm. (E) Quantitation of co-localized Adpn-RFP+/Ucp1+ beige adipocytes in response to CL (n= 1000 beige adipocytes). (F) Experimental procedure to stimulate beiging in vitro. (G) Representative images of Ucp1-RFP fluorescence co-localization with lipid staining (LipidToxTM) after CL treatment of cultured white adipocytes.
To continue to test the interconversion of white adipocytes to beige adipocytes in response to Adrb3 agonists, we turned to an in vitro system in which we could test the interconversion of white adipocytes to beige adipocytes. We isolated SV cells from un-induced Ucp1Cre-ERT2; RFP mice (marks only mature beige adipocytes) and were induced with white adipogenic media for seven days. Cell culture-induced white adipocytes were then treated with vehicle, CL, or MB for 4 hr and thermogenic genes were examined (Figure 4—figure supplement 1). Vehicle-treated white adipocytes did not express Ucp1 mRNA nor other thermogenic genes. However, CL- or MB-treated white adipocytes had elevated expression of Ucp1 and Pgc1a, as previously observed (Figure 4—figure supplement 1) (Cao et al., 2001). Next, we tested if Ucp1-RFP (Ucp1Cre-ERT2; RFP) expression could be turned on in cultured white adipocytes as a marker of beige adipocyte interconversion. Cultured white adipocytes were treated with three different conditions to monitor Ucp1-RFP expression: vehicle and TM, TM and CL, and MB and TM (Figure 4F). Under vehicle conditions Ucp1-RFP was not expressed. However, treating the cells with CL or MB, and then administering TM, induced the expression of the Ucp1-RFP reporter (Figure 4G). As a control, we administered TM first then added CL. Under this condition, we did not observe Ucp1-RFP reporter expression (Figure 4G). Of note, we treated SV cells with CL or MB, and found that Ucp1 and other thermogenic genes remained undetectable (not shown). Taken together, these data indicate that both pre-existing white adipocytes and cell culture-induced white adipocytes can induce a beige adipocyte morphology and thermogenic program.
Pre-existing white adipocytes are required for Adrb3-induced beige adipocytes
To test if white adipocytes are required to form Adrb3-induced beige adipocytes, we incorporated a Prdm16fl/fl allele with AdiponectinCre-ERT2; Rosa26RRFP mice (Figure 5A). We used AdiponectinCre-ERT2 to specifically target pre-existing mature white and beige adipocytes to avoid potentially affecting newly evolving progenitors undergoing adipogenesis. Additionally, we are not testing the indisputable role of Prdm16 in beige adipocyte formation but are testing the requirement of white adipocytes to generate beige adipocytes under Adrb3 agonists (Cohen et al., 2014). In these studies, if white adipocytes are not required then new Adrb3-induced beige adipocytes should be observed. We TM induced two-month-old AdiponectinCre-ERT2; Rosa26RRFP; Prdm16fl/fl (Adpn-Prdm16) male mice; two weeks later mice were randomized to vehicle, CL, or cold exposed for seven days (Figure 5B). We found that cold-exposed Adpn-Prdm16 mice showed normal beiging (Figure 5—figure supplement 1). In contrast, CL-induced beiging was diminished, but not completely absent, in Adpn-Prdm16 mice (Figure 5C–G and Figure 5—figure supplement 1). As a next step, we isolated SV cells from un-induced Adpn-Prdm16 mice. Cells were cultured in white adipogenic media and then administered TM at the end of differentiation to delete Prdm16 in mature white adipocytes (Figure 5H). Adipocytes were treated with vehicle or CL for 24 hr then examined for triglyceride levels and thermogenic gene expression. We found that adipocytes deficient in Prdm16 were unable to lower triglyceride levels compared to control CL-treated adipocytes (Figure 5I). Further, mRNA expression induction of beige and thermogenic genes was dampened in response to Prdm16 deletion (Figure 5J).
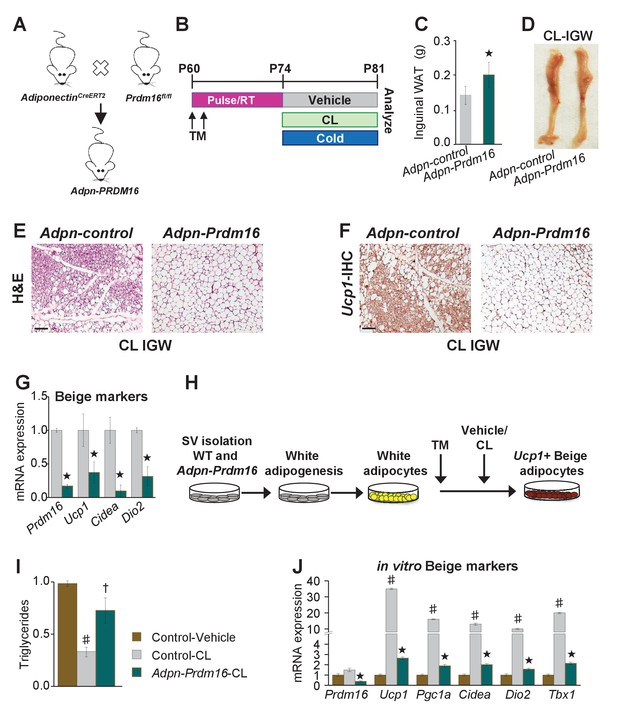
Pre-existing white adipocytes are required for Adrb3-induced beiging.
(A) Illustration of genetic alleles used to generate two-month-old AdiponectinCre-ERT2; RosaR26RFP; Prdm16fl/fl (Adiponectin-Prdm16) male mice (n = 10/group). (B) Experimental procedure to TM induced and treated Adiponectin-Prdm16 mice. (C) IGW depot weight from mice described in (B). ★p<0.001 unpaired t-test, two tailed: Mutant CL-treated compared to control CL-treated mice. (D) Representative photographs of intact IGW depots from mice described in (B). (E) Representative H&E staining of IGW depot sections from mice described in (B). (F) Representative Ucp1 IHC staining of IGW depot sections from mice described in (B). (G) mRNA expression of beige markers from mice described in (B). ★p<0.001 unpaired t-test, two tailed: Mutant CL- treated compared to control CL-treated mice. (H) Experimental procedure for in vitro beiging. (I) Triglyceride levels in denoted cells after 24 hr vehicle or CL treatment (n = 4/group in triplicate). ♯p<0.001 unpaired t-test, two tailed: CL- treated compared to vehicle-treated cells. †p<0.05 unpaired t-test, two tailed: mutant CL-treated compared to vehicle-treated control cells. (J) mRNA expression of beige and thermogenic genes from cells described in (H) (n = 6/group in triplicate). ♯p<0.001 unpaired t-test, two tailed: CL-treated compared to vehicle-treated cells. ★p<0.001 unpaired t-test, two tailed: Mutant CL-treated compared to control CL-treated cells. All data are means ±SEM. Scale bar = 200 µm.
To substantiate our white adipocyte necessity results and to test the requirement of Prdm16 in cold-induced beiging process, we incorporated the Prdm16fl/fl allele with the Acta2Cre-ERT2 (Acta2-Prdm16). We TM induced two-month-old Acta2-control and Acta2-Prdm16 mice; two weeks later mice were cold exposed for one week (Figure 5—figure supplement 2). We found that control mice exhibited beiging whereas Acta2-Prdm16 mice had reduced beiging potential as assessed by rectal temperature, sera glucose, H&E staining, Ucp1 IHC, Acta2 fate mapping and beige and thermogenic gene expression (Figure 5—figure supplement 2). Collectively, these data suggest that white unilocular adipocytes are a major source and are required for beige adipocyte formation in response to Adrb3 activation.
Adrb3 does not mediate cold-induced beiging
The above data suggested that Adrb3 agonists convert white adipocytes to beige adipocytes whereas cold provokes perivascular smooth muscle cells to form beige adipocytes. Previous reports have indicated that Adrb signaling mediates beige and brown adipocyte recruitment and thermogenic action in response to cold temperatures and Adrb3 agonists (Cannon and Nedergaard, 2004; Lidell et al., 2014). Since cold and Adrb3 agonists trigger different cell types to form beige adipocytes, we tested if inhibiting Adrb3 activation altered cold-induced beiging. To test this, C57BL/6J-inbred mice were administered vehicle or SR59230A (SR59 1 mg/Kg/day), a Adrb3 antagonist, for 5 days prior to room temperature, cold exposure or CL administration (Figure 6A). SR59 had little to no effect on cold-induced beige adipocyte formation (Figure 6B–F). In contrast, blocking Adrb3 by SR59 reduced the ability of CL to promote beige adipocyte formation (Figure 6B–G). Of note, SR59 did not appear to alter brown adipose tissue, morphologically or genetically, in response to either CL or cold exposure (Figure 6—figure supplement 1), as observed in Adrb3 null genetic models (de Jong et al., 2017).
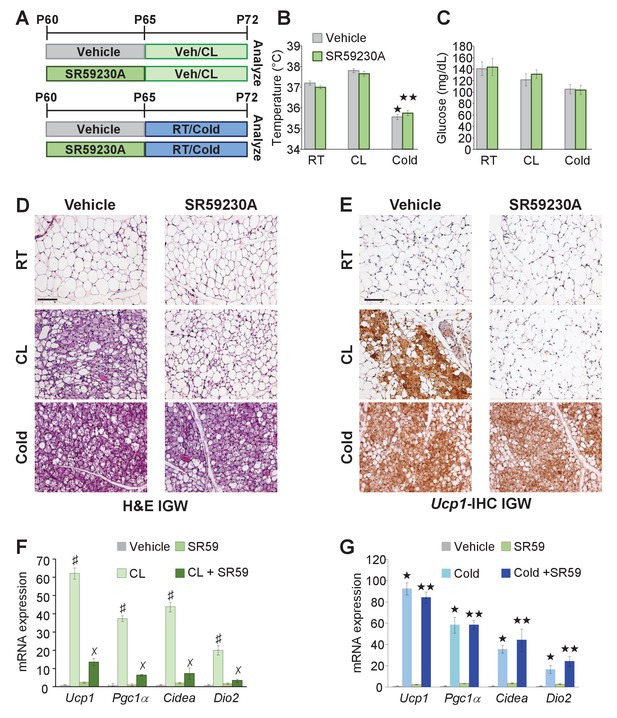
Adrb3 is not required for cold-induced beiging.
(A) Illustration of experimental paradigm: Two-month-old male mice were administered vehicle or SR59230A (SR59) for 5 days and then maintained at room temperature, cold exposed or treated with CL for seven days (n= 10/group). (B) Rectal temperature from mice described in (A). ★p<0.001 unpaired t-test, two tailed: cold exposed compared to room temperature vehicle-treated mice. ★★p<0.05 unpaired t-test, two tailed: cold + SR59 compared to vehicle room temperature-treated mice. (C) Sera glucose from mice described in (A). (D) Representative H&E staining of IGW depot sections from mice described in (A). (E) Representative Ucp1 IHC staining of IGW depot sections from mice described in (A). (F) mRNA expression of beige and thermogenic genes from mice described in (A) treated with vehicle and SR59 and then maintained at room temperature or cold exposed for seven days (n= 6/group in triplicate). ★p<0.001 unpaired t-test, two tailed: cold exposed compared to room temperature vehicle-treated mice. ★★p<0.05 unpaired t-test, two tailed: cold + SR59 compared to vehicle room temperature-treated mice. (G) mRNA expression of beige and thermogenic genes from mice described in (A) treated with vehicle and SR59 and then administered vehicle or CL for seven days (n= 6/group in triplicate). ♯p<0.01 unpaired t-test, two tailed: CL-treated compared to vehicle-treated mice. ✗p<0.01 unpaired t-test, two tailed: CL316 + SR59-treated mice compared to CL-treated mice. All data are means ±SEM. Scale bar = 200 µm.
Adrb1 mediates cold-induced beiging
In contrast to Adrb3 expression, which is restricted to mature white and brown adipocytes (Collins et al., 1994), other adrenergic receptors such as Adrb1 are expressed in the WAT SVF, the source of cold-induced beige progenitors (Bronnikov et al., 1992). We too examined if Adrb1-3 expression changes in response to white adipogenic media. We found that under white adipogenic conditions, Adrb1 and Adrb2 were slightly upregulated, and Adrb3 was significantly upregulated (Figure 7—figure supplement 1). As a next step, we performed quantitative real-time PCR analysis on FACS isolated Acta2-RFP+ cells to examine if Acta2+ cells expressed any Adrbs. We found that Acta2-RFP+ cells were enriched for Adrb1 but not for Adrb2, and Adrb3 was undetectable in both SV compartments (Figure 7—figure supplement 1). We also performed quantitative real-time PCR analysis of FACS isolated PRα-RFP+ fibroblast from WAT depots. We found that neither Adrb1 nor Adrb2 were enriched in PRα-RFP+ cells and Adrb3 was undetectable in both compartments (Figure 7—figure supplement 1). Since Acta2+ cells are enriched in Adrb1 this suggested that these cells might be engaged by Adrb1 activation to generate cold-induced beige adipocytes. To test this, we inhibited Adrb1 by treating mice with vehicle or talinolol (1 mg/Kg/day), a specific Adrb1 blocker, for 5 days prior to randomization to room temperature, cold or CL (Figure 7A). Under room temperature conditions, talinolol had no effect on temperature, adipose tissue mass, adipose tissue histology and beige adipocyte gene expression (Figure 7B–G and Figure 7—figure supplement 2). Similarly, talinolol appeared to have no effect on beiging when combined with CL-treatment; that is temperature, adipose tissue mass, histology and beige gene expression were similar between CL alone and CL with talinolol (Figure 7B–F and Figure 7—figure supplement 2). Conversely, talinolol-treated cold-exposed mice demonstrated an inability to defend body temperature, reduce blood glucose and maintained elevated levels of adipose tissue mass (Figure 7B,C and Figure 7—figure supplement 2). Further, talinolol-treated cold-exposed mice had reduced beiging potential as assessed by histology and beige and thermogenic gene expression (Figure 7D,E and G). Taken together, these data indicate that Adrb1 signaling is involved in cold-induced beige adipocyte formation but not Adrb3-induced beiging.
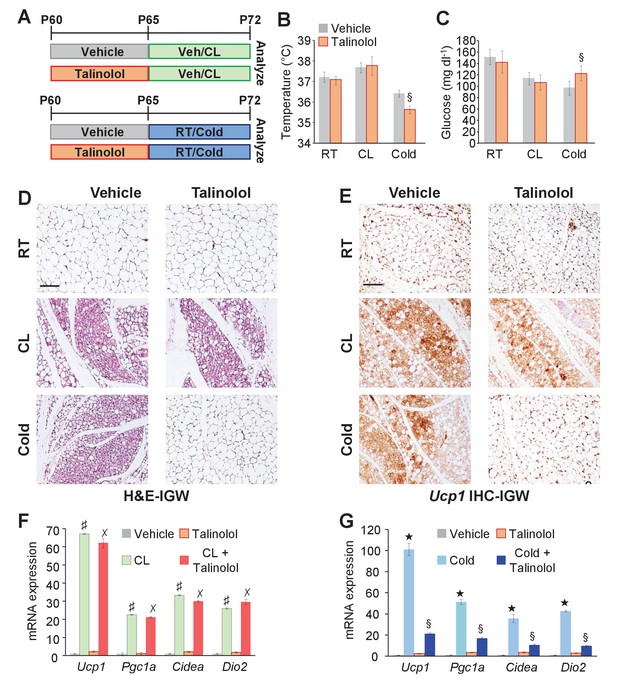
Adrb1 is required for cold-induced beiging.
(A) Experimental paradigm: Two-month-old male mice were first treated with vehicle or talinolol (1 mg/Kg/day) for five days. Mice were then maintained at room temperature, treated with CL or cold exposed for seven days (n= 10/group). (B) Rectal temperature from mice described in (A). §p<0.05 unpaired t-test, two tailed: cold + talinolol compared to cold + vehicle. (C) Sera glucose from mice described in (A). §p<0.05 unpaired t-test, two tailed: cold + talinolol compared to cold + vehicle. (D) Representative H&E staining of IGW depot sections from mice described in (A). (E) Representative Ucp1 IHC staining of IGW depot sections from mice described in (A). (F) mRNA expression of beige and thermogenic genes from mice described in (A) treated with vehicle and talinolol and then administered vehicle or CL for seven days. ♯ p<0.001 unpaired t-test, two tailed: CL-treated mice compared to vehicle-treated mice. ✗p<0.001 unpaired t-test, two tailed: CL + Talinolol compared to vehicle-treated mice. (G) mRNA expression of beige and thermogenic genes from mice described in (A) treated with vehicle and talinolol and then maintained at room temperature or cold exposed for seven days. ★p<0.001 unpaired t-test, two tailed: cold compared to room temperature vehicle-treated mice. §p<0.05 unpaired t-test, two tailed: cold + talinolol compared to cold + vehicle. All data are means ±SEM. Scale bar = 200 µm.
Discussion
Beige adipocytes have the potential to increase energy expenditure through the consumption of glucose and free fatty acids (Kajimura et al., 2015). These potential cellular energy sinks could help address the worldwide epidemic of obesity and its associated metabolic disorders (Yoneshiro et al., 2013). Several studies have indicated the presence and induction of metabolically relevant beige adipocytes in humans (Cypess et al., 2015; Saito, 2013; Saito et al., 2009; van der Lans et al., 2013; Wu et al., 2012; Yoneshiro et al., 2011b). For example, administration of mirabegron (MB) increased the resting metabolism of patients and induced the formation of beige adipocytes (Cypess et al., 2015). Other studies using cold exposure have seen a similar phenomenon including the induction of beige adipocytes (Cypess et al., 2009; Yoneshiro et al., 2011a). The full therapeutic potential of beige adipocytes has not been fully realized due, in part, to the lack of genetic tools to mark, track and manipulate the beige adipocyte progenitor cell source; however, the current study appears to overcome some of these barriers.
The cellular sources for cold- and Adrb3-induced beige adipocytes have attracted much attention but whether these two beiging stimuli trigger the same or different progenitor sources has remained ambiguous. The same set of tools, used herein, allowed us to identify that Acta2+ perivascular progenitor cells could serve as an indispensable source of cold-induced beige adipocytes (Berry et al., 2016). However, using two independent Acta2 genetic tools, we were unable to observe Acta2 beige adipocyte fate mapping in response to Adrb3 agonists. In addition, we were also unable to observe noticeable fate mapping of Myh11+ smooth muscle cells, which also serve as a cold-induced beige adipocyte source, into Adrb3-induced beige adipocytes. These data suggest that Adrb3 agonists do not engage smooth muscle-perivascular cells. These findings also correlated with our gene expression data that Acta2+ cells express Adrb1 not Adrb3. Moreover, our pharmacological studies indicate that cold-induced beige adipocyte formation relies primarily on Adrb1 activation rather than ARDB3 stimulation, which agrees with both our fate mapping and gene expression studies. These findings echo with a recent genetic study that showed that cold-induced beiging is intact in Adrb3 null mice (de Jong et al., 2017). However, other studies have demonstrated that Adrb3 null mice have less beiging potential (Barbatelli et al., 2010; Jimenez et al., 2003). The underlying differences between these studies may be, in part, due to genetic backgrounds of the null mice, further studies would be required.
Our studies support the notion that Adrb3-induced beige adipocytes originate from white adipocytes. Using AdiponectinCre-ERT2; RFP fate mapping studies, we found that the majority of Adrb3-induced beige adipocytes emanate from these pre-existing adipocytes. However, cold relies on Acta2+ mural cells. WAT necessity tests using AdiponectinCre-ERT2; Prdm16 demonstrated that pre-existing white adipocytes are required for Adrb3-induced beige adipocyte formation. A previous study demonstrated that deleting Prdm16 within the WAT lineage sufficiently blocked beige adipocyte formation from both cold exposure and Adrb3 agonists (Cohen et al., 2014). The major difference between the two studies is the use of the temporal AdiponectinCre-ERT2 genetic tool compared to the global AdiponectinCre genetic tool. In our studies using the AdiponectinCre-ERT2, Prdm16 is specifically deleted in all pre-existing adipocytes (beige and white) and not in newly generated adipocytes. In contrast, using AdiponectinCre model, Prdm16 is continuously deleted in all pre-existing and newly developing adipocytes (Jeffery et al., 2014; Shao et al., 2016; Wang et al., 2013), meaning that under all conditions, cold or Adrb3 agonist, beige adipogenesis is disrupted. Yet, fate mapping limitations aside, the AdiponectinCre tool identified the critical role of Prdm16 in beige adipogenesis.
Classical biochemical and pharmacokinetic studies have indicated that Adrb1 activation is not coupled to thermogenesis in brown adipocytes. In agreement, our data imply that Adrb1 is critical for Acta2+ perivascular cells to leave their vascular niche and form beige adipocytes. However, using pharmacological agents such as talinolol, could have systemic affects that may alter beiging potential independent of Adrb1 blockade within WAT. Pharmacokinetically, talinolol is highly hydrophilic with a half-life of 6–7 hr and has difficulty crossing the blood-brain barrier (Neil-Dwyer et al., 1981; Sourgens et al., 2003). Of note, mice were exposed to the cold or CL, 24 hr post talinolol treatment. Further investigation into the downstream signaling of Adrb1 in Acta2+ cells in response to the cold could provide important insight into potential mechanisms and therapeutic targets to stimulate cold-induced beiging perhaps in the absence of cold treatment.
The current study demonstrates that several cell types can form multilocular beige-like adipocytes within white adipose depots. This is consistent with other reports that Pdgfra+ perivascular cells as well other non-adipocyte sources generate beige adipocytes (Wang et al., 2013; Lee et al., 2012). Additionally, other studies have suggested that beige and white adipocytes are bi-potential; that is beige adipocytes become white adipocytes but can revert to beige adipocytes in response to a beiging stimulus (Rosenwald et al., 2013; Altshuler-Keylin et al., 2016). Our studies resonate with these previous findings; however, the data herein suggest that a majority of Adrb3-induced beige adipocytes are generated from mature white adipocytes. A potential limitation of these study is that we cannot decipher if these white adipocytes that converted to Adrb3-induced beige adipocytes were once beige. Further studies into genetic program and memory could help elucidate how these cells interconvert in response to sympathetic activation. These studies also raise another interesting question; do beige adipocytes that form in response to cold exposure or by Adrb3 agonists activate thermogenesis in an analogous manner? That is; does cold temperatures invoke the same thermogenic program as Adrb3 agonist? More studies directed at examining beige metabolic properties could provide pivotal insight into which beige stimuli would be more clinically germane regarding energy utilization. Also do these differently induced beige adipocytes have different genetic signatures? Towards this end, a recent study showed that beige adipocytes generated by CL compared to beige adipocytes produced by roscovitine/rosiglitazone had unique genetic signatures suggesting that not all beige adipocytes are created equally (Wang et al., 2016). Hence, further research into the genetic regulation and programs could yield answers to these questions.
In summary, using several genetic models encompassing several proposed sources of Adrb3-induced beige adipocytes, we found that white adipocytes generate most Adrb3-induced beige adipocytes. Further, we found that cold-induced beiging relies on Adrb1 activation, and not Adrb3, to engage and license smooth muscle cells to form beige adipocytes. These data highlight that several cellular sources exist for two different beiging stimuli and that not all beiging stimuli should be considered equal.
Materials and methods
Animals
All animals were maintained under the ethical guidelines of the UT Southwestern Medical Center Animal Care and Use Committee according to NIH guidelines under the protocol number 2016–101336. Mice were housed in a 12:12 light:dark cycle and chow and water were provided ad libitum. Experiments were performed on two or six-month-old male mice unless otherwise stated. For all experiments, mice were randomized to their respective groups without restrictions. All mouse lines were backcrossed on a C57BL/6J- 129S1/SvImJ mixed background for at least nine generations. AdipoTrak mice were previously established in our lab (Tang et al., 2008). C57BL/6J (stock no: 000664), AdiponectinCre-ERT2 (stock no: 025124), Myh11Cre-ERT2 (stock no: 019079), Ppargfl/fl (stock no: 004584), Prdm16fl/fl (stock no: 024992), Rosa26RDTA (stock no: 006331), and Rosa26RRFP (stock no: 007908) mice were obtained from the Jackson Laboratory. Acta2Cre-ERT2 mice were generously provided by Dr. Pierre Chambon. Acta2rtTA mice were generously provided by Dr. Beverly Rothermel. Drs. Sean Morrison and Bill Richardson generously provided the PdgfraCre-ERT2 mice. Ucp1Cre-ERT2 were generously provided by Dr. Eric Olson. Cre recombination was induced by administering one dose of tamoxifen dissolved in sunflower oil (Sigma, 50 mg/Kg interperitoneally injection) for two consecutive days. rtTA activation was induced by Doxycycline (0.5 mg/ml in 1% sucrose) provided in the drinking water and protected from light, and it was changed every 2–3 days. For cold experiments, mice were placed in a 6.5°C cold chamber or maintained at room temperature (23–25°C) for seven days.
Pharmacological administration
Request a detailed protocolCL316,243 was purchased from Tocris and dissolved in water. CL316,243 was administered at one dose (1 mg/Kg/day) for seven consecutive days by interperitoneal injections (IP). Mirabegron was purchased from Cayman Chemical and dissolved in water and was administered at one dose (1 mg/Kg/day) for seven consecutive days by IP. Talinolol was purchased from Cayman Chemical and was dissolved in DMSO. Administration solution was dissolved further in water (DMSO ~5%) and was administered at one dose (1 mg/Kg/day) for five consecutive days by IP. SR59230A was purchased from Sigma-Aldrich and was dissolved in DMSO. Administration solution was dissolved further in water (DMSO ~5%) and was administered at one dose (1 mg/Kg/day) for five consecutive days by IP.
Stromal vascular fractionation isolation and cell culture
Request a detailed protocolNo cell lines were used. Stromal-vascular (SV) cells were isolated from pooled subcutaneous (inguinal, interscapular) white adipose tissues for fractionation, unless indicated otherwise. After 2 hr of slow shaking in isolation buffer (100 mM HEPES pH7.4, 120 mM NaCl, 50 mM KCl, 5 mM glucose, 1 mM CaCl2, 1.5% BSA) containing 1 mg/ml collagenase at 37°C. The suspension was then spun at 800 g for 10 min and the pellet contained a crude SV fraction. The SV pellet was then re-suspended in erythrocyte lysis buffer (0.83% NH4Cl in H2O) for 5 min, spun at 800xg for 5 min. The pellet was washed once in 1X PBS, re-suspended and passed through 40 μm mesh. Isolated SV cells were cultured in DMEM supplemented with 10% FBS with 1% penicillin and streptomycin. White adipogenesis was induced by treating confluent cells with DMEM containing 10% FBS, insulin (0.5 µg/ml), dexamethasone (5 µM), and isobutylmethylxanthine (0.5 mM). To induce beige and thermogenic genes, cells were treated with 5 µM CL316,243 or 5 µM mirabegron for 4 or 24 hr. mRNA was harvested or cells were imaged (Wu et al., 2012). Triglyceride accumulation was performed using a kit from ZenBio (Berry and Noy, 2009; Berry et al., 2010) and manufacturer's protocol was followed.
Flow cytometry
Request a detailed protocolSV cells were isolated as above and washed, centrifuged at 1200 g for 5 min, and analyzed with a FACScans analyzer or sorted with a BD FACS Aria operated by the UT Southwestern Flow Cytometry Core. Data analysis was performed using BD FACS Diva and FlowJo software. For RFP+ sorting, live SV cells from Acta2Cre-ERT2; R26RRFP, or PdgfraCre-ERT2; R26RRFP, or AdiponectinCre-ERT2; R26RRFP mice were sorted based on native fluorescence (RFP). The SV cells from TM-induced Acta2Cre-ERT2 or PdgfraCre-ERT2 (without RFP) control mice were used to determine background fluorescence levels. SV cells were incubated with primary antibodies on ice for 30 min and then washed twice with the staining buffer and incubated with secondary antibody for another 30 min on ice before flow cytometry analysis. Primary antibodies include: rabbit-anti-Pdgfrα (1:100, Santa Cruz Biotechnology).
Quantitative real-time PCR (qPCR)
Request a detailed protocolTotal RNA was extracted using TRIzol (Invitrogen: item no: 15596026) from either mouse tissues or cells. Mouse tissues (n= 6 individual tissues) and cells (n= 4 individual wells) were pooled and analyzed in technical quadruplicates. These experiments were performed on three independent cohorts. cDNA synthesis was performed using RNA to cDNA high capacity kit (Invitrogen: item no: 4387406). Gene expression was analyzed using Power SYBR Green PCR Master Mix with ABI 7500 Real-Time PCR System. qPCR values were normalized by 18 s rRNA expression. Primer sequences are available in Supplementary file 1.
Histological staining
Request a detailed protocolAdipose tissues were fixed in 10% formalin, dehydrated, embedded in paraffin, and sectioned with a Microm HM 325 microtome at 5–15 μm thickness. For immunofluorescence staining, paraffin sections were preincubated with permeabilization buffer (0.3% Triton X-100 in PBS) for 30 min at room temperature and then incubated sequentially with primary antibody (4°C, overnight) and secondary antibody (2 hr at room temperature), all in blocking buffer (5% normal donkey serum in 1X PBS). Antibodies used for immunostaining are: rabbit-anti-Ucp1 (1:500, Abcam), mouse-anti-RFP (1:200, Clontech), mouse-anti-Acta2 (1:500, Abcam), rabbit-anti-Pdgfrα (1:100, Santa Cruz Biotechnology), and goat-anti-Perilipin (1:500, Abcam). Secondary antibodies, Alexa Fluor 488 donkey anti-rabbit, cy3 donkey anti-mouse, and Alexa Fluor 647 donkey anti-goat, were from Jackson ImmunoResearch. All secondary antibodies were used at a 1:500 dilution. For immunohistochemistry staining, slides were deparaffinized and rehydrated before heat-induced antigen retrieval. Antigens were detected using primary antibody and in conjunction with an HRP/DAB (ABC) detection kit (Abcam; ab64264) according to the manufacturer’s instructions (R&D Systems). Immunostained images were collected on a Zeiss LSM500 confocal microscope, or a Lecia DMi6. For quantification of images, two independent observers assessed three random fields in 10 random sections from at least three mice per cohort and used Image J to quantify co-localization.
Metabolic phenotyping experiments
Request a detailed protocolTemperature was monitored daily using a rectal probe (Physitemp). The probe was lubricated with glycerol and was inserted 1.27 centimeters (1/2 inch) and temperature was measured when stabilized. Glucose monitoring: tail blood glucose levels were measured immediately after cold exposure (9am CST) with a Contour glucometer (Bayer).
Statistical analysis
Request a detailed protocolStatistical significance was assessed by an unpaired Student’s t-test using Origin Labs 8.1 software, Excel or GraphPad Prism 6. p<0.05 was considered statistically significant. Mouse experiments were performed in biological triplicate with at least six mice/group and results are expressed as means ± SEM. No power analysis was used to calculate samples size rather sample size was calculated on historical fate-mapping and metabolic studies from the ours and other’s research group. For fate-mapping experiments, a minimum of 6 mice/group were analyzed replicated thrice. For metabolic studies, a minimum of 8 mice/group were analyzed replicated thrice. Experiments were performed on male mice at denoted ages. No mice were excluded from the study unless visible fight wounds were observed. Cell culture experiments were collected from three or four independent cultures for each sample and pooled.
References
-
Challenges in β(3)-adrenoceptor agonist drug developmentTherapeutic Advances in Endocrinology and Metabolism 2:59–64.https://doi.org/10.1177/2042018811398517
-
The emergence of cold-induced brown adipocytes in mouse white fat depots is determined predominantly by white to brown adipocyte transdifferentiationAJP: Endocrinology and Metabolism 298:E1244–E1253.https://doi.org/10.1152/ajpendo.00600.2009
-
Repression of cellular retinoic acid-binding protein II during adipocyte differentiationJournal of Biological Chemistry 285:15324–15332.https://doi.org/10.1074/jbc.M110.110635
-
Increased brown adipose tissue oxidative capacity in cold-acclimated humansThe Journal of Clinical Endocrinology & Metabolism 99:E438–E446.https://doi.org/10.1210/jc.2013-3901
-
Dietary fatty acid metabolism of brown adipose tissue in cold-acclimated menNature Communications 8:14146.https://doi.org/10.1038/ncomms14146
-
Beta-adrenergic, cAMP-mediated stimulation of proliferation of brown fat cells in primary culture. Mediation via beta 1 but not via beta 3 adrenoceptorsThe Journal of Biological Chemistry 267:2006–2013.
-
Brown adipose tissue: function and physiological significancePhysiological Reviews 84:277–359.https://doi.org/10.1152/physrev.00015.2003
-
beta-Adrenergic activation of p38 MAP kinase in adipocytes: cAMP induction of the uncoupling protein 1 (UCP1) gene requires p38 MAP kinaseThe Journal of Biological Chemistry 276:27077–27082.https://doi.org/10.1074/jbc.M101049200
-
Identification and importance of brown adipose tissue in adult humansNew England Journal of Medicine 360:1509–1517.https://doi.org/10.1056/NEJMoa0810780
-
The β3-adrenergic receptor is dispensable for browning of adipose tissuesAmerican Journal of Physiology - Endocrinology And Metabolism 312:E508–E518.https://doi.org/10.1152/ajpendo.00437.2016
-
Regulation of Cre recombinase activity by mutated estrogen receptor ligand-binding domainsBiochemical and Biophysical Research Communications 237:752–757.https://doi.org/10.1006/bbrc.1997.7124
-
Beta3-adrenergic receptors on white and brown adipocytes mediate beta3-selective agonist-induced effects on energy expenditure, insulin secretion, and food intake. A study using transgenic and gene knockout miceThe Journal of Biological Chemistry 272:17686–17693.https://doi.org/10.1074/jbc.272.28.17686
-
Effect of CL-316,243, a thermogenic beta 3-agonist, on energy balance and brown and white adipose tissues in ratsThe American Journal of Physiology 266:R1371–R1382.
-
Beta 3-adrenoceptor knockout in C57BL/6J mice depresses the occurrence of brown adipocytes in white fatEuropean Journal of Biochemistry 270:699–705.https://doi.org/10.1046/j.1432-1033.2003.03422.x
-
Cellular origins of cold-induced brown adipocytes in adult miceThe FASEB Journal 29:286–299.https://doi.org/10.1096/fj.14-263038
-
Brown adipose tissue and its therapeutic potentialJournal of Internal Medicine 276:364–377.https://doi.org/10.1111/joim.12255
-
A smooth muscle-like origin for beige adipocytesCell Metabolism 19:810–820.https://doi.org/10.1016/j.cmet.2014.03.025
-
Beta-adrenoceptor blockers and the blood-brian barrierBritish Journal of Clinical Pharmacology 11:549–553.https://doi.org/10.1111/j.1365-2125.1981.tb01169.x
-
Bi-directional interconversion of brite and white adipocytesNature Cell Biology 15:659–667.https://doi.org/10.1038/ncb2740
-
Brown adipose tissue as a regulator of energy expenditure and body fat in humansDiabetes & Metabolism Journal 37:22–29.https://doi.org/10.4093/dmj.2013.37.1.22
-
Brown and beige fat in humans: thermogenic adipocytes that control energy and glucose homeostasisJournal of Clinical Investigation 125:478–486.https://doi.org/10.1172/JCI78362
-
The adipose organ: white-brown adipocyte plasticity and metabolic inflammationObesity Reviews 13 Suppl 2:83–96.https://doi.org/10.1111/j.1467-789X.2012.01039.x
-
Comparison of talinolol and atenolol effects on blood pressure in relation to lipid and glucose metabolic parameters. Results from the TALIP studyInternational Journal of Clinical Pharmacology and Therapeutics 41:22–29.
-
β(1) Adrenergic receptor is key to cold- and diet-induced thermogenesis in miceJournal of Endocrinology 214:359–365.https://doi.org/10.1530/JOE-12-0155
-
Cold acclimation recruits human brown fat and increases nonshivering thermogenesisJournal of Clinical Investigation 123:3395–3403.https://doi.org/10.1172/JCI68993
-
Cold-activated brown adipose tissue in healthy menNew England Journal of Medicine 360:1500–1508.https://doi.org/10.1056/NEJMoa0808718
-
Recruited brown adipose tissue as an antiobesity agent in humansJournal of Clinical Investigation 123:3404–3408.https://doi.org/10.1172/JCI67803
Article and author information
Author details
Funding
National Institute of Diabetes and Digestive and Kidney Diseases (K01 DK109027)
- Daniel C Berry
National Institute of Diabetes and Digestive and Kidney Diseases (K01 DK111771)
- Yuwei Jiang
National Institute of Diabetes and Digestive and Kidney Diseases (R01 DK088220)
- John M Graff
National Institute of Diabetes and Digestive and Kidney Diseases (R01 DK064261)
- John M Graff
National Institute of Diabetes and Digestive and Kidney Diseases (R01 DK066556)
- John M Graff
The funders had no role in study design, data collection and interpretation, or the decision to submit the work for publication.
Ethics
Animal experimentation: This study was performed in accordance with the recommendations in the Guide for the Care and Use of Laboratory Animals of the National Institutes of Health. All animals were maintained under the approved protocols and ethical guidelines of the UT Southwestern Medical Center Animal Care and Use Committee under the protocol number 2016--101336.
Copyright
© 2017, Jiang et al.
This article is distributed under the terms of the Creative Commons Attribution License, which permits unrestricted use and redistribution provided that the original author and source are credited.
Metrics
-
- 4,480
- views
-
- 820
- downloads
-
- 113
- citations
Views, downloads and citations are aggregated across all versions of this paper published by eLife.
Download links
Downloads (link to download the article as PDF)
Open citations (links to open the citations from this article in various online reference manager services)
Cite this article (links to download the citations from this article in formats compatible with various reference manager tools)
Further reading
-
- Cell Biology
- Developmental Biology
The rete ovarii (RO) is an appendage of the ovary that has been given little attention. Although the RO appears in drawings of the ovary in early versions of Gray’s Anatomy, it disappeared from recent textbooks, and is often dismissed as a functionless vestige in the adult ovary. Using PAX8 immunostaining and confocal microscopy, we characterized the fetal development of the RO in the context of the mouse ovary. The RO consists of three distinct regions that persist in adult life, the intraovarian rete (IOR), the extraovarian rete (EOR), and the connecting rete (CR). While the cells of the IOR appear to form solid cords within the ovary, the EOR rapidly develops into a convoluted tubular epithelium ending in a distal dilated tip. Cells of the EOR are ciliated and exhibit cellular trafficking capabilities. The CR, connecting the EOR to the IOR, gradually acquires tubular epithelial characteristics by birth. Using microinjections into the distal dilated tip of the EOR, we found that luminal contents flow toward the ovary. Mass spectrometry revealed that the EOR lumen contains secreted proteins potentially important for ovarian function. We show that the cells of the EOR are closely associated with vasculature and macrophages, and are contacted by neuronal projections, consistent with a role as a sensory appendage of the ovary. The direct proximity of the RO to the ovary and its integration with the extraovarian landscape suggest that it plays an important role in ovary development and homeostasis.
-
- Cell Biology
- Developmental Biology
Long thought to have little relevance to ovarian physiology, the rete ovarii may have a role in follicular dynamics and reproductive health.