Gene Regulation: Acting in tandem
According to the RNA World hypothesis, RNA was the original molecule from which all life has since evolved. Like DNA, RNA can store genetic information and replicate itself. However, it can also perform tasks that DNA leaves to proteins, such as catalyzing chemical reactions. Many of these roles rely on RNA molecules adopting sophisticated tertiary structures which are mostly conserved across the three kingdoms of life. This reflects the ancient origins of these structures, possibly in the RNA World.
In messenger RNA molecules in bacteria, certain sequences upstream of the coding region form structures called riboswitches that can bind various ligands. By stabilizing or altering these structures, the binding of the ligand turns the production of the downstream gene either on or off (hence the name riboswitch). A change in the concentration of a given ligand therefore regulates the production of a given protein via the relevant riboswitch. Most of these ligands are molecules that are essential for the cell to survive and grow, which suggests that riboswitches are ancient structures. Riboswitches are therefore of interest for at least two reasons: their role as metabolic regulators in bacteria and as potential relics of the RNA World.
A large number of putative riboswitches have been identified by analyzing bacterial genome sequences, but many of these have remained as 'orphans' because it has been difficult to identify the molecules they bind to (Weinberg et al., 2010; Weinberg et al., 2017). The large ykkC family of orphans can be subdivided in different classes and subclasses based on the tertiary structure of the riboswitches, which exact molecule they bind to, and which genes they control. Recently, the ligand shared by the most abundant ykkC classes was identified as guanidine. The remaining subclass, called ‘subtype 2’, has been shown to regulate a group of genes that is distinct from those regulated by other members of the ykkC family (Nelson et al., 2017). However, its ligand and exact role were still unclear.
Now, in eLife, Ronald Breaker and colleagues at Yale University – Madeline Sherlock as first author, Narasimhan Sudarsan and Shira Stav – report that there are four distinct classes of subtype 2 riboswitch (called 2a, 2b, 2c and 2d), and that they have identified the ligand that binds to the 2b riboswitch (Sherlock et al., 2018). This ligand is a molecule called PRPP (phosphoribosyl pyrophosphate), which has a central role in purine biosynthesis; in particular, it helps to make the nucleobases adenine and guanine, which are crucial building blocks for DNA and RNA. In a separate yet-to-be-published paper they report that the 2a riboswitch binds to ppGpp: this molecule is an example of an alarmone, a class of small signal molecules that are produced by bacteria when they are under stress (ME Sherlock, N Sudarsan, RR Breaker, In preparation).
Sherlock et al. go on to study the 2b riboswitch in more detail. On its own, this riboswitch appears to respond to high levels of PRPP by turning on genes that are involved in purine biosynthesis. However, the 2b riboswitch mostly works in tandem with another riboswitch that recognizes guanine, and the researchers studied how this 'tandem riboswitch' responded to different combinations of high and low levels of guanine and PRPP. They found that gene expression was suppressed when levels of guanine were high and levels of PRPP were low: however, genes were expressed for the three other possible combinations (Figure 1). The fact that the tandem riboswitch is acting like a logic gate is not itself unusual. However, the logic operation performed by the tandem riboswitch (the IMPLY operation) has not been observed in nature before.
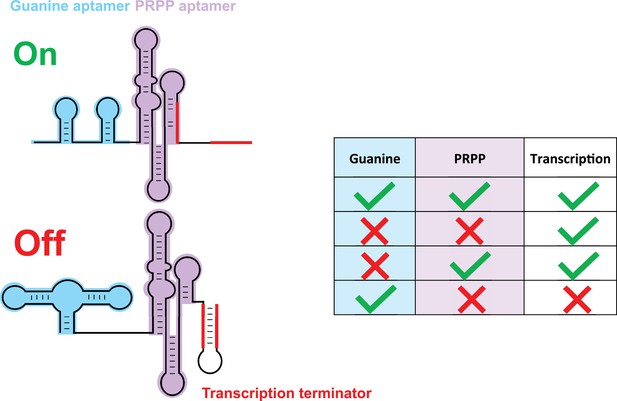
A tandem riboswitch as an IMPLY logic gate.
Sherlock et al. studied a tandem riboswitch, made of a guanine riboswitch (blue) and a PRPP riboswitch (purple), that regulates genes involved in purine biosynthesis. These genes are transcribed when PRPP binds to its riboswitch (first and third rows in table) or when neither guanine nor PRPP are available (second row). However, a transcription terminator structure (red) forms and blocks transcription when PRPP is absent and guanine binds to its riboswitch (last row). This is the only known example of an IMPLY logic gate in nature. The PRPP riboswitch belongs to the larger family of ykkC riboswitches, which is subdivided in three main groups based on their tertiary structures. The riboswitches in this family bind to guanidine and participate in the guanidine detoxification process (Battaglia et al., 2017; Huang et al., 2017a; Huang et al., 2017b; Nelson et al., 2017; Reiss and Strobel, 2017; Reiss et al., 2017; Sherlock and Breaker, 2017; Sherlock et al., 2017).
Why would an organism evolve such a logic gate? When bacteria are deprived of nutrients, and the levels of both guanine and PRPP are low, one would expect purine biosynthesis to be reduced as the bacteria attempt to conserve their resources. However, somewhat counter-intuitively, the tandem riboswitch ensures that the genes responsible for purine biosynthesis continue to be expressed. Sherlock et al. reasoned that this might have something to do with the fact that bacteria need to keep their levels of GTP low and their levels of ATP high in response to starvation. GTP levels are kept low because this molecule prevents bacteria from producing their own amino acids, which is a necessary step during nutrient deprivation. However, ATP levels are kept high to promote the transcription of genes that are involved in the stress response to starvation. The guanine-PRPP riboswitch regulates genes involved in the first steps of purine biosynthesis or the shuttling of these precursors to ATP synthesis pathways. The end outcome is that the tandem riboswitch allows the genes necessary for ATP production to stay on during starvation without stimulating the synthesis of GTP.
In the tandem riboswitches reported to date, the individual structures typically operate independently of each other, with their outputs being combined later to establish a higher-order logic gate. However, the tandem riboswitches described by Sherlock et al. must depend on each other in some way. Further research is needed to understand this interdependency in greater detail.
The versatility of the ykkC class of riboswitches – at least five types of ligands can be recognized – surpasses anything that has been seen in the riboswitches that have been characterized to date. What is so special about this class of riboswitches and why is it involved in the regulation of so many different sets of genes? We look forward to researchers in bioinformatics and structural biology working together in the future to improve our understanding of the evolution of riboswitches.
References
-
The structure of the guanidine-II riboswitchCell Chemical Biology 24:695–702.https://doi.org/10.1016/j.chembiol.2017.05.014
-
Structure of the guanidine III riboswitchCell Chemical Biology 24:1407–1415.https://doi.org/10.1016/j.chembiol.2017.08.021
-
Detection of 224 candidate structured RNAs by comparative analysis of specific subsets of intergenic regionsNucleic Acids Research 45:10811–10823.https://doi.org/10.1093/nar/gkx699
Article and author information
Author details
Publication history
Copyright
© 2018, Battaglia et al.
This article is distributed under the terms of the Creative Commons Attribution License, which permits unrestricted use and redistribution provided that the original author and source are credited.
Metrics
-
- 882
- views
-
- 96
- downloads
-
- 0
- citations
Views, downloads and citations are aggregated across all versions of this paper published by eLife.
Download links
Downloads (link to download the article as PDF)
Open citations (links to open the citations from this article in various online reference manager services)
Cite this article (links to download the citations from this article in formats compatible with various reference manager tools)
Further reading
-
- Biochemistry and Chemical Biology
- Genetics and Genomics
Yerba mate (YM, Ilex paraguariensis) is an economically important crop marketed for the elaboration of mate, the third-most widely consumed caffeine-containing infusion worldwide. Here, we report the first genome assembly of this species, which has a total length of 1.06 Gb and contains 53,390 protein-coding genes. Comparative analyses revealed that the large YM genome size is partly due to a whole-genome duplication (Ip-α) during the early evolutionary history of Ilex, in addition to the hexaploidization event (γ) shared by core eudicots. Characterization of the genome allowed us to clone the genes encoding methyltransferase enzymes that catalyse multiple reactions required for caffeine production. To our surprise, this species has converged upon a different biochemical pathway compared to that of coffee and tea. In order to gain insight into the structural basis for the convergent enzyme activities, we obtained a crystal structure for the terminal enzyme in the pathway that forms caffeine. The structure reveals that convergent solutions have evolved for substrate positioning because different amino acid residues facilitate a different substrate orientation such that efficient methylation occurs in the independently evolved enzymes in YM and coffee. While our results show phylogenomic constraint limits the genes coopted for convergence of caffeine biosynthesis, the X-ray diffraction data suggest structural constraints are minimal for the convergent evolution of individual reactions.
-
- Biochemistry and Chemical Biology
- Structural Biology and Molecular Biophysics
The SARS-CoV-2 main protease (Mpro or Nsp5) is critical for production of viral proteins during infection and, like many viral proteases, also targets host proteins to subvert their cellular functions. Here, we show that the human tRNA methyltransferase TRMT1 is recognized and cleaved by SARS-CoV-2 Mpro. TRMT1 installs the N2,N2-dimethylguanosine (m2,2G) modification on mammalian tRNAs, which promotes cellular protein synthesis and redox homeostasis. We find that Mpro can cleave endogenous TRMT1 in human cell lysate, resulting in removal of the TRMT1 zinc finger domain. Evolutionary analysis shows the TRMT1 cleavage site is highly conserved in mammals, except in Muroidea, where TRMT1 is likely resistant to cleavage. TRMT1 proteolysis results in reduced tRNA binding and elimination of tRNA methyltransferase activity. We also determined the structure of an Mpro-TRMT1 peptide complex that shows how TRMT1 engages the Mpro active site in an uncommon substrate binding conformation. Finally, enzymology and molecular dynamics simulations indicate that kinetic discrimination occurs during a later step of Mpro-mediated proteolysis following substrate binding. Together, these data provide new insights into substrate recognition by SARS-CoV-2 Mpro that could help guide future antiviral therapeutic development and show how proteolysis of TRMT1 during SARS-CoV-2 infection impairs both TRMT1 tRNA binding and tRNA modification activity to disrupt host translation and potentially impact COVID-19 pathogenesis or phenotypes.