Chemokines: The cell sets the tone
In the body, cells constantly ‘talk’ to each other via extracellular signaling molecules. When one of these molecules binds to a receptor on a cell, it generally activates a signaling pathway that leads to this cell doing something.
Chemokines are signaling molecules that mainly direct how cells migrate during immunity and developmental processes. Vertebrates have more than 40 chemokines and 20 chemokine receptors, which all belong to the well-known class of G protein coupled receptors. There is a certain amount of redundancy in the system, with some chemokines being able to bind to more than one type of receptor, and some receptors being able to bind different types of chemokines (Rot and von Andrian, 2004). Chemokines likely evolved to orchestrate the trafficking of numerous different types of immune cells, but some are also important in embryonic development (Wang and Knaut, 2014). Given the number of different signaling molecules and receptors involved, and the range of roles they play, how does the chemokine receptor-ligand system encode specificity? In other words, how does a cell ‘understand’ which pathway to activate when a chemokine binds to a chemokine receptor on its surface?
To answer this, researchers concentrated on the differences between the signaling pathways triggered by different chemokine receptors. They found that there is a ‘ligand bias’: a receptor can trigger different cellular responses depending on the ligand it binds (Steen et al., 2014). In these cases, the cell ‘knows’ how to respond to a signal because a specific ligand-receptor combination activates a unique cellular pathway (Figure 1A). Now, in eLife, Erez Raz of the University of Münster and colleagues – including Divyanshu Malhotra (Münster), Jimann Shin and Lilianna Solnica-Krezel (both at Washington University School of Medicine) – report results of experiments in zebrafish larvae that challenge the importance of specificity in chemokine recognition (Malhotra et al., 2018).
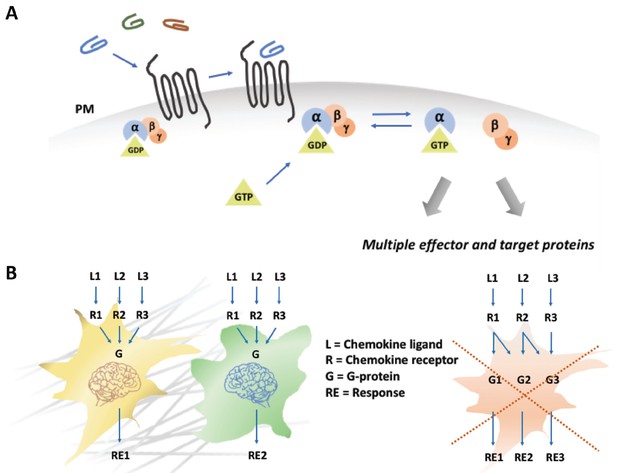
Chemokines trigger a 'yes or no' response in cells.
(A) When a chemokine (blue, green or brown line) binds to a chemokine receptor (black lines) embedded in the plasma membrane (PM; grey) of a cell, a G protein formed of three subunits (α, ß, γ), one of which (α) is attached to a molecule called GDP, is recruited. The GDP is then replaced with a molecule known as GTP, the G protein dissociates, and the different subunits go on to activate a range of different cellular pathways. (B) Different models can explain how chemokines signal within a cell. In the first model (left), different chemokines (L1, L2, L3) bind to their corresponding receptors (R1, R2, R3) and activate a generic G protein mediated pathway (G) in two types of cells (in yellow and green). The final response (RE1, RE2) triggered by a chemokine is ultimately dependent on interpretation modules (depicted as cellular brains) that are specific to the cell type, rather than on the identity of the signaling chemokine-receptor complex. The second model (right) proposes that each chemokine-receptor pairs activates a specific cellular pathway that determines a particular cellular response. The work by Malhotra et al. supports the first model (Malhotra et al., 2018).
The researchers took advantage of the fact that zebrafish have a duplicated genome, which means that many of the receptors and ligands come in two versions. Malhotra et al. also made use of the fact that chemokines act in a range of distinct developmental phenomena, such as the migration of germ cells, the adhesion of endoderm cells and the specification of cell fate during gastrulation. They used a combination of genetic approaches, together with imaging-based readouts, to test if receptor-ligand interactions (and the resulting signals) specified the biological process, or if the response depended on the cell type. In their genetic manipulations, the researchers gradually went from subtle to radical. They started by swapping the receptor-ligand pairs that arose due to the duplication of the zebrafish genome. The most extreme changes involved replacing a receptor-ligand pair with a pair that was involved in a completely different developmental process.
From all these experiments, Malhotra et al. got a surprisingly clear answer to their question. The cells more or less always behaved the way they would have if they still had their original receptors: germ cells migrated (albeit to the wrong place), endoderm cells adhered, and they differentiated during gastrulation. While most chemokines signal through the cell via two families of G protein subunits, Gαi and G12/13, further manipulations confirmed that all the different receptors used in the study signaled through the Gαi pathway. This means that the role of the chemokine receptors is to switch on the Gαi signaling cascade; it is then up to the cell to interpret that ‘yes or no’ signal and to trigger the right molecular processes. It is the cell type that dictates the response, not the receptor-ligand pair (Figure 1B). In simple words: no matter which chemokine a specific cell type 'smells', it will always respond the way it 'wants'. This is surprising given the wealth of research that describes different signal modalities for different chemokine receptors.
However, a large number of signaling processes, including many that involve G proteins, also work in such a modular way. A typical example is the system used by vertebrates to discriminate between different odors. In mice, over a thousand different G protein-coupled odor receptors are expressed in the tissue that lines the nose. Yet, a single olfactory sensory neuron does not carry a thousand different receptors, each signaling via a specific pathway. Instead, each neuron only expresses one type of receptor, which responds to only one chemical, and these neurons all work together to identify odors (Buck, 2000). An even more extreme case is the immune system, where every clone of B or T cells carries an individually assembled and therefore specific receptor at its surface. However, all these receptors signal to the cell via the same conserved pathway (Nussenzweig, 1998).
Such funneling of information through one common pathway creates a ‘weak regulatory linkage’ (Gerhart and Kirschner, 2007). In this situation, the input (cell senses something) is coupled to the output (cell does something) via a single conserved process that does not convey any specific information: rather it just signals yes or no. This process can be a membrane potential, a flux of calcium ions or a Gαi signal. This means that inputs and outputs, such as regulatory signals and functional responses, are free to evolve independently from each other. The work of Malhotra et al. provides an excellent example of weak regulatory linkage in the chemokine system.
References
-
Chemokines in innate and adaptive host defense: basic chemokinese grammar for immune cellsAnnual Review of Immunology 22:891–928.https://doi.org/10.1146/annurev.immunol.22.012703.104543
-
Biased and G protein-independent signaling of chemokine receptorsFrontiers in Immunology 5:277.https://doi.org/10.3389/fimmu.2014.00277
-
Chemokine signaling in development and diseaseDevelopment 141:4199–4205.https://doi.org/10.1242/dev.101071
Article and author information
Author details
Publication history
Copyright
© 2018, Alanko et al.
This article is distributed under the terms of the Creative Commons Attribution License, which permits unrestricted use and redistribution provided that the original author and source are credited.
Metrics
-
- 1,510
- views
-
- 150
- downloads
-
- 1
- citations
Views, downloads and citations are aggregated across all versions of this paper published by eLife.
Download links
Downloads (link to download the article as PDF)
Open citations (links to open the citations from this article in various online reference manager services)
Cite this article (links to download the citations from this article in formats compatible with various reference manager tools)
Further reading
-
- Developmental Biology
- Neuroscience
The blood-brain barrier (BBB) controls the movement of molecules into and out of the central nervous system (CNS). Since a functional BBB forms by mouse embryonic day E15.5, we reasoned that gene cohorts expressed in CNS endothelial cells (EC) at E13.5 contribute to BBB formation. In contrast, adult gene signatures reflect BBB maintenance mechanisms. Supporting this hypothesis, transcriptomic analysis revealed distinct cohorts of EC genes involved in BBB formation and maintenance. Here, we demonstrate that epigenetic regulator’s histone deacetylase 2 (HDAC2) and polycomb repressive complex 2 (PRC2) control EC gene expression for BBB development and prevent Wnt/β-catenin (Wnt) target genes from being expressed in adult CNS ECs. Low Wnt activity during development modifies BBB genes epigenetically for the formation of functional BBB. As a Class-I HDAC inhibitor induces adult CNS ECs to regain Wnt activity and BBB genetic signatures that support BBB formation, our results inform strategies to promote BBB repair.
-
- Developmental Biology
- Neuroscience
Williams syndrome (WS; OMIM#194050) is a rare disorder, which is caused by the microdeletion of one copy of 25–27 genes, and WS patients display diverse neuronal deficits. Although remarkable progresses have been achieved, the mechanisms for these distinct deficits are still largely unknown. Here, we have shown that neural progenitor cells (NPCs) in WS forebrain organoids display abnormal proliferation and differentiation capabilities, and synapse formation. Genes with altered expression are related to neuronal development and neurogenesis. Single cell RNA-seq (scRNA-seq) data analysis revealed 13 clusters in healthy control and WS organoids. WS organoids show an aberrant generation of excitatory neurons. Mechanistically, the expression of transthyretin (TTR) are remarkably decreased in WS forebrain organoids. We have found that GTF2IRD1 encoded by one WS associated gene GTF2IRD1 binds to TTR promoter regions and regulates the expression of TTR. In addition, exogenous TTR can activate ERK signaling and rescue neurogenic deficits of WS forebrain organoids. Gtf2ird1-deficient mice display similar neurodevelopmental deficits as observed in WS organoids. Collectively, our study reveals critical function of GTF2IRD1 in regulating neurodevelopment of WS forebrain organoids and mice through regulating TTR-ERK pathway.