Molecular Chaperones: Confirmation for conformational selection
Almost every process in biology relies on proteins in one way or another, and most of these proteins need to have a specific three-dimensional structure to carry out their roles. A network of molecular machines – called chaperones – makes sure that proteins tend to end up folded correctly (Balchin et al., 2016), and a chaperone called Hsp70 is a central hub in this network.
Hsp70 has a domain that binds to substrates at one end (Mayer and Bukau, 2005), and a domain that binds to nucleotides like ATP at the other end. The chaperone’s affinity for substrates decreases when ATP binds to this domain, and it increases again when the ATP is broken down by hydrolysis to yield ADP. Importantly, Hsp70 does not work alone; another protein called Hsp40 both helps to deliver substrates to Hsp70 and also catalyzes the hydrolysis of ATP.
However, despite much progress in recent years (Clerico et al., 2015; Mayer and Kityk, 2015), major questions remain about the interactions between Hsp70 and its substrates. For instance, does Hsp70 passively bind to exposed segments of unfolded proteins, as proposed in the ‘conformational selection’ hypothesis, or does it actively unfold misfolded substrates, as proposed by the ‘induced fit’ hypothesis? Now, in eLife, Ashok Sekhar, Lewis Kay and colleagues report an answer to this long-standing question (Sekhar et al., 2018).
It has proven challenging to decide between these two hypotheses, partly because chaperones are highly dynamic molecular machines, which limits the number of techniques that can be used to study them in action. Fortunately, NMR spectroscopy provided a perfect solution to tackle this problem (Kay, 2016; Huang and Kalodimos, 2017).
According to the conformational selection hypothesis, the substrates switch between being folded and unfolded, and Hsp70 selectively binds to the unfolded state (Figure 1A). The induced fit hypothesis, however, proposes that Hsp70 binds to a folded or misfolded substrate and then unfolds it (Figure 1B). Therefore, if someone could directly detect a substrate switching between an unfolded state and an Hsp70 bound state, that would be evidence for the conformational selection hypothesis. No such exchange should occur if the induced fit model is correct.
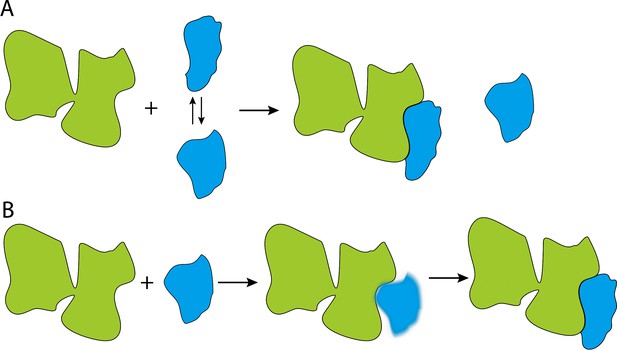
Cartoon illustrations of the conformational selection and induced fit mechanisms.
(A) In the conformational selection mechanism, the substrate (blue) switches between different conformations, and the chaperone (green) selectively interacts with one of these conformations. (B). In the induced fit mechanism, the chaperone directly interacts with the substrate, irrespective of the latter's conformation, and then changes its conformation.
Using advanced NMR methodologies, Sekhar et al. – who are based at the University of Toronto, the Weizmann Institute of Science, the École Normale Supérieure in Paris and the Hospital for Sick Children, also in Toronto – could keep track of two processes – magnetization exchange and chemical exchange – as Hsp70 interacted with a model substrate. Roughly speaking, these two processes can be used to measure conformational changes undergone by the substrate during its interaction with Hsp70.
Sekhar et al. found that Hsp70 from humans and a related bacterial chaperone called DnaK both interact with substrates through the conformational selection mechanism. It was already known that DnaK behaves in a similar way to human Hsp70 (Clerico et al., 2015; Mayer and Kityk, 2015). The results of Sekhar et al. suggest, therefore, that the Hsp70 chaperone machinery prevents the misfolding of proteins by selectively binding to unfolded substrates instead of actively unfolding substrates that have misfolded. The methods reported by Sekhar et al. could also be used to study other chaperone systems such as the Trigger Factor (Saio et al., 2014) and SecB (Huang et al., 2016), both of which capture their substrate proteins in an unfolded state.
In vivo, the Hsp70 machinery needs to process long proteins consisting of more than 300 to 400 amino acids (Balchin et al., 2016). Yet Hsp70 has just one relatively small substrate-binding site that can accommodate only seven to eight amino acids. How can Hsp70 fulfill such a challenging task? It is thought that Hsp40 helps deliver substrates to Hsp70, and so it will be important to examine the exact role that Hsp40 plays in recognizing substrates and delivering them to Hsp70. Will the current model, which works for isolated Hsp70, still apply when Hsp40 and the rest of the Hsp70 machinery are present? Many key questions remain unanswered, yet this latest study gives hope that NMR spectroscopy is well suited to address these questions.
References
-
How Hsp70 molecular machines interact with their substrates to mediate diverse physiological functionsJournal of Molecular Biology 427:1575–1588.https://doi.org/10.1016/j.jmb.2015.02.004
-
Structures of large protein complexes determined by nuclear magnetic resonance spectroscopyAnnual Review of Biophysics 46:317–336.https://doi.org/10.1146/annurev-biophys-070816-033701
-
New views of functionally dynamic proteins by solution NMR spectroscopyJournal of Molecular Biology 428:323–331.https://doi.org/10.1016/j.jmb.2015.11.028
-
Hsp70 chaperones: cellular functions and molecular mechanismCellular and Molecular Life Sciences 62:670–684.https://doi.org/10.1007/s00018-004-4464-6
-
Insights into the molecular mechanism of allostery in Hsp70sFrontiers in Molecular Biosciences 2:58.https://doi.org/10.3389/fmolb.2015.00058
Article and author information
Author details
Publication history
Copyright
© 2018, Jiang et al.
This article is distributed under the terms of the Creative Commons Attribution License, which permits unrestricted use and redistribution provided that the original author and source are credited.
Metrics
-
- 1,607
- views
-
- 196
- downloads
-
- 7
- citations
Views, downloads and citations are aggregated across all versions of this paper published by eLife.
Download links
Downloads (link to download the article as PDF)
Open citations (links to open the citations from this article in various online reference manager services)
Cite this article (links to download the citations from this article in formats compatible with various reference manager tools)
Further reading
-
- Structural Biology and Molecular Biophysics
In addition to its role as cellular energy currency, adenosine triphosphate (ATP) serves as an extracellular messenger that mediates diverse cell-to-cell communication. Compelling evidence supports that ATP is released from cells through pannexins, a family of membrane proteins that form heptameric large-pore channels. However, the activation mechanisms that trigger ATP release by pannexins remain poorly understood. Here, we discover lysophospholipids as endogenous pannexin activators, using activity-guided fractionation of mouse tissue extracts combined with untargeted metabolomics and electrophysiology. We show that lysophospholipids directly and reversibly activate pannexins in the absence of other proteins. Secretomics experiments reveal that lysophospholipid-activated pannexin 1 leads to the release of not only ATP but also other signaling metabolites, such as 5’-methylthioadenosine, which is important for immunomodulation. We also demonstrate that lysophospholipids activate endogenous pannexin 1 in human monocytes, leading to the release of IL-1β through inflammasome activation. Our results provide a connection between lipid metabolism and purinergic signaling, both of which play major roles in immune responses.
-
- Structural Biology and Molecular Biophysics
Integral membrane proteins carry out essential functions in the cell, and their activities are often modulated by specific protein-lipid interactions in the membrane. Here, we elucidate the intricate role of cardiolipin (CDL), a regulatory lipid, as a stabilizer of membrane proteins and their complexes. Using the in silico-designed model protein TMHC4_R (ROCKET) as a scaffold, we employ a combination of molecular dynamics simulations and native mass spectrometry to explore the protein features that facilitate preferential lipid interactions and mediate stabilization. We find that the spatial arrangement of positively charged residues as well as local conformational flexibility are factors that distinguish stabilizing from non-stabilizing CDL interactions. However, we also find that even in this controlled, artificial system, a clear-cut distinction between binding and stabilization is difficult to attain, revealing that overlapping lipid contacts can partially compensate for the effects of binding site mutations. Extending our insights to naturally occurring proteins, we identify a stabilizing CDL site within the E. coli rhomboid intramembrane protease GlpG and uncover its regulatory influence on enzyme substrate preference. In this work, we establish a framework for engineering functional lipid interactions, paving the way for the design of proteins with membrane-specific properties or functions.