Differential requirement of kindlin-3 for T cell progenitor homing to the non-vascularized and vascularized thymus
Abstract
The role of integrin-mediated adhesion during T cell progenitor homing to and differentiation within the thymus is ill-defined, mainly due to functional overlap. To circumvent compensation, we disrupted the hematopoietic integrin regulator kindlin-3 in mice and found a progressive thymus atrophy that is primarily caused by an impaired homing capacity of T cell progenitors to the vascularized thymus. Notably, the low shear flow conditions in the vascular system at midgestation allow kindlin-3-deficient fetal liver-derived T cell progenitors to extravasate via pharyngeal vessels and colonize the avascular thymus primordium. Once in the thymus, kindlin-3 promotes intrathymic T cell proliferation by facilitating the integrin-dependent crosstalk with thymic antigen presenting cells, while intrathymic T cell migration, maturation into single positive CD4 and CD8 T cells and release into the circulation proceed without kindlin-3. Thus, kindlin-3 is dispensable for integrin-mediated T cell progenitor adhesion and signalling at low and indispensable at high shear forces.
https://doi.org/10.7554/eLife.35816.001Introduction
T cell progenitors develop in the fetal liver (FL) or in the bone marrow (BM) and require the specialized environment of the thymus to mature into single positive (SP) CD4 or CD8 T cells (Cai et al., 2007; Foss et al., 2001; Jotereau et al., 1987). FL-derived T cell progenitors start colonizing the thymus anlage at around embryonic day (E) 11.5. Since the thymus anlage is initially avascular, T cell progenitors exit the adjacent pharyngeal vessels, migrate towards the thymic primordium, cross the dense extracellular matrix of the thymic capsule and finally settle in the thymic tissue. After thymus vascularization, which occurs at around E15 (Liu et al., 2006), T cell progenitors enter the thymus by extravasating from post-capillary venules at the cortico-medullary junction. Once in the thymus the progenitor cells interact and receive signals from thymic epithelial cells, macrophages, dendritic cells (DCs) and extracellular matrix proteins, which are essential for their proliferation, differentiation, selection and release into the circulation as mature, single positive T cells (Boehm and Bleul, 2006; Koch and Radtke, 2011).
Leukocyte extravasation follows a cascade of adhesion events commencing with selectin-mediated rolling of leukocytes on endothelial cells followed by the interaction with endothelial cell-bound chemokines, integrin-mediated leukocyte arrest and crawling and finally the transmigration through the vessel wall (Ley et al., 2007). Although integrins are expressed on all hematopoietic cells, conflicting reports exist on their role for T cell progenitor extravasation. While the treatment with function-blocking antibodies against α4 and/or β2 integrins reduced the homing of thymic progenitors to the adult thymus (Rossi et al., 2005; Scimone et al., 2006), genetic ablation of neither α4 (Arroyo et al., 1996), nor αL (Schmits et al., 1996), α6 (Georges-Labouesse et al., 1996), β2 (Scharffetter-Kochanek et al., 1998), β7 integrins (Bungartz et al., 2006; Wagner et al., 1996), or the integrin ligands intercellular adhesion molecule (ICAM)−1 and vascular cell adhesion molecule (VCAM)−1 (Koni et al., 2001; Sligh et al., 1993; Xu et al., 1994) interfered with thymic colonization. Similarly, it is not known whether migration of T cell progenitors within the thymus, or their proliferation and differentiation require integrin-mediated adhesion.
Kindlins are a family of β integrin tail binding proteins that regulate the affinity of integrins for their ligands and induce signal transduction pathways downstream of activated, ligand-bound integrins (Moser et al., 2009b). Kindlins consist of 3 members: kindlin-1,–2, and −3; while kindlin-1 and −2 are widely expressed, hematopoietic cells exclusively express kindlin-3 (Ussar et al., 2006; Weinstein et al., 2003). Consequently, loss of kindlin-3 impairs the functions of all integrins on hematopoietic cells studied so far and leads to severe defects affecting platelets, leukocytes, osteoclasts and hematopoietic stem and progenitor cells (HSPCs) (Krüger et al., 2008; Moser et al., 2009a; Moser et al., 2008; Ruppert et al., 2015; Schmidt et al., 2011). In line with genetic studies in mice, loss-of-function mutations in the human KINDLIN-3/FERMT3 gene cause leukocyte adhesion deficiency type-III (LAD-III) syndrome, which is characterized by severe bleedings, infections and accumulation of HSPCs in the blood circulation (Kuijpers et al., 2009; Malinin et al., 2009; Mory et al., 2008; Ruppert et al., 2015; Svensson et al., 2009).
In the present study, we investigated T-lymphopoiesis in kindlin-3-deficient mice. We found that loss of kindlin-3 protein expression results in progressive thymus atrophy, which is mainly caused by impaired colonization of the vascularised thymus by BM-derived T cell progenitors during late embryogenesis and after birth. In contrast, however, colonization of the non-vascularized thymic primordium by kindlin-3-deficient FL-derived progenitors proceeded without kindlin-3, albeit less efficiently, due to the lower vascular shear flow in embryos. Within the thymus anlage, the proliferation rate of kindlin-3-deficient T cell populations was reduced, while differentiation into mature CD4 and CD8 T cells was unaffected. Thus, these findings clearly show the crucial role of integrins during T cell development. Specifically, in the absence of kindlin-3 only a weak integrin-mediated T cell adhesion can occur, which suffices resistance to low systemic shear forces and enables T cell progenitor homing early during development. However, at later time points during development, when vascular shear forces increase, kindlin-3 is critical to stabilize T cell adhesion on endothelial cells allowing T cell progenitor homing into the thymus.
Results
Loss of kindlin-3 protein leads to progressive thymus atrophy
Kindlin-3 is expressed in CD4/CD8 double negative (DN) and double positive (DP) T cells from wild-type (WT) thymi and SP CD4 and CD8 T cells from WT spleens (Figure 1—figure supplement 1A). To test whether kindlin-3 expression is required for thymopoiesis, we investigated thymus morphology and size in kindlin-3-deficient (Fermt3-/-) mice, which die of severe bleedings and anemia within the first week after birth (Moser et al., 2008). The morphology of Fermt3-/- thymi was normal and the size steadily increased until postnatal day (P) 3 (Figure 1A,B). However, while WT thymi continued to grow postnatally, Fermt3-/- thymi shrank and became atrophic (Figure 1B). Consistently, the cellularity of WT thymi continuously increased during development (Figure 1C), while Fermt3-/- thymi showed an increase in cellularity only until P3, followed by a dramatic decrease at P6 and P8 (Figure 1C). Despite the progressive increase in cellularity until P3 the number of cells was significantly lower in Fermt3-/- thymi compared to controls at all time points analyzed (Figure 1C). Importantly, we did not observe any compensatory expression of kindlin-1 or −2 in kindlin-3-deficient thymi (Figure 1—figure supplement 1B).
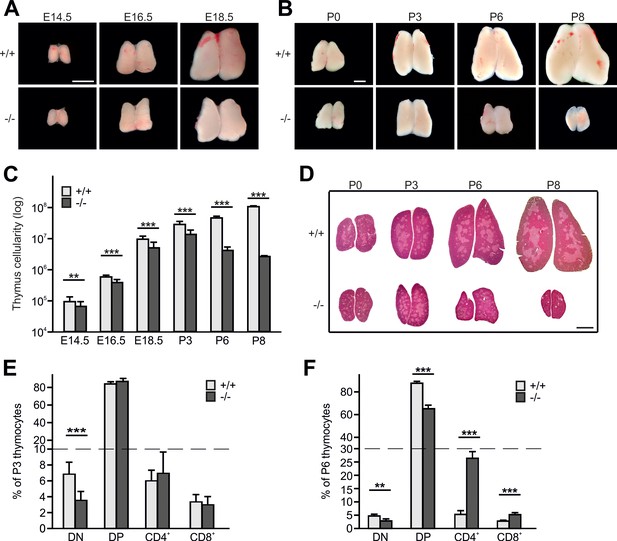
Kindlin-3 deficiency causes thymus atrophy.
(A and B) Images from Fermt3+/+ (upper panel) and Fermt3-/- (lower panel) thymi isolated at the indicated embryonic and postnatal stages. Scale bars 1 mm. (C) Total thymocyte numbers at indicated time points (E14.5 to P8). N(E14.5)=28/28; N(E16.5)=12/12; N(E18.5)=30/21; N(P3)=6/4; N(P8)=3/2. (D) Haematoxylin and Eosin staining of paraffin-embedded thymus sections. Scale bar 1 mm. (E and F) Frequencies of CD4 and CD8 double negative (DN), double positive (DP) and single positive CD4 and CD8 thymocytes isolated from P3 and P6 thymi. N(P3)=16/18; N(P6)=8/4. Bars indicate means ± standard errors. **p<0.01; ***p<0.001. See also Figure 1—figure supplement 1.
Hematoxylin/eosin (H/E) staining of thymic section at different stages of development revealed a similar formation of medullary islets in P0 WT and Fermt3-/- thymi that enlarged and fused at P3 to produce a large central medulla surrounded by the cortex (Figure 1D). In line with the normal thymic cyto-architecture at P3, Fermt3-/- thymi displayed normal cortico-medullary junctions (CMJ) that separate CD4/CD8 DP T cells in the cortex from SP CD4 and CD8 T cells in the medulla, and normal epithelial meshes and vascular networks (Figure 1—figure supplement 1C). At P6 and P8, however, the atrophic Fermt3-/- thymi showed a progressive enlargement of the medulla and a strong reduction of the cortex suggesting an imbalanced development towards more mature SP T cells (Figure 1D). This observation was confirmed by flow cytometry showing that DN T cells containing the most immature intrathymic T cells were significantly reduced at P3 and P6 in Fermt3-/- thymi compared to controls. Notably, while the relative distribution of DP and SP CD4 and CD8 T cells was still comparable between WT and Fermt3-/- thymi at P3, the relative numbers of DP T cells decreased and SP CD4 and CD8 T cells increased in P6 thymi of Fermt3-/- mice (Figure 1E,F). However, despite the shift towards SP T cell populations in P6 Fermt3-/- thymi, their absolute numbers were still significantly reduced compared to controls (Figure 1—figure supplement 1D,E). The presence of normal relative SP T cell numbers in Fermt3-/- thymi until P3 suggests that intrathymic T cell differentiation proceeds independently of kindlin-3. This observation was supported by the normal levels of T cell receptor (TCR), CD3 and costimulatory molecules such as CD5 and CD24 on DP and SP T cells (Figure 1—figure supplement 1F).
Thymocyte proliferation depends on kindlin-3 expression
To test whether the number of apoptotic thymocytes is altered within the P3 thymus we immunostained thymic sections for cleaved caspase-3 (Figure 2A) and analysed thymocytes by flow cytometry after annexin V and 7-AAD (7-Aminoactinomycin) staining (Figure 2B). We found that neither the number (Figure 2C) nor the frequency (Figure 2D) of apoptotic cells (annexin V+/7-AAD-) was altered in Fermt3-/- thymi. Furthermore, the different T cell subsets isolated from WT and Fermt3-/- thymi showed a comparable annexin V staining (Figure 2E).
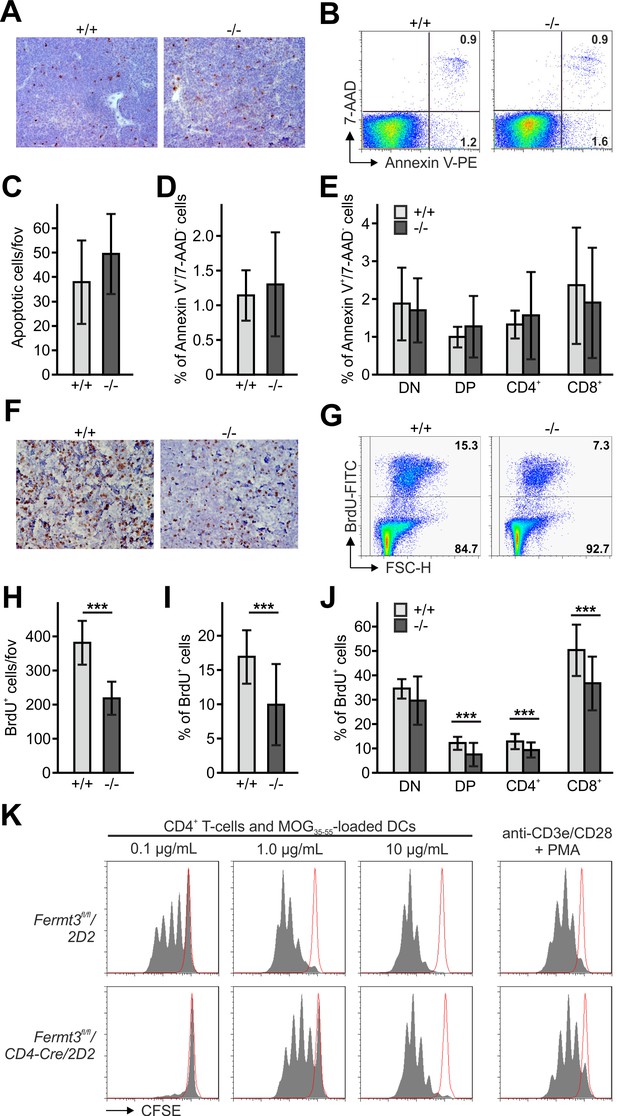
Reduced thymocyte proliferation contributes to thymus atrophy of kindlin-3-deficient mice.
(A) Staining for cleaved caspase-3 counterstained with Mayer´s haematoxylin on paraffin sections of Fermt3+/+ and Fermt3-/- P3 thymi. Scale bar 100 µm. (B) Single-cell suspensions of thymocytes from control and Fermt3-/- P3 thymi were stained with the apoptotic (Annexin V) and dead cell (7-AAD) markers. Numbers within the FACS blots represent percent of cells within each quadrant. (C) Number of apoptotic cells per field of view (fov) observed in (A). N = 10. Percentage of early apoptotic cells (Annexin V+, 7-AAD-) in control and Fermt3-/- thymi (D) and in distinct T cell subpopulations (CD4/CD8 DN, CD4/CD8 DP, CD4 single positive and CD8 single positive) (E). N = 7/10. (F) Sections of Fermt3+/+ and Fermt3-/- P3 thymi stained for BrdU incorporation and counterstained with Mayer´s haematoxylin. Scale bar 100 µm. (G) BrdU incorporation in thymocytes analysed by flow cytometry. (H) Numbers of BrdU positive cells per field of view (fov) observed in (F). N = 8/6. (I) Percentage of proliferating, BrdU positive thymocytes measured by flow cytometry. N = 14. (J) Percentage of BrdU positive cells in distinct T cell subpopulations. N = 14. (K) CD4 T cells isolated from spleens of control Fermt3fl/fl/2D2 and Fermt3fl/fl/2D2/CD4Cre mice were stained with CFSE and stimulated either with DCs loaded with different concentrations of MOG35-55 peptide or primed with anti-CD3e/CD28 antibodies and PMA. Representative histograms show CSFE dilution. Red-lined histograms represent cells incubated with not-loaded DCs or no antibodies. Bars indicate means ± standard errors. **p<0.01; ***p<0.001. See also Figure 2—figure supplement 1.
Next, we determined thymocyte proliferation by in vivo bromodeoxyuridine (BrdU) labelling, which revealed a significantly reduced number of BrdU positive cells in P3 Fermt3-/- thymi both by immunohistochemistry (Figure 2F,H) and flow cytometry (Figure 2G,I). Notably, reduced proliferation was detected in both the DP and the SP T cell populations (Figure 2J). Since thymocyte proliferation relies on proper contact with antigen presenting cells (APC), we tested kindlin-3-deficient T cells for their ability to form immune synapses (IS) with WT DCs. To this end we loaded mature DCs with MOG35-55 peptide and added splenic CD4 T cells from Fermt3+/fl and Fermt3fl/fl mice, which express a TCR transgene specific for the myelin oligodendrocyte glycoprotein (MOG) 35–55 peptide (2D2), a double-fluorescent reporter transgene for measuring Cre activity (mTmG) and the CD4-Cre transgene (Bettelli et al., 2003; Moretti et al., 2013; Muzumdar et al., 2007). After 30 min the T-DC contacts were analysed by immunofluorescence staining. Although Fermt3-/- T cells formed fewer contacts with DCs compared to control T cells, recruitment of LFA-1 and actin to the contact area as well as phospho-Tyrosine signals were indistinguishable between them suggesting that Fermt3-/- T cells form IS with DCs (Figure 2—figure supplement 1). To study APC-induced T cell proliferation in vitro, we co-cultured MOG35-55 peptide-loaded dendritic cells with CSFE-labelled control and kindlin-3-deficient T cells derived from Fermt3fl/fl/CD4-Cre/2D2 mice, and measured CSFE dilution by flow cytometry. In line with the observation that Fermt3-/- T cells can form contacts with DCs, a comparable proliferative response was observed when these T cells were stimulated by DCs loaded with high concentration of MOG35-55 peptide or when a strong TCR signal was induced directly with anti-CD3/CD28 antibodies. In contrast, Fermt3-/- T cells failed to proliferate when dendritic cells were loaded with lower concentrations of MOG35-55 peptide (Figure 2K) indicating that defective integrin-mediated contact between kindlin-3-deficient T cells and APCs causes a reduced proliferative response contributing to thymus atrophy of kindlin-3-deficient mice.
T cell progenitor homing to the postnatal thymus
The reduced CD4 and CD8 DN T cell numbers in Fermt3-/- thymi (Figure 1E,F and Figure 1—figure supplement 1D,E) point to an impaired T cell progenitor homing to the thymus. Upon homing of T cell progenitors to the thymus, they progress through four DN stages before developing into DP cells. To test whether loss of kindlin-3 affects the early and/or late stages of DN development, we separated lineage-positive thymic cells from lineage-negative (Linneg) DN cells by flow cytometry and gated the latter cell population for high and low CD44 and c-kit expression into DN stage 1 and 2 (DN1-2) and DN stage 3 and 4 (DN3-4) cells, respectively. In parallel, we gated DN cells for high and low CD44 and CD25 expression to discriminate between the four DN stages. These experiments revealed that the Linneg DN population was significantly reduced in P3 and P6 thymi and the DN1 and DN2 stage thymocytes were virtually absent from P3 Fermt3-/- thymi (Figure 3A and Figure 3—figure supplement 1). DN1 stage cells are the immediate intrathymic descendants of circulating hematopoietic progenitor cells (Linneg, c-kit+, Sca-1- and Sca-1+), which accumulate in the blood circulation of Fermt3-/- mice suggesting that extravasation of Fermt3-/- T cell progenitors is impaired (Figure 3B,C). To test this hypothesis experimentally, we transferred FL cells from E14.5 WT and Fermt3-/- embryos into RAG2-deficient (Rag2-/-) mice, in which T cell development is blocked at the DN3 stage due to loss of V(D)J rearrangement (Shinkai et al., 1992) and analysed size, weight and cellularity of the chimeric thymus and T cell frequency in the peripheral blood 8 to 10 weeks later. The transfer of Fermt3-/- FL cells failed to reconstitute T cell development in the thymus of Rag2-/- recipient mice (Figure 4A–C), which also contained almost no DN1-2 cells (Figure 4D,E). Interestingly, a very small number of Fermt3-/- T cell progenitors entered the Rag2-/- recipient thymus, differentiated into DN4 cells (Figure 4D,F) and produced a small number of circulating CD4 and CD8 T cells, which homed to the spleen of Rag2-/- mice (Figure 4G–K) indicating that kindlin-3 is very important for T cell progenitor homing to the adult thymus, but once in the thymus the progenitor cells differentiate into mature SP T cells that are released into the circulation.
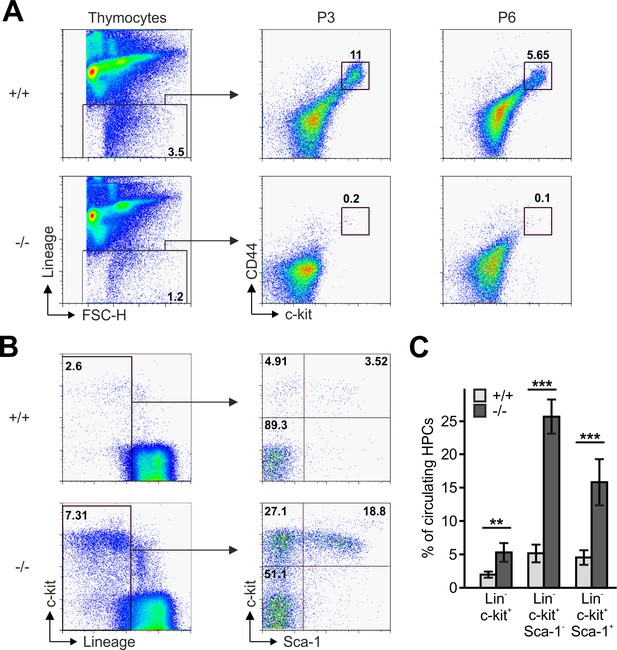
Reduced frequencies of T cell progenitors in Fermt3-/- thymi.
(A) Thymocytes from Fermt3+/+ and Fermt3-/- mice were stained for lineage markers (B220, CD19, TER119, NK1.1, CD11b, Gr-1, CD8α, CD3e, TCRβ, TCRγδ and CD11c), CD44 and c-kit to identify DN1-2 (Linneg, c-kithi, CD44hi) and the DN3-4 (Linneg, c-kitlow, CD44low) populations. (B and C) PB from Fermt3+/+ and Fermt3-/- animals (P3) were stained for lineage markers (TER119, B220, CD11b, Gr-1, CD11c, NK1.1, CD4 and CD8α), c-kit and Sca-1 to identify hematopoietic progenitor cells and analysed by flow cytometry. N = 4. Numbers within the representative FACS plots indicate cell percentages. Bars indicate means ± standard errors. **p<0.01; ***p<0.001. See also Figure 3—figure supplement 1.
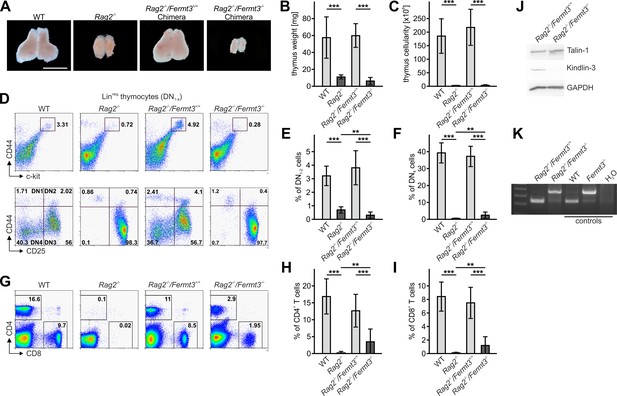
Kindlin-3-deficient T cell progenitors are severely impaired in seeding the adult thymus.
(A–C) Images (A), weights (B) and cell numbers (C) of thymi from adult WT and Rag2-/- mice and Rag2-/- mice 10 weeks after reconstitution with Fermt3+/+ and Fermt3-/- fetal liver cells. Scale bar 5 mm. N = 10. (D) Single-cell suspensions were stained for lineage markers (B220, CD19, TER119, NK1.1, CD11b, Gr-1, CD11c, CD8α, CD3e, TCRβ, and TCRγδ), c-kit, CD44 and CD25 to identify the DN1-2 (Linneg, c-kithi, CD44hi) and the DN3-4 (Linneg, c-kitlow, CD44low) populations (upper panel), and DN1 (Linneg, CD44hi, CD25-), DN2 (Linneg, CD44hi, CD25+), DN3 (Linneg, CD44low, CD25+) and DN4 (Linneg, CD44low, CD25-) populations (lower panel) by flow cytometry. Frequencies of DN1-2 (E) and DN4 (F) thymocytes. N = 8–10. (G) Analysis of peripheral blood T-lymphocytes. (H and I) Frequencies of circulating CD4+ and CD8+ T cells calculated from flow cytometric analyses shown in (G). N = 10. Numbers within the representative FACS plots indicate cell percentages. (J) Expression of talin-1 and kindlin-3 in CD4+ T cells isolated from the spleen of Rag2-/- mice after reconstitution with Fermt3+/+ and Fermt3-/- fetal liver cells. GAPDH serves as loading control. (K) genomic PCR of CD8+ T cells sorted from the spleen of Rag2-/-/Fermt3+/+ and Rag2-/-/Fermt3-/- chimeras. Numbers within the representative FACS plots indicate cell percentages. Bars indicate means ± standard errors. **p<0.01; ***p<0.001. See also Figure 4—figure supplements 1 and 2.
Since kindlin-3-deficient HSCs show an approximately 5-fold reduced homing capacity to the bone marrow, which might eventually impact on T cell progenitor generation (Ruppert et al., 2015), we conditionally deleted Fermt3 by injecting polyIC into Fermt3fl/fl/Mx1-Cre mice and detected almost no DN (Linneg) cells in their thymi, whereas control thymi from polyIC-treated Fermt3fl/fl mice clearly contained a discrete population of DN1-2 stage T cells (Figure 4—figure supplement 1A). In addition, we generated mixed FL cell chimeras by transferring nine times more Fermt3-/- FL cells than WT FL cells into lethally irradiated recipient mice and compared their homing capacity to the thymus. To discriminate between both cell populations the WT FL cells were isolated from B6.SJL embryos, which express the CD45.1 allelic variant, while the Fermt3-/- embryos are from a C57BL/6 background expressing CD45.2. Despite the excess in transferred kindlin-3-deficient FL cells, only around 25% of the DN cells were of CD45.2 origin. As a control, chimeras made from 90% WT CD45.2 cells and 10% WT B6.SJL CD45.1 cells showed the 9:1 ratio in DN and all later T cell stages (Figure 4—figure supplement 1B). Moreover, to exclude a defect in a non-hematopoietic cell type as cause for the Fermt3-/- T cell progenitor homing defect, we analysed thymi from Kindlin-3-EGFP knockin mice, which express kindlin-3 tagged C-terminally with EGFP. Flow cytometry of their thymi revealed that only CD45+ leukocytes were EGFP-positive and that CD31+ endothelial cells and EPCAM+ thymic epithelial cells were EGFP negative (Figure 4—figure supplement 2). Altogether, these experiments show that kindlin-3-deficient T cell progenitors have a strong defect in homing to the postnatal and adult thymus and that the homing defect is of hematopoietic origin.
T cell progenitor homing to the thymus primordium
Since thymi of newborn Fermt3-/- mice lacked DN1 and DN2 cells but contained DN3 and DN4 cells (Figure 3A) we surmised that during development significant numbers of T cell progenitors from the FL must be able to colonize the fetal thymus. To test this hypothesis, we determined numbers and locations of CD45+ hematopoietic progenitor cells in sections of the thymus primordium at different time points. In line with previous reports (Douagi et al., 2000; Itoi et al., 2001), we observed the first wave of WT CD45+ progenitor cells within the perithymic mesenchyme at E11.5, and initial migration into the thymus primordium at E12.0 and scattering throughout the thymus at E12.5 and E13.5 (Figure 5A–F). Interestingly, also Fermt3-/- progenitor cells could be detected in the thymus primordium and the surrounding mesenchyme, however at lower numbers (Figure 5A–E). Despite the reduction, CD45+ progenitor cells accumulated at E13.5 in the Fermt3-/- thymus (Figure 5D) and continued developing into DN1-2 cells (Figure 5F). Strikingly, a dramatic drop in the DN1-2 population was observed in Fermt3-/- thymi at E15-16 (Figure 5G,H and Figure 5—figure supplement 1A,B).
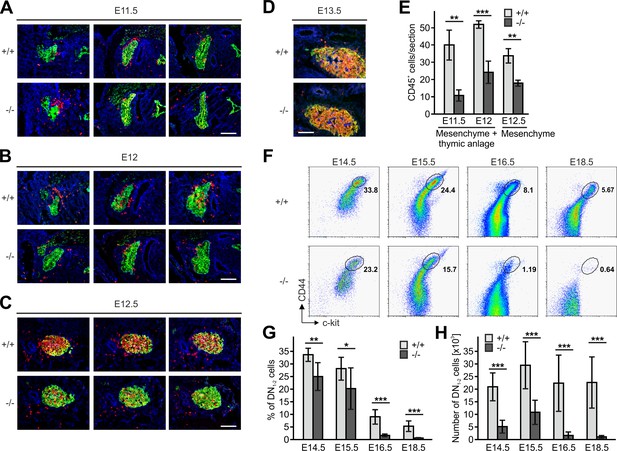
Kindlin-3-independent colonization of the fetal thymus.
(A–D) Sagittal sections of whole embryos at gestational ages E11.5 (A), E12 (B), E12.5 (C) and E13.5 (D) were stained for fibronectin (perithymic mesenchyme, blue), cytokeratin (thymic primordium, green) and CD45 (potential T cell progenitor cells, red). Scale bars 100 µm. (E) Numbers of CD45+ cells within the thymic primordium and in the perithymic mesenchyme at the indicated time points. N = 3–4. (F) Representative flow cytometric profiles of fetal thymocytes from control and Fermt3-/- thymi at the indicated time points stained for lineage markers (B220, CD19, TER119, NK1.1, CD11b, Gr-1, CD11c, CD8α, CD3e, TCRβ, and TCRγδ), c-kit and CD44 to identify the DN1-2 (Linneg, c-kithi, CD44hi) and the DN3-4 (Linneg, c-kitlow, CD44low) populations. Numbers within the representative FACS plots indicate cell percentages. Frequencies (G) and total number (H) of DN1-2 cells measured in the flow cytometric analyses shown in (F). N = 7–10 Bars indicate means ± standard errors. *p<0.05; **p<0.01; ***p<0.001. See also Figure 5—figure supplement 1.
Next we tested whether an abnormal Fermt3-/- progenitor cell development in the FL or release from the FL into the circulation caused the reduced colonization of the thymus primordium. The flow cytometric analyses revealed that the absolute numbers of Linneg, c-kithi, CD45+, PIR+ T cell progenitors were comparable between WT and Fermt3-/- FLs, while their frequency, due to a decreased cellularity of Fermt3-/- FLs, was increased (Figure 6A–C). The numbers of circulating WT and Fermt3-/- Linneg, c-kithi, PIR+ T cell progenitors increased between E11.5 to E12. However, while the frequency of WT T cell progenitors steadily decreased between E12 and E13.5, the T cell progenitors accumulated in the circulation of Fermt3-/- fetuses due to their reduced extravasation from pharyngeal vessels and/or impaired migration towards the thymus primordium (Figure 6D,E).
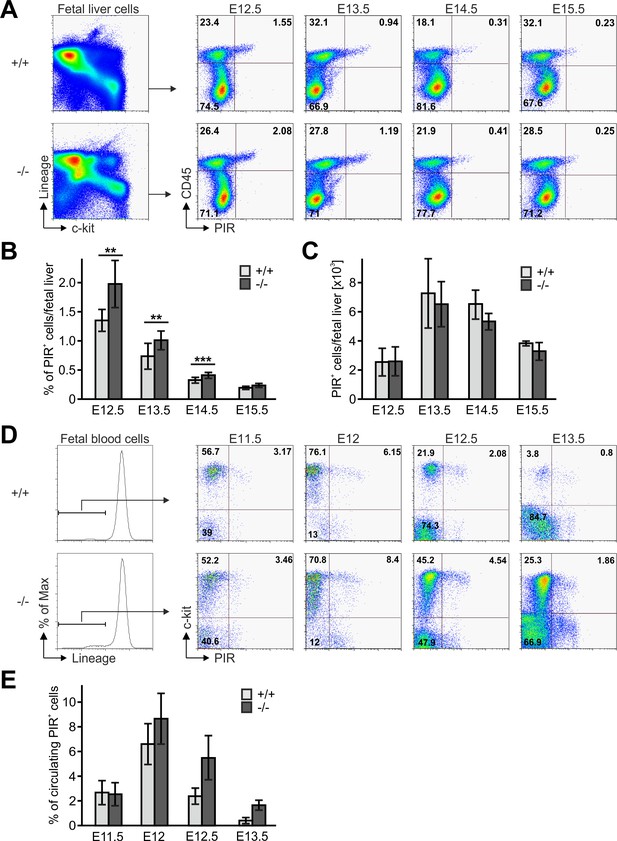
Fetal liver-derived T cell progenitors accumulate in the blood.
(A) Fetal liver cells from control and Fermt3-/- embryos from indicated gestational stages were stained for lineage markers (B220, CD19, TER119, NK1.1, Gr-1 and Thy1.2), c-kit, CD45 and PIR and analysed by flow cytometry. Frequencies (B) and total numbers (C) of PIR+ T cell progenitors per fetal liver. N(E12.5)=7/6; N(E13.5)=8/5; N(E14.5)=10/11; N(E15.5)=4/7. (D) FB from Fermt3+/+ and Fermt3-/- embryos were stained for lineage markers (B220, CD19, TER119, NK1.1, Gr-1 and Thy1.2), c-kit and PIR and analysed by flow cytometry. (E) Frequencies of circulating PIR+ T cell progenitors determined in (D). N(E11.5)=5; N(E12)=8/3; N(E12.5)=4/5; N(E13.5)=7/6. Numbers within the representative FACS plots indicate cell percentages. Bars indicate means ± standard errors. *p<0.05; **p<0.01; ***p<0.001.
T cell progenitor homing to the thymus is directed by chemokine gradients produced by thymic epithelial cells (Koch and Radtke, 2011). Therefore, we decided to test whether Fermt3-/- progenitors are able to sense the chemokine gradient released by thymic lobes in vitro and to invade the thymus. To this end, thymic lobes from WT E15.5 SJL embryos (CD45.1+) were depleted from their T cells with deoxyguanosine (dGuo) and then positioned in a collagen matrix in close proximity to FL-derived Linneg cells from WT or Fermt3-/- E14.5 C57BL/6 (CD45.2+) embryos (Figure 7A). Progenitor migration towards the thymic lobes was monitored for 36 to 48 hr by time-lapse video microscopy, which revealed that WT as well as Fermt3-/- FL cells moved persistently towards the thymic lobes, while migration was not induced when thymic lobes were absent (Figure 7B). To further test whether FL-derived T cell progenitor cells had invaded the thymus capsule and developed into mature T cells ex vivo, the lobes were recovered from the collagen matrix and cultured for additional 18 days under conventional fetal thymus organ culture (FTOC) conditions (Figure 7C). Consistent with our in vivo data, CD45.2+ Fermt3-/- donor cells normally developed into DP, CD4 and CD8 SP cells (Figure 7D).
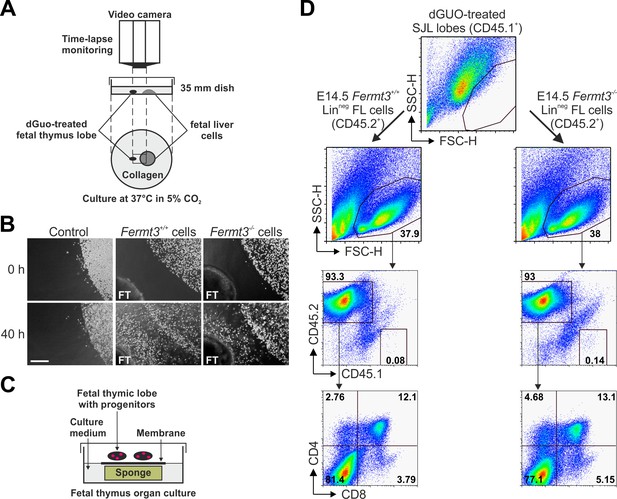
Kindlin-3-deficient T cell progenitors home to the fetal thymus and further develop into SP T cells ex vivo.
(A) Schematic description of the thymus attraction experiment. (B) Representative images of the thymus attraction experiment at the beginning and 40 hr after starting the experiment. Linneg fetal liver cells from control or Fermt3-/- embryos (both CD45.2+) are seen in the upper right corner of the images, a dGuo-treated wild-type fetal thymus lobe (FT, CD45.1+) is located at the lower left corner. Scale bar 250 µm. (C) Schematic description of the fetal thymus organ culture. (D) dGuo-treated fetal thymus lobes seeded with fetal liver cells as performed in (B) were further cultured for 18 days. Cells were stained for CD45.1, CD45.2, CD4, and CD8. Numbers within the representative FACS plots indicate cell percentages.
Altogether these findings indicate that Fermt3-/- T cell progenitors normally develop in the FL, are released into the circulation, sense the chemokine gradient, leave the pharyngeal vessel, albeit less efficiently than WT T cell progenitors, migrate towards the thymus primordium, invade the thymus capsule and differentiate into different T cell subsets.
Blood flow velocity modulates Fermt3-/- T cell progenitor adhesion to vascular walls
The differential efficacy of Fermt3-/- T cell progenitor homing to the avascular and vascularized thymus was neither due to different kindlin-3 and talin-1 protein levels in lysates from sorted FL-derived T progenitor cells (Linneg, c-kit+, PIR+) isolated from E13.5 embryos and DN1 cells (Linneg, CD44+, CD25-) isolated from P6 thymi nor from an upregulation of talin-1 in Fermt3-/- PIR+ T cell progenitors (Figure 8A,B). Flow cytometry also excluded differences in surface expression of α4, αL, β1, β2, β3 and β7 integrins on FL-derived T progenitor cells (Linneg, c-kit+, PIR+) (Figure 8—figure supplement 1) and BM-derived LSK cells (Ruppert et al., 2015). Similarly, the CD31-positive vascular network was indistinguishable in E13.5 and E15.5 thymi from WT and Fermt3-/- mice excluding the possibility that a potential kindlin-3 expression in endothelial cells (Bialkowska et al., 2010) accounts for the defective homing to the vascularized thymus (data not shown). Since adhesion and extravasation of Fermt3-/- T cells can occur on inflamed endothelium expressing high levels of VCAM-1 and ICAM-1 (Manevich-Mendelson et al., 2009; Moretti et al., 2013), we determined the expression of VCAM-1, ICAM-1 and ICAM-2 by immunostaining and flow cytometry and found them even lower expressed in pharyngeal vessels compared to intrathymic vessels. Thus this also excludes that an elevated adhesion molecule expression on pharyngeal vessels compared to intrathymic vessels accounts for the better adhesion and extravasation (Figure 8—figure supplement 2A–E).
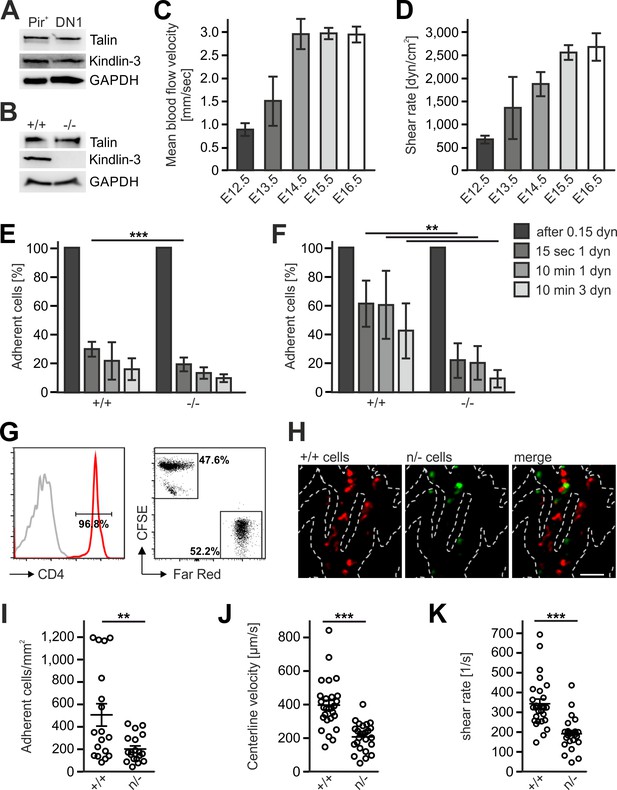
Kindlin-3 is important to stabilize the adhesion of T cell progenitors and mature T cells to vascular integrin ligands when blood flow velocities and shear rate levels increase during development and in vessel segments of higher blood flow within lymph nodes.
(A) Talin and kindlin-3 expression in FACS-sorted Pir+ T cell progenitor cells from WT FL of E13.5 embryos compared to DN1 cells sorted from the thymus of P6 mice. GAPDH served as loading control. (B) Talin and kindlin-3 expression in control and kindlin-3-/- Pir+ T cell progenitor cells isolated from E13.5 FL. GAPDH served as loading control. Mean blood flow velocities (C) and shear rates (D) within the yolk sac vasculature of E12.5 to E16.5 embryos were determined by intravital microscopy. N = 14/3/8/21/18. (E and F) Relative adhesion of Pir+ T cell progenitor cells FACS-sorted from the FLs of control and Fermt3-/- E13.5 embryos on ICAM-1, P-selectin, CCL21 and CCL25 or on VCAM-1, P-selectin, CCL21 and CCL25 coated ibidi flow chambers with stepwise increasing shear rates. N ≥ 5. Bars indicate means ± standard deviation. (G) Purity of CD4+ T cells from WT and Fermt3 hypomorphic (n/-) mice that have been labelled with CFSE and Far Red and mixed in a 1:1 ratio. Grey line represents isotype control. (H,I) Adhesion of CD4+ T cells in vivo. (H) Representative microscopic images of adherent (+/+, red) and (n/-, green) cells in the lymph node vasculature after adoptive transfer. Sum intensity Z projections of confocal stacks are shown. Segmented lines indicate vessel outlines. Scale bar = 50 µm. (I) Quantification of adherent CD4+ T cells (N = 18–19 vessels from three mice). (J, K) Microvascular blood flow in the lymph node vasculature. (J) Centerline blood flow velocity and (K) vascular shear rate in LN microvessel segments (N = 25–27 field of views from three mice). Bars indicate means ± standard deviation. **p<0.01; ***p<0.001. See also Figure 8—figure supplements 1 and 2.
Integrin-mediated adhesion of T cells to endothelial adhesion molecules is influenced by the existing vascular shear stress (Moretti et al., 2013). We therefore hypothesized that Fermt3-/- T cell progenitors show impaired adhesion to the vascular endothelium when blood flow velocity and shear forces increase during intrauterine development. To test this hypothesis, we injected red fluorescent microbeads into the yolk-sac vasculature of E12.5 to E16.5 embryos and measured blood flow velocity by intravital microscopy. We found an increase in blood flow velocity (Figure 8C) and shear rates (Figure 8D) between E12.5 to 16.5. Accordingly, in vitro flow chamber assays revealed that isolated T cell progenitors (Linneg, c-kit+, CD45+, Pir+) hardly adhered to P-selectin, CCL21/CCL25 and ICAM-1 (Figure 8E), while they readily adhered to P-selectin, CCL21/CCL25 and VCAM-1 in a shear stress-dependent manner. Interestingly, Fermt3-/- T cell progenitors adhered to VCAM-1 but displayed a significantly reduced shear force resistance (Figure 8F) suggesting that the increase in blood flow velocity and shear forces during fetal development prevents Fermt3-/- T cell progenitor adhesion and extravasation at intrathymic vessels. To demonstrate that a strong reduction in kindlin-3 expression only allows adhesion of T cells to the vascular surface at sites with lower shear rates, we adoptively transferred a 1:1 ratio of CSFE and Far Red-labelled CD4 T cells from spleens and LNs of WT and Fermt3 hypomorphic mice (K3n/-), respectively, into recipient mice and analysed their adhesion to the popliteal LN vasculature by spinning disc confocal microscopy (Figure 8G,H). Fermt3 hypomorphic mice express only 5% kindlin-3 protein and therefore show a strong defect in leukocyte adhesion (Klapproth et al., 2015). As expected, we observed a reduced number of adherent Fermt3 hypomorphic T cells in the LN vasculature compared to WT cells (Figure 8H,I). We then injected fluorescent microspheres and measured the blood flow velocities in LN vessel segments and determined shear rates adherent cells were exposed to in those vessels. We found that Fermt3 hypomorphic cells adhered preferentially in vessel segments where blood flow velocity and shear rates were lower compared to WT T cells. The latter adhered to vessel segments with higher blood flow velocities and shear rates (Figure 8J,K). These findings indicate that kindlin-3 is crucial to stabilize integrin-mediated T cell adhesion to vessel walls exposed to high vascular shear forces.
Discussion
In the present study, we used mice lacking the essential integrin regulatory protein kindlin-3 to address the role of integrin-mediated adhesion and signalling during T cell progenitor homing to the thymus and subsequent differentiation within the thymus. We found that in the absence of kindlin-3 a well-organized thymus with normal thymus architecture consisting of the four major compartments (subcapsular zone, cortex, medulla and cortico-medullary junction) is initially formed. The presence and clear separation of DP thymocytes in the cortex from SP CD4 and CD8 T cells in the medulla of postnatal thymi indicate that chemokine-driven migration and positioning of developing T cells to specialized thymic microdomains is not impaired in the absence of kindlin-3 allowing a well-orchestrated developmental maturation from T cell progenitors to mature T cells. Thus, our data indicate that chemokine sensing and leukocyte migration within a 3D environment can occur in the absence of kindlin-3´s role to regulate integrin activity, which is in line with a previous report (Lämmermann et al., 2008).
While early thymic development is hardly affected by the lack of kindlin-3, the thymi from kindlin-3 deficient mice began shrinking after birth. We found two main reasons for the thymic atrophy. First, kindlin-3-deficient thymocytes exhibited reduced proliferation rates due to a weakened interaction with thymic APCs. A weakened interaction with APCs was also reported for CD4 T cells isolated from LFA-1 and talin-1 knockout mice to cause reduced T cell proliferation (Graf et al., 2007; Kandula and Abraham, 2004; Wernimont et al., 2011).
Secondly, we observed a severe T cell progenitor homing defect, which becomes apparent at the end of embryogenesis. T cell development in the thymus requires a periodic homing of FL or BM-derived T cell progenitors. The trafficking routes taken by T cell progenitors to the thymus depend on the vascularisation state of the thymus. After vascularization at E15/E16 (Liu et al., 2006), FL and later on BM-derived lymphoid progenitors directly enter the thymus at large post-capillary venules at the thymus cortico-medullary junction. This process is critically dependent on kindlin-3. Furthermore, our findings also show that kindlin-3-dependent adhesiveness through multiple integrin classes expressed on T lymphoid progenitors is essential for their homing to the vascularized thymus, while loss of individual integrins either did not or only partially diminish precursor T cell homing (Scimone et al., 2006).
Our findings also revealed that kindlin-3-deficient T cell progenitors were able to colonize the non-vascularized thymus primordium and develop into SP CD4 and CD8 T cells that are released into the circulation and found in the spleen. Although early colonization of the thymus anlage was slightly delayed and less efficient, kindlin-3-deficient T cell progenitors were capable to leave the pharyngeal vessels and migrate through the mesenchymal tissue before they reach and enter the thymic anlage. The reduced homing performance of kindlin-3-deficient T cell progenitors was neither due to their reduced production in the FL and/or release into the circulation, nor due to a defect in sensing chemokine cues that direct them towards the thymic primordium. So, we assume that reduced adhesion to the pharyngeal vessel wall accounts for the mild T cell progenitor homing defect of kindlin-3-deficient embryos, an assumption, which is also supported by the accumulation of T cell progenitors in the embryonic circulation.
Why can T cell progenitors extravasate from pharyngeal vessels at early developmental stages but fail to do so from post-capillary venules at the cortico-medullary junction of the vascularized thymus? We cannot fully exclude that differences in the expression of other cell surface receptors, such as CD44 or selectin ligands, may exist between FL and BM-derived T cell progenitors, which allow kindlin-3/integrin independent adhesion and extravasation. However, since impaired adhesion and extravasation becomes evident already before E16, a time point at which BM-derived T cell progenitors have not formed yet, we do not believe that this can explain the homing defect. The fact that FL-derived T cell progenitors are initially capable but later fail to home to the thymus suggests that cell extrinsic rather than cell intrinsic factors are responsible for the impaired colonization. Differences in thymus vascularization might be an obvious possibility, especially since a previous report suggested kindin-3 expression in endothelial cells (Bialkowska et al., 2010). However, we found normal vascularization of the thymus anlage in the absence of kindlin-3 and could show that thymic endothelial cells do not express kindlin-3. Since kindlin-3-deficient thymi exhibited a dramatic drop in the number of DN1 and DN2 cells between E15 to E16, which is the time point when the thymus becomes vascularized (Liu et al., 2006), we suggest that differences in the adhesive properties of pharyngeal vs. intrathymic vessels account for this phenomenon. In support of this hypothesis, we reported that kindlin-3-deficient effector T cells can leave the brain vascular system during inflammation when the endothelium expresses ICAM-1 and VCAM-1 at high levels (Moretti et al., 2013). Similarly, kindlin-3-deficient effector T cells cannot extravasate from inflamed dermal microvessels but are able to leave postcapillary venules of skin-draining inflamed lymph nodes, probably because the high expression of integrin ligands and P-selectin stabilizes the weak adhesions of kindlin-3-deficient T cells (Cohen et al., 2013). However, expression of the integrin ligands ICAM-1/2 and VCAM-1 in pharyngeal vessels is lower than in intrathymic vessels thereby excluding this possibility. Therefore, we reasoned that another mechanism must be responsible for the homing ability of kindlin-3-deficient T cell progenitors to the early thymus.
Beside its role in triggering integrin activation in association with talin-1 (Ma et al., 2008; Montanez et al., 2008; Moser et al., 2009a; Moser et al., 2009b; Moser et al., 2008), kindlin-3 is important in stabilizing integrin-ligand interactions by promoting integrin clustering (Feng et al., 2012; Ye et al., 2013). Thus, the gradual T cell progenitor homing defect observed in kindlin-3-deficient mice might be due to instable T cell progenitor/endothelial adhesions, which are unable to withstand increasing shear forces elicited by the maturing blood circulation. Using an intravital microscopy approach we indeed measured an increase in systemic blood flow velocities and shear rates leading to higher hemodynamic forces T-cell progenitors are exposed to during development. In addition, our flow chamber experiments with FL-derived T cell progenitors confirmed this as kindlin-3-deficient cells could adhere to VCAM-1 coated surfaces at low shear stress levels but readily detached at higher shear stress levels when compared to control cells. Moreover, T cells expressing very low kindin-3 levels predominantly adhere to LN vessel segments with lower shear rates than WT T cells. Thus, low shear force conditions may allow adhesion of kindlin-3-deficient T cell progenitors to fetal pharyngeal microvessels, hence enabling their extravasation. However with increasing shear forces at later time points during fetal life, T cell progenitors lacking kindlin-3 and therefore the ability to strengthening integrin-mediated adhesion are not able to efficiently adhere to and migrate out of microvessels into the thymus. The ability to resist low shear forces is also supported by an early report that found high expression of α4β1 on the surface of FL-derived T cell progenitors (Kawakami et al., 1999). In addition, we and others have shown that α4β1-mediated adhesiveness to VCAM-1 is less affected by loss of kindlin-3 in contrast to αLβ2 integrin-mediated adhesion to ICAM-1 (Klapproth et al., 2015; Manevich-Mendelson et al., 2009; Moretti et al., 2013). In this respect, it is interesting to note that lack of β1 integrin expression on hematopoietic stem cells (HSC) abolishes their extravasation and homing to the BM (Potocnik et al., 2000), whereas kindlin-3-deficient HSCs revealed significantly diminished but not absent homing to the BM (Ruppert et al., 2015).
Taken together, our work demonstrates that kindlin-3 is dispensable for integrin-mediated T cell adhesion and signalling at low and indispensable at high vascular shear forces. Furthermore, we also show a general, crucial role of integrins during T cell development and suggest an additional not yet investigated T cell phenotype in LADIII patients.
Materials and methods
Reagent type (species) or resource | Designation | Source or reference | Identifiers | Additional information |
---|---|---|---|---|
Genetic reagent (M.musculus) | Fermt3-/- | PMID: 18278053 | RRID: MGI:2147790 | Dr. Reinhard Fässler (Max Planck Institute of Biochemistry) |
Genetic reagent (M.musculus) | Fermt3flox | PMID: 24089451 | RRID: MGI:5551978 | Dr. Reinhard Fässler (Max Planck Institute of Biochemistry) |
Genetic reagent (M.musculus) | Fermt3n/- | PMID: 26438512 | RRID: MGI:3785479 | Dr. Reinhard Fässler (Max Planck Institute of Biochemistry) |
Genetic reagent (M.musculus) | Rag2-/- | PMID: 1547487 | RRID: MGI:1858556 | Dr. Frederick W Alt (Howard Hughes Medical Institute) |
Genetic reagent (M.musculus) | Mx1-Cre | PMID: 7660125 | RRID: MGI:2176073 | Dr. Klaus Rajewsky (Max Delbrück Center for Molecular Medicine) |
Genetic reagent (M.musculus) | CD4-Cre | PMID: 11728338 | RRID: MGI: 2386448 | Dr. Christopher B. Wilson, (University of Washington) |
Genetic reagent (M.musculus) | 2D2 | PMID: 12732654 | RRID: MGI:3700794 | Dr. Vijay K Kuchroo (Center for Neurologic Diseases) |
Genetic reagent (M.musculus) | mTmG | PMID: 17868096 | RRID: MGI:3716464 | Dr. Liqun Luo (Howard Hughes Medical Institute) |
Antibody | anti-B220-biotin | BD Pharmingen | Cat. #: 553085; RRID: AB_394615 | FACS (1:200) |
Antibody | POD-coupled anti-BrdU | Roche | Cat. #: 11 585 860 001; RRID: AB_514485 | IHC (1:30) |
Antibody | rabbit anti-mouse cleaved caspase-3 | Cell Signalling | Cat. #: 9661; RRID: AB_2341188 | IHC (1:100) |
Antibody | anti-CD3e | eBioscience | Cat. #: 14-0031-82; RRID: AB_467049 | 10 µg/ml |
Antibody | anti-CD3e-biotin | BD Pharmingen | Cat. #: 553060; RRID: AB_394593 | FACS (1:200) |
Antibody | anti-CD3-PE | BD Pharmingen | Cat. #: 555275; RRID: AB_395699 | FACS (1:200) |
Antibody | anti-CD4-biotin | BD Pharmingen | Cat. #: 553649; RRID: AB_394969 | FACS (1:200) |
Antibody | anti-CD4-FITC | BD Pharmingen | Cat. #: 553651; RRID: AB_394971 | FACS (1:200) |
Antibody | anti-CD4-PerCP | BD Pharmingen | Cat. #: 550954; RRID: AB_393977 | FACS (1:200) |
Antibody | anti-CD5-PE | eBioscience | Cat. #: 12-0051-82; RRID: AB_465523 | FACS (1:200) |
Antibody | anti-CD8α-biotin | BD Pharmingen | Cat. #: 553028; RRID: AB_394566 | FACS (1:200) |
Antibody | anti-CD8-APC | eBioscience | Cat. #: 17-0081-81; RRID: AB_469334 | FACS (1:200) |
Antibody | anti-CD8-PE | eBioscience | Cat. #: 12-0081-81; RRID: AB_465529 | FACS (1:200) |
Antibody | anti-CD11b-biotin | BD Pharmingen | Cat. #: 557395; RRID: AB_2296385 | FACS (1:200) |
Antibody | anti-CD11c-biotin | BD Pharmingen | Cat. #: 553800; RRID: AB_395059 | FACS (1:200) |
Antibody | anti-CD16/CD32 | BD Pharmingen | Cat. #: 553142; RRID:AB_394657 | FACS (1:400) |
Antibody | anti-CD19-biotin | eBioscience | Cat. #: 13-0193-82; RRID: AB_657656 | FACS (1:200) |
Antibody | anti-CD24-PE | BD Pharmingen | Cat. #: 553262; RRID: AB_394741 | FACS (1:200) |
Antibody | anti-CD25-FITC | BD Pharmingen | Cat. #: 553071; RRID: AB_394603 | FACS (1:200) |
Antibody | anti-CD28 | eBioscience | Cat. #: 14-0281-82; RRID: AB_467190 | 2 µg/ml |
Antibody | rat anti-CD31 (PECAM-1) | BD Pharmingen | Cat. #: 557355; RRID: AB_396660 | IHC (1:300) |
Antibody | anti-CD31- Alexa Fluor 647 | Biolegend | Cat. #: 102415; RRID: AB_493411 | IHC (1:200) |
Antibody | anti-CD31-eFLour450 | eBioscience | Cat. #: 48-0311-82; RRID: AB_10598807 | FACS (1:200) |
Antibody | anti-CD44-PE | BD Pharmingen | Cat. #: 553134; RRID: AB_394649 | FACS (1:200) |
Antibody | anti-CD45-FITC | eBioscience | Cat. #: 14-0451-82; RRID: AB_467251 | IHC (1:100) |
Antibody | anti-CD45.2-biotin | BD Pharmingen | Cat. #: 553771; RRID: AB_395040 | FACS (1:200) |
Antibody | anti-CD45.2-APC | eBioscience | Cat. #: 17-0454-82; RRID: AB_469400 | FACS (1:200) |
Antibody | hamster anti- CD54 (ICAM) | BD Pharmingen | Cat. #: 553250; RRID: AB_394732 | IHC (1:100) |
Antibody | goat anti- CD106 (VCAM) | R and D Systems | Cat. #: AF643; RRID: AB_355499 | IHC (1:80) |
Antibody | anti-c-kit-APC | eBioscience | Cat. #: 17-1171-81; RRID: AB_469429 | FACS (1:200) |
Antibody | anti-c-kit-PerCP | Biolegend | Cat. #: 105821; RRID: AB_893230 | FACS (1:200) |
Antibody | mouse anti-pan -cytokeratin | Sigma-Aldrich | Cat. #: C2562; RRID: AB_476839 | IHC (1:400) |
Antibody | anti-EpCAM-APC -eFlour780 | Thermo Fisher Scientific | Cat. #: 47-5791-82; RRID: AB_2573986 | FACS (1:200) |
Antibody | rat anti-ER-TR4 | Eric Vroegindeweij (Princess Maxima center of pediatric oncology) | IHC undiluted | |
Antibody | rat anti-ER-TR5 | Eric Vroegindeweij (Princess Maxima center of pediatric oncology) | IHC undiluted | |
Antibody | anti-F4/80-biotin | Bio-Rad | Cat. #: MCA497GA; RRID: AB_323806 | FACS (1:200) |
Antibody | alexa-fluor-488- conjugated anti- Fluorescin IgG | Thermo Fisher Scientific | Cat. #: A-11090; RRID: AB_221562 | IHC (1:500) |
Antibody | rat anti-ICAM-1 PE | Biolegend | Cat. #:116107; RRID: AB_313698 | FACS (1:200) |
Antibody | rabbit anti-Fibronectin | Merck Millipore | Cat. #: AB2033; RRID: AB_2105702 | IHC (1:300) |
Antibody | mouse anti-GAPDH | Merck Millipore | Cat. #: CB1001; RRID: AB_2107426 | WB (1:20.000) |
Antibody | anti-Gr-1-biotin | BD Pharmingen | Cat. #: 553125; RRID: AB_394641 | FACS (1:200) |
Antibody | alexa-fluor-546 -conjugated anti-goat IgG | Thermo Fisher Scientific | Cat: #: A-11056; RRID: AB_2534103 | IHC (1:300) |
Antibody | alexa-fluor-546- conjugated anti-hamster IgG | Thermo Fisher Scientific | Cat: #: A-21111; RRID: AB_2535760 | IHC (1:300) |
Antibody | alexa-fluor-546- conjugated anti-mouse IgG | Thermo Fisher Scientific | Cat: #: A-11003; RRID: AB_2534071 | IHC (1:300) |
Antibody | alexa-fluor-647 -conjugated anti-mouse IgG | Thermo Fisher Scientific | Cat: #: A-31571; RRID: AB_162542 | IHC (1:300) |
Antibody | alexa-fluor-647 -conjugated anti-rabbit IgG | Thermo Fisher Scientific | Cat: #: A-21244; RRID: AB_2535812 | IHC (1:300) |
Antibody | alexa-fluor-488- conjugated anti-rat IgG | Thermo Fisher Scientific | Cat: #: A-11006; RRID: AB_2534074 | IHC (1:300) |
Antibody | alexa-fluor-647- conjugated anti-rat IgG | Thermo Fisher Scientific | Cat: #: A-21247; RRID: AB_141778 | IHC (1:300) |
Antibody | anti-Integrin α4-PE | BD Pharmingen | Cat. #: 553157; RRID: AB_394670 | FACS (1:200) |
Antibody | anti-Integrin αL-PE | BD Pharmingen | Cat. #: 553121; RRID: AB_394637 | FACS (1:200) |
Antibody | anti-Integrin β1-PE | Biolegend | Cat. #: 102207; RRID: AB_312884 | FACS (1:200) |
Antibody | anti-Integrin β2-PE | BD Pharmingen | Cat. #: 553293; RRID: AB_394762 | FACS (1:200) |
Antibody | anti-Integrin β3-PE | eBioscience | Cat. #: 12-0611-81; RRID: AB_465717 | FACS (1:200) |
Antibody | anti-Integrin β7-PE | BD Pharmingen | Cat. #: 557498; RRID: AB_396735 | FACS (1:200) |
Antibody | rabbit anti-Kindlin-1 | Markus Moser (MPI of Biochemistry) | WB (1:1000) | |
Antibody | mouse anti-Kindlin-2 | Sigma-Aldrich | Cat. # SAB4200525 -200UL | WB (1:1000) |
Antibody | rabbit anti-Kindlin-3 | Markus Moser (MPI of Biochemistry) | WB (1:3000) | |
Antibody | rabbit anti-pan-laminin | Rupert Timpl | IHC (1:700) | |
Antibody | anti-LFA-1-PE | BD Pharmingen | Cat. #: 553121; RRID: AB_394637 | IF (1:100) |
Antibody | anti-NK1.1-biotin | BD Pharmingen | Cat. #: 553163; RRID: AB_394675 | FACS (1:200) |
Antibody | anti-PIR-APC | eBioscience | Cat. #: 17-3101-82; RRID: AB_1944406 | FACS (1:200) |
Antibody | anti-PIR-PE | BD Pharmingen | Cat. #: 550349; RRID: AB_393628 | FACS (1:200) |
Antibody | anti-p-Tyrosine | Santa Cruz Biotechnology | Cat. #: sc-7020; RRID: AB_628123 | IF (1:50) |
Antibody | anti-Sca-1-PE | eBioscience | Cat. #: 12-5981-82; RRID: AB_466086 | FACS (1:200) |
Antibody | mouse anti-talin | Sigma-Aldrich | Cat. #: T3287; RRID: AB_477572 | WB (1:20.000) |
Antibody | anti-TCRβ-PE | eBioscience | Cat. #: 12-5961-82; RRID: AB_466066 | FACS (1:200) |
Antibody | anti-TCRγδ-biotin | BD Pharmingen | Cat. #: 553176; RRID: AB_394687 | FACS (1:200) |
Antibody | anti-Ter119-biotin | BD Pharmingen | Cat. #: 553672; RRID: AB_394985 | FACS (1:200) |
Antibody | anti-Thy1.2-biotin | BD Pharmingen | Cat. #: 553001; RRID: AB_394540 | FACS (1:200) |
Antibody | rat anti-VCAM-1 PE | Biolegend | Cat. #: 105713; RRID: AB_1134166 | FACS (1:200) |
Peptide, recombinant protein | recombinant mouse CCL21 | R and D Systems | Cat. #: 457–6C; Accession #: P84444.1 | |
Peptide, recombinant protein | recombinant mouse CCL25 | R and D Systems | Cat. #: 481-TK; Accession #: O35903.1 | |
Peptide, recombinant protein | recombinant mouse ICAM-1-FC | R and D Systems | Cat. #: 796-IC; Accession #: Q3U8M7 | |
Peptide, recombinant protein | MOG35-55 | MPI of Biochemistry, Core facility | ||
Peptide, recombinant protein | recombinant mouse P-Selectin-FC | R and D Systems | Cat. #: 737-PS; Accession #: Q01102 | |
Peptide, recombinant protein | recombinant mouse VCAM-1-FC | R and D Systems | Cat. #: 643-VM; Accession #: CAA47989 | |
Commercial assay or kit | PE Annexin V Apoptosis Detection Kit | BD Biosciences | Cat. #: 559763 | |
Commercial assay or kit | BrdU Flow Kit | BD Pharmingen | Cat. #: 559619; RRID: AB_2617060 | |
Commercial assay or kit | anti-biotin MicroBeads | Miltenyi | Cat. #: 130-090-485; RRID: AB_244365 | |
Commercial assay or kit | CD4 T cell isolation kit | Miltenyi | Cat. #: 130-104-454 | |
Commercial assay or kit | Vectastain Elite ABC HRP Kit, rabbit IgG | VECTOR laboratories | Cat. #: PK-6101; RRID: AB_2336820 | |
Chemical compound, drug | CellTrace CFSE Cell Proliferation Kit | Thermo Fisher Scientific | Cat. #: C34554 | |
Chemical compound, drug | Collagenase D | Sigma-Aldrich | Cat. #: C0130 | 1 mg/ml |
Chemical compound, drug | FITC-Dextran | Sigma-Aldrich | Cat. #: FD150S | |
Chemical compound, drug | DNaseI | Sigma-Aldrich | Cat. #: D5025 | 100 U/ml |
Chemical compound, drug | dGuo | Sigma-Aldrich | Cat. #: D0901 | 1.35 mM |
Chemical compound, drug | CellTrace Far Red Cell Proliferation Kit | Thermo Fisher Scientific | Cat. #: C34564 | 1 µM |
Chemical compound, drug | FluoSpheres 580/606 | Thermo Fisher Scientific | Cat. #: F8821 | 1 µM |
Chemical compound, drug | LPS | Sigma-Aldrich | Cat. #: L2654 | 1 mg/ml |
Chemical compound, drug | poly-L-Lysine | Sigma-Aldrich | Cat. #: P8920 | pure |
Chemical compound, drug | Phalloidin Alexa350 | Thermo Fisher Scientific | Cat. #: A22281 | IF (1:40) |
Chemical compound, drug | PureCol | Advanced BioMatrix | Cat. #: 5005–100 ML | |
Chemical compound, drug | Streptavidin Cy3 | Jackson Immuno Research | Cat. #: 016-160-084; RRID: AB_2337244 | FACS (1:400) |
Chemical compound, drug | Streptavidin FITC | Thermo Fisher Scientific | Cat. #: SA10002 | FACS (1:200) |
Chemical compound, drug | Streptavidin PerCP-Cy5.5 | BD Pharmingen | Cat. #: 551419 | FACS (1:200) |
Software, algorithm | ImageJ | National Institute of Health | RRID:SCR_003070 | |
Software, algorithm | ImSpector Pro | LaVision BioTec GmbH | ||
Software, algorithm | Photoshop | Adobe | RRID:SCR_014199 | |
Software, algorithm | Slidebook 6.0.8 Software | Intelligant Imaging Innovations | RRID:SCR_014300 | |
Software, algorithm | VirtualDub | GNU General Public License (GPL) | ||
Other | Glass Bottom Microwell Dishes | MatTek Corporation | Cat. #: P35G-1.5–20 C | |
Other | Ibidi flow chambers µ-Slide I 0.8 Luer IbiTreat | Ibidi | Cat. #: 80196 |
Mice and chimeras
Request a detailed protocolFermt3-/- mice have been described previously (Moser et al., 2008). All mice were from a mixed 129/SvJ x C57BL/6 background. FL cell chimeras were generated as previously described (Prümmer and Fliedner, 1986; Tulunay et al., 1975). Briefly, 5–6 × 106 cells from the FL of E14.5 embryos were injected into the tail vain of sub-lethally irradiated (1 × 6 Gy) Rag2-/- mice (Shinkai et al., 1992) and chimeras were analyzed 8 to 10 weeks later. Mixed FL cell chimeras were generated by injection of 5 × 106 E14.5 FL cells from WT or Fermt3-/- embryos, respectively, which were mixed with E14.5 FL cells from congenic B6.SJL WT embryos in a 9:1 ratio, into lethally irradiated C57BL/6 mice (1 × 7 Gy and 1 × 6 Gy with 4 hr in between). Conditional Fermt3 deletion was obtained upon two intraperitoneal injections of pI-pC (500 μg/mouse) (GE Healthcare) into Fermt3fl/fl/Mx1-Cre mice. Pictures of dissected thymi were taken with a ProgRes C14 camera (JenOptic) connected to a Leica MZ FLIII stereomicroscope. All animals were mated and maintained under specific pathogen-free conditions at the animal facility of the Max Planck Institute of Biochemistry. All mouse experiments were performed with the approval of the District Government of Bavaria.
Western blot analysis
Request a detailed protocolProtein lysates were separated on a 10% SDS-PAGE and analyzed by Western blotting using polyclonal rabbit anti-mouse kindlin-1 and kindlin-3 antisera (Ussar et al., 2008; Ussar et al., 2006), monoclonal mouse anti-kindlin-2 (Sigma), monoclonal mouse anti-talin (Sigma) and mouse anti-GAPDH monoclonal antibody (Calbiochem) with a LAS-4000 imager (Fujifilm).
Multi-color flow cytometry analysis and cell sorting
Request a detailed protocolMulti-color flow-cytometric analysis and cell sorting were performed using FACS-Calibur and FACS-Aria flow cytometers (BD Biosciences), respectively. Data for viable cells, which were determined by forward light scatter and propidium iodide exclusion, were obtained with BD CellQuest Pro software. All antibodies we used were purchased from eBioscience or BD Pharmingen. Lineage negative (Linneg) cell populations were obtained by removal of mature cells using the following cocktails of biotinylated antibodies: anti-B220, anti-CD19, anti-TER119, anti-NK1.1, anti-CD11b (Mac-1), anti-Gr-1, anti-CD8α, anti-CD3e, anti-TCRβ, anti-TCRγδ and anti-CD11c for fetal and adult thymi; anti-TER119, anti-B220, anti-CD11b, anti-Gr-1, anti-CD11c, anti-NK1.1, anti-CD4 and-CD8α for postnatal peripheral blood; anti-B220, anti-CD19, anti-TER119, anti-NK1.1, anti-Gr-1 and anti-Thy1.2 for FL and fetal blood (FB). Cells were then stained either with PerCP-Cy5.5-conjugated or FITC-coniugated Streptavidin and excluded by FL3 or FL1 FACS channels. The resulting Linneg cell populations were further gated with anti-CD25-FITC, anti-CD44-PE and anti-c-kit-APC for thymocytes; with anti-Sca-1-PE and anti-c-kit-APC for postnatal peripheral blood cells; with anti-CD45-FITC, anti-PIR-PE and anti-c-kit-APC or anti-c-kit-PerCP, anti-PIR-APC and anti-integrin-PE (α4, αL, β1, β2, β3, β7) for FL cells; with anti-PIR-PE and anti-c-kit-APC for FB cells.
Mature T cells were analyzed for surface receptor expression with anti-CD4-FITC, anti CD8-APC, anti-CD3-PE, anti-CD5-PE, anti-CD24-PE and TCRβ-PE.
FL-Linneg cells used for the time-lapse visualization of thymus attraction (see below) were obtained by negative selection through MACS separation columns with anti-Biotin-coupled magnetic beads according to the manufacturer’s instructions (MACS Miltenyi Biotec).
Expression of kindlin-3 in different thymic cell types was analysed by flow cytometry with thymi from Kindlin-3-EGFP knockin mice. A characterization of this mouse strain expressing a kindlin-3-EGFP fusion protein will be described elsewhere. In order to analyse kindlin-3 expression in leukocytes, thymic epithelial cells and endothelial cells, an adult thymus or a pool of 5 E15.5 fetal thymi were squeezed between two glass slides to remove the majority of T cells. The remaining tissue was digested in 1 mg/ml Collagenase D and 100 U/ml DNase I (both from Sigma) in 3% FCS/PBS for 20 to 30 min at 37°C, followed by a Fc-receptor block with anti-CD16/CD32 antibody and incubation with anti-CD31 eFlour450, anti-EpCAM APC eFlour780 (both Invitrogen), anti-CD45.2 APC and anti-CD3 PE antibodies.
Immunofluorescence analysis of embryos and thymi
Request a detailed protocol4% PFA-prefixed, frozen embryos and thymi embedded in Shandon Cryomatrix compound (Thermo Scientific) were first sliced into 8–10 μm thick sections and stained with the following antibodies for the embryos: rabbit anti-fibronectin polyclonal antibody (Chemicon), mouse anti-pan-cytokeratin monoclonal antibody (Sigma), rat anti-CD45-FITC (eBioscience), goat anti-CD106 (VCAM-1, R & D Systems), hamster anti-CD54 (ICAM-1, Pharmingen) and rat anti-CD31 (PECAM-1, Pharmingen) followed by Alexa-Fluor-647-conjugated anti-rabbit IgG, Alexa-Fluor-546-conjugated anti-mouse IgG, Alexa-Fluor-488-conjugated anti-rat IgG, Alexa-Fluor-647-conjugated anti-mouse IgG, Alexa-Fluor-546-conjugated anti-goat IgG, and Fluor-546-conjugated anti-hamster IgG antibodies (Invitrogen); for the thymus: rabbit anti-pan-laminin polyclonal antibody (gift from Rupert Timpl), mouse anti-pan-cytokeratin monoclonal antibody (Sigma), rat anti-CD31 (PECAM-1, Pharmingen), rat anti-CD4-FITC (eBioscience), rat anti-CD8-Biotin (eBioscience), anti-CD106 (VCAM-1, R & D Systems), hamster anti-CD54 (ICAM-1, Pharmingen), rat anti-ER-TR4 and anti-ER-TR5 polyclonal antibodies (kindly provided by Eric Vroegindeweij) followed by Alexa-Fluor-647-conjugated anti-rabbit IgG, Alexa-Fluor-647-conjugated anti-rat IgG, Alexa-Fluor-488-conjugated anti-Fluorescein/Oregon green IgG, Cy3-coniugated Streptavidin (Jackson), Alexa-Fluor-488-conjugated anti-rat IgG, Fluor-546-conjugated anti-goat IgG, and Fluor-546-conjugated anti-hamster IgG antibodies (Invitrogen). Thymic sections were fixed with acetone instead of PFA for anti-ER-TR4 and -TR5 staining.
Relative quantification of ICAM-1 and VCAM-1 expression was performed with directly labelled antibodies: rat anti-ICAM-1-PE, rat anti-VCAM-1-PE and the respective isotypes, rat anti-CD31-Alexa-Fluor 647 (all from Biolegend).
The stained sections were mounted with a fluorescence mounting medium (Elvanol) and images were acquired with a fluorescence microscope (Imager.Z1, Zeiss) and analyzed by the Axio Vision 40 (version 4.8.2.0) software (Zeiss), utilizing 10x, 20x and 40x magnifications.
Immunohistologic analysis of the thymus
Request a detailed protocol4% PFA fixed and paraffin-embedded thymi were sliced into 8 μm tick sections and stained with antibodies. The sections were counterstained with Mayer’s haematoxylin (Merck) and mounted in Entellan (Merck). Haematoxylin and Eosin (H/E) staining was performed according to standard protocol. Images were acquired with the light microscope Axioskop (Zeiss) and analyzed with the Adobe Photoshop software.
Apoptosis assays
Request a detailed protocolParaffin-embedded thymus sections were stained with rabbit anti-mouse cleaved caspase-3 antibody (Cell Signalling). Apoptotic cells were detected by DAB staining after treatment with the immunoperoxidase Vectastain ABC system (Vector Laboratories).
Thymocytes from P3 mice were stained with PE-labeled Annexin V and 7-AAD according to the manufacturer’s instructions (BD Pharmingen Apoptosis Detection Kit). T cells were additionally stained for anti-CD4-FITC and anti-CD8-APC and analysed by FACS.
Immune synapse analysis
Request a detailed protocolCD4 T cells were purified from spleen by negative selection using biotinylated anti-CD8, anti-B220, anti-Gr-1, anti-F4/80 and anti-Ter119 antibodies and anti-biotin microbeads following the manufacturer´s instructions (MACS Miltenyi Biotec). Bone marrow derived, LPS matured dendritic cells were loaded with 1 µg/ml MOG35-55 peptide for 2 hr at a concentration of 106 cell/ml. 1 × 105 loaded DCs were mixed with 5 × 105 CD4 T cells in 200 µl R10 medium and seeded on poly-L-Lysine coated glass-bottom dishes (Mattek). Cells were fixed after 30 min by adding 200 µl warm 6% PFA in R10 medium for 20 min at 37°C. After permeabilization with 0.2% Triton X-100 in PBS for 15 min and blocking with 1% BSA for 1 h cells were stained with antibodies against LFA-1 (clone 2D7, BD Biosciences) and p-Tyrosine (PY199, Santa Cruz Biotechnology). Phalloidin Alexa350 was used to visualize actin. Cells were imaged with a Leica TCS SP5 X confocal microscope (Leica Microsystems) equipped with a 63 × NA 1.40 oil objective and Leica Confocal Software (LAS AF). Single channels were imaged sequentially. All pictures were processed with Photoshop (Adobe Systems, San José, California, USA).
Proliferation assays
Request a detailed protocolFor in vivo labelling of thymocytes, bromodeoxyuridine (BrdU; 50 μg/gram body weight) was intraperitoneally injected into P3 mice. One hour after injection, cells were isolated from thymi and stained with FITC-labeled anti-BrdU monoclonal antibody (BD Pharmingen BrdU Flow Kit), anti-CD4-PerCP and anti-CD8-PE antibodies, and analyzed by flow cytometry. Alternatively, paraffin-embedded thymus sections were DAB stained after incubation with a POD-coupled anti-BrdU antibody (Roche).
For the in vitro labeling of control (WT/2D2) and kindlin-3-deficient (Fermt3-/-/CD4-Cre/2D2) CD4+ T cells (Moretti et al., 2013), CellTrace CFSE Cell Proliferation Kit (Thermo Fisher) was used to trace dividing cells. Briefly, single cell suspensions from spleens were treated with ammonium-chloridepotassium for RBC lysis and CD4 T cells were sorted using CD4 T cell isolation kit (Milteniy Biotech). T cells were then stained with CFSE according to the manufacturer’s instructions and about 5 × 104 T cells per sample were cocultured in triplicate in 96-well round bottom plate with 2 × 104 DCs and increasing concentration of soluble MOG35-55 peptide (0.1, 1 and 10 μg/mL). For T cell stimulation with anti-CD3e and anti-CD28 Abs, 2 × 105 CFSE-labelled cells per sample were cultured in wells pre-coated for 1 hr at 37°C with anti-CD3e (10 µg/ml, eBioscience), with soluble anti-CD28 (2 µg/ml, eBioscience) and PMA (20 ng/ml). Proliferation was analysed by FACS after 3 days of incubation in R10 (RPMI-1640, 10% FCS, 1x Penicillin-Streptomycin) at 37°C.
Time-lapse visualization of thymus attraction
Request a detailed protocolTime-lapse imaging of horizontal cell migration to fetal thymus lobes was recorded as previously described (Liu et al., 2005; Ueno et al., 2005). Briefly, 10 µL of freshly neutralized collagen solution (1.72 mg/mL PureCol, Advanced BioMatrix, in RPMI 1640-based culture medium) containing 1–2 × 105 FL-Linneg cells from either Fermt3+/+ or Fermt3-/- C57BL/6 (CD45.2+) animals was placed in a 35 mm plastic dish and solidified at 37°C for 10 min. An E15.5 fetal thymus lobe from wild-type SJL (CD45.1+) mouse, pre-treated for 6 days with 1.35 mM 2-deoxyguanosine (dGuo; Sigma) was positioned approximately 0.5 mm away from the cell spot. The culture was submerged in 1.72 mg/mL collagen medium and solidified at 37°C for 30 min. The dish was placed in 5% CO2 under an Axiovert 40 CFL microscope (Zeiss) equipped with a digital CCD camera. The culture was time-lapse monitored for 36 to 48 hr. After colonization, fetal thymus lobes were removed from the collagen gel, rinsed, and further cultured for 18 days under conventional organ culture conditions (see below). Thymi were than pooled, squeezed through a cell strainer and the resulting single cell suspensions were stained with anti-CD45.2 to identify FL cell-derived thymocytes and further gated for anti-CD4-FITC and anti-CD8-APC.
Fetal Thymus Organ Culture
Request a detailed protocolSeeded thymus lobes were cultured on 0.8 μm isopore membrane filters (Millipore) supported by a Gelfoam sponge (Pfizer) at an interface between 5% CO2-humidified air and RPMI 1640-based culture medium containing 10% FCS, 100 U/ml penicillin and 100 μg/ml streptomycin (PAA), 2 mM L-glutamine (PAA), 1X non-essential amino acids (Gibco), 1 mM sodium pyruvate (Gibco), 10 mM HEPES, 50 μM 2-mercaptoethanol (Sigma). Details have been described (Ueno et al., 2005).
Blood flow velocity measurement
Request a detailed protocolBlood flow velocity measurements were performed in the fetal yolk-sac vasculature of E12.5 to E16.5 fetuses as described (Margraf et al., 2017). Briefly, pregnant WT mice were anesthetised and fetuses surrounded by the yolk sac and still connected to the placenta were surgically exteriorized and placed on a modified intravital microscopy stage. Manually prepared glass microcapillaries (Clark Capillaries GCI150TF-10, Clark Electromedical Instruments, Pangbourne Reading) were used to inject 4 µl of a 5% FITC dextrane solution (FD150S, Sigma-Aldrich, Taufkirchen, Germany) with 1 µl of red fluorescent microspheres (FluoSpheres polystyrene microspheres 1,0 µm red fluorescent 580/605; Invitrogen) into the fetal vasculature. Imaging was performed using an in vivo microscope setup (Olympus BX1, Olympus, Hamburg, Germany) equipped with appropriate filter sets and a double-flash device (Rapp Optoelectronics, Hamburg, Germany). Images were recorded using a 60x objective (Olympus LUM Plan FI/IR 60x/0.90W) with setup specific CCD-cameras (Kappa CF5 HS; Kappa Optronics GmbH, Gleichen, Germany, and LaVision Imager ProX pco.1600L, LaVision GmbH, Goettingen, Germany) and recorded utilizing VirtualDub (www.virtualdub.org) and Imspector Pro Software (Lavision BioTec GmbH, Bielefeld, Germany). Analysis was performed using ImageJ (National Institute of Health). Vessel diameters were chosen between 20 and 50 µm.
Flow chamber assays
Request a detailed protocolFlow chamber assays were performed with the ibidi pump system (Martinsried, Germany). Ibidi flow chambers (µ-Slide I 0.8 Luer ibiTreat) were coated with 10 µg/ml recombinant mouse (rm) ICAM-1-Fc or rmVCAM-1-Fc, 10 µg/ml rmP-selectin-Fc, 5 µg/ml rmCCL21 and 5 µg/ml rmCCL25 (all from R & D systems) over night at 4°C. After blocking for 1 hr with 1% casein (Thermo Fisher Scientific) at room temperature, the channels were filled with control or Fermt3-/- Pir+ T cell progenitors sorted from E13.5 fetal livers. After 3 min incubation without flow, slow flow of approximately 0.15 dyn/cm2 was applied for 3 min followed by increasing flow of 1 and 3 dyn/cm2 for 10 min each. Pictures were taken every 5 s for a total of 25 min at an Axiovert 40 CFL microscope (Zeiss) equipped with a digital CCD camera and a 10 x objective. The number of cells that were adherent immediately before the start of the fast flow was set to 100%. Cells that did not change position within 10 s were considered to be firmly adherent.
Live cell imaging of T cell adhesion within the lymph node vasculature and flow in vivo
Request a detailed protocolCD4 T cells were isolated from spleen by negative selection as described above. Anaesthetized mice were injected intravenously with a 1:1 mixture of CFSE and Far Red labelled WT and Fermt3 hypomorphic CD4 T cells. The hair of the hind legs was removed and mice were placed into an imaging chamber with integrated heating plate. In a dorsal approach the skin of the hind legs was cut and the popliteal lymph nodes were exposed. To reveal the superficial vessels of the lymph nodes, they were fixed to the biceps femoris muscle using Histoacryl tissue adhesive (B. Braun). PBS-soaked batting was placed around the legs to keep the tissue moist. The lymph nodes were covered with a cover glass, which was topped with PBS to facilitate imaging. Time-lapse video microscopy was performed using an upright spinning disk confocal microscope (Examiner, Zeiss, Germany) equipped with a confocal scanner unit CSU-X1 (Yokogawa Electric Corporation, Japan), an EMCCD camera (Evolve, Photometrics, USA) and a 20x/1.0NA water immersion objective (Zeiss, Germany). 4D images (30 z-stacks with a step size of 4 µm and an average time lapse interval of 42 s) were acquired by using two lasers with an excitation wavelength of 488 nm and 640 nm. Adhesion was analyzed by generating sum projections of z-stacks over time using Slidebook 6.0.8 Software (3i, USA). The number of adherent leukocytes was determined as cells attached to the same position for more than 1 min using Fiji/ImageJ software (NIH, USA). Centerline blood flow velocities in the same vessels were obtained upon subsequent intravenous injection of 107 fluorescent microspheres (1 µm in diameter; FluoSpheres 580/605, Invitrogen) by measuring frame‐to-frame displacement of single beads in LN microvessel segments. Images of one plane with an average time lapse interval of 66.5 ms were acquired for one minute using one laser with an excitation wavelength of 561 nm. Wall shear rates were calculated as previously described (Sperandio et al., 2006).
Statistic analysis
Request a detailed protocolMeans, standard deviations and statistical comparisons with Student’s t-test (*p<0.05, **p<0.01, ***p<0.001) were made using Microsoft Excel software.
Data availability
All data generated or analysed during this study are included in the manuscript and supporting files.
References
-
Myelin oligodendrocyte glycoprotein-specific T cell receptor transgenic mice develop spontaneous autoimmune optic neuritisThe Journal of Experimental Medicine 197:1073–1081.https://doi.org/10.1084/jem.20021603
-
The integrin co-activator Kindlin-3 is expressed and functional in a non-hematopoietic cell, the endothelial cellJournal of Biological Chemistry 285:18640–18649.https://doi.org/10.1074/jbc.M109.085746
-
Thymus-homing precursors and the thymic microenvironmentTrends in Immunology 27:477–484.https://doi.org/10.1016/j.it.2006.08.004
-
T cell development in the thymus: from periodic seeding to constant outputJournal of Theoretical Biology 249:384–394.https://doi.org/10.1016/j.jtbi.2007.07.028
-
Kindlin-3 mediates integrin αLβ2 outside-in signaling, and it interacts with scaffold protein receptor for activated-C kinase 1 (RACK1)Journal of Biological Chemistry 287:10714–10726.https://doi.org/10.1074/jbc.M111.299594
-
The importation of hematogenous precursors by the thymus is a gated phenomenon in normal adult miceThe Journal of Experimental Medicine 193:365–374.https://doi.org/10.1084/jem.193.3.365
-
Two distinct steps of immigration of hematopoietic progenitors into the early thymus anlageInternational Immunology 13:1203–1211.https://doi.org/10.1093/intimm/13.9.1203
-
Cell kinetics in the fetal mouse thymus: precursor cell input, proliferation, and emigrationJournal of Immunology 138:1026–1030.
-
LFA-1 on CD4+ T cells is required for optimal antigen-dependent activation in vivoThe Journal of Immunology 173:4443–4451.https://doi.org/10.4049/jimmunol.173.7.4443
-
Roles of integrins and CD44 on the adhesion and migration of fetal liver cells to the fetal ThymusJournal of Immunology 163:3211–3216.
-
Mechanisms of T cell development and transformationAnnual Review of Cell and Developmental Biology 27:539–562.https://doi.org/10.1146/annurev-cellbio-092910-154008
-
Conditional vascular cell adhesion molecule 1 deletion in mice: impaired lymphocyte migration to bone marrowThe Journal of experimental medicine 193:741–754.
-
Getting to the site of inflammation: the leukocyte adhesion cascade updatedNature Reviews Immunology 7:678–689.https://doi.org/10.1038/nri2156
-
Kindlin-2 (Mig-2): a co-activator of beta3 integrinsThe Journal of Cell Biology 181:439–446.https://doi.org/10.1083/jcb.200710196
-
Maturation of platelet function during murine fetal development in vivoArteriosclerosis, Thrombosis, and Vascular Biology 37:1076–1086.https://doi.org/10.1161/ATVBAHA.116.308464
-
Kindlin-2 controls bidirectional signaling of integrinsGenes & Development 22:1325–1330.https://doi.org/10.1101/gad.469408
-
Kindlin-3 is essential for integrin activation and platelet aggregationNature Medicine 14:325–330.https://doi.org/10.1038/nm1722
-
The fetal liver as an alternative stem cell source for hemolymphopoietic reconstitutionThe International Journal of Cell Cloning 4:237–249.https://doi.org/10.1002/stem.5530040402
-
Kindlin-3-mediated integrin adhesion is dispensable for quiescent but essential for activated hematopoietic stem cellsThe Journal of Experimental Medicine 212:1415–1432.https://doi.org/10.1084/jem.20150269
-
Spontaneous skin ulceration and defective T cell function in CD18 null miceThe Journal of Experimental Medicine 188:119–131.https://doi.org/10.1084/jem.188.1.119
-
Kindlin-3-mediated signaling from multiple integrin classes is required for osteoclast-mediated bone resorptionThe Journal of Cell Biology 192:883–897.https://doi.org/10.1083/jcb.201007141
-
LFA-1-deficient mice show normal CTL responses to virus but fail to reject immunogenic tumorJournal of Experimental Medicine 183:1415–1426.https://doi.org/10.1084/jem.183.4.1415
-
Analysis of leukocyte rolling in vivo and in vitroMethods in enzymology 416:346–371.https://doi.org/10.1016/S0076-6879(06)16023-1
-
Development of T-lymphocytes in mouse fetal Thymus organ cultureMethods in Molecular Biology 290:117–133.
-
The Kindlins: subcellular localization and expression during murine developmentExperimental Cell Research 312:3142–3151.https://doi.org/10.1016/j.yexcr.2006.06.030
-
URP1: a member of a novel family of PH and FERM domain-containing membrane-associated proteins is significantly over-expressed in lung and colon carcinomasBiochimica et Biophysica Acta (BBA) - Molecular Basis of Disease 1637:207–216.https://doi.org/10.1016/S0925-4439(03)00035-8
-
Contact-dependent T cell activation and T cell stopping require talin1The Journal of Immunology 187:6256–6267.https://doi.org/10.4049/jimmunol.1102028
-
Leukocytosis and resistance to septic shock in intercellular adhesion molecule 1-deficient miceJournal of Experimental Medicine 180:95–109.https://doi.org/10.1084/jem.180.1.95
-
The mechanism of kindlin-mediated activation of integrin αIIbβ3Current Biology 23:2288–2295.https://doi.org/10.1016/j.cub.2013.09.050
Article and author information
Author details
Funding
Deutsche Forschungsgemeinschaft (SFB914)
- Markus Moser
- Federico Andrea Moretti
- Sarah Klapproth
- Raphael Ruppert
- Andreas Margraf
- Weber Jasmin
- Robert Pick
- Christoph Scheiermann
- Markus Sperandio
- Reinhard Fässler
Max-Planck-Gesellschaft (Open-access funding)
- Markus Moser
- Reinhard Fässler
The funders had no role in study design, data collection and interpretation, or the decision to submit the work for publication.
Acknowledgements
We thank Arnoud Sonnenberg for critically reading the manuscript and the Fässler lab members for discussion, Michal Grzejszczyk and Kerstin Kraus for excellent technical assistance and Eric Vroegindeweij for providing the anti-ER-TR4 and 5 antibodies. The work was supported by the Deutsche Forschungsgemeinschaft (SFB914; Projects A01, A05, B01, B09 and Z03) and the Max Planck Society.
Ethics
Animal experimentation: Animal experimentation: Housing and use of laboratory animals at the Max Planck Institute of Biochemistry are fully compliant with all German (e.g. German Animal Welfare Act) and EU (e.g. Annex III of Directive 2010/63/EU on the protection of animals used for scientific purposes) applicable laws and regulations concerning care and use of laboratory animals. All of the animals were handled according to approved licenses from the Government of Upper Bavaria (55.2-1-54-2532-96-2015 and 55.2-1-54-2532-175-2011)
Copyright
© 2018, Moretti et al.
This article is distributed under the terms of the Creative Commons Attribution License, which permits unrestricted use and redistribution provided that the original author and source are credited.
Metrics
-
- 1,255
- views
-
- 188
- downloads
-
- 10
- citations
Views, downloads and citations are aggregated across all versions of this paper published by eLife.
Download links
Downloads (link to download the article as PDF)
Open citations (links to open the citations from this article in various online reference manager services)
Cite this article (links to download the citations from this article in formats compatible with various reference manager tools)
Further reading
-
- Developmental Biology
The morphogen FGF8 establishes graded positional cues imparting regional cellular responses via modulation of early target genes. The roles of FGF signaling and its effector genes remain poorly characterized in human experimental models mimicking early fetal telencephalic development. We used hiPSC-derived cerebral organoids as an in vitro platform to investigate the effect of FGF8 signaling on neural identity and differentiation. We found that FGF8 treatment increases cellular heterogeneity, leading to distinct telencephalic and mesencephalic-like domains that co-develop in multi-regional organoids. Within telencephalic regions, FGF8 affects the anteroposterior and dorsoventral identity of neural progenitors and the balance between GABAergic and glutamatergic neurons, thus impacting spontaneous neuronal network activity. Moreover, FGF8 efficiently modulates key regulators responsible for several human neurodevelopmental disorders. Overall, our results show that FGF8 signaling is directly involved in both regional patterning and cellular diversity in human cerebral organoids and in modulating genes associated with normal and pathological neural development.
-
- Developmental Biology
Wnt signaling plays crucial roles in embryonic patterning including the regulation of convergent extension (CE) during gastrulation, the establishment of the dorsal axis, and later, craniofacial morphogenesis. Further, Wnt signaling is a crucial regulator of craniofacial morphogenesis. The adapter proteins Dact1 and Dact2 modulate the Wnt signaling pathway through binding to Disheveled. However, the distinct relative functions of Dact1 and Dact2 during embryogenesis remain unclear. We found that dact1 and dact2 genes have dynamic spatiotemporal expression domains that are reciprocal to one another suggesting distinct functions during zebrafish embryogenesis. Both dact1 and dact2 contribute to axis extension, with compound mutants exhibiting a similar CE defect and craniofacial phenotype to the wnt11f2 mutant. Utilizing single-cell RNAseq and an established noncanonical Wnt pathway mutant with a shortened axis (gpc4), we identified dact1/2-specific roles during early development. Comparative whole transcriptome analysis between wildtype and gpc4 and wildtype and dact1/2 compound mutants revealed a novel role for dact1/2 in regulating the mRNA expression of the classical calpain capn8. Overexpression of capn8 phenocopies dact1/2 craniofacial dysmorphology. These results identify a previously unappreciated role of capn8 and calcium-dependent proteolysis during embryogenesis. Taken together, our findings highlight the distinct and overlapping roles of dact1 and dact2 in embryonic craniofacial development, providing new insights into the multifaceted regulation of Wnt signaling.