Descending neurons: Disentangling the strings that organize behavior
The nervous systems of animals are typically divided into two parts: a brain in the head and either a ventral nerve cord or a spinal cord in the body. Each plays a role in helping the animal to navigate and interact with its environment. The brain combines information from a number of sources – including the senses, contextual information and the internal state of the animal – to select an appropriate behavior. The ventral nerve cord then coordinates the activity of motor neurons to perform the particular action selected by the brain.
Descending neurons connect the brain to the ventral nerve cord, and it has been estimated that at most 550 pairs of descending neurons exist in adult Drosophila fruit flies (Hsu and Bhandawat, 2016). Reaching a better understanding of the anatomy of these descending neurons is a necessary step toward describing the logic of behavioral control. Now, in eLife, Gwyneth Card of the Janelia Research Campus and colleagues – including Shigehiro Namiki as first author, Michael Dickinson, Allan Wong and Wyatt Korff – report a major advance toward this goal by working out a detailed map that traces the path of nearly half of the descending neurons (Namiki et al., 2018). In a second paper David Stern of Janelia, Gordon Berman of Emory University and colleagues – including Jessica Cande as first author, Namiki, Jirui Qui, Korff, Card and Joshua Shaevitz – report the results of experiments on behaviors associated with these descending neurons (Cande et al., 2018).
Using cutting-edge genetic tools, Namiki et al. labeled 190 pairs of descending neurons covering 54 distinct cell types. This allowed the neurons to be mapped from their origin in the brain to their destination in the ventral nerve cord. This anatomical analysis is rare in its depth and coverage. Although the function of a neural circuit cannot be unraveled from its wiring diagram alone (Bargmann and Marder, 2013), such knowledge does help to formulate testable hypotheses about the function of the circuit (Takemura et al., 2013; Takemura et al., 2017).
One striking finding reported by Namiki et al. is that descending neurons tend to be individual and unique rather than members of large populations of neurons with similar structures. An attempt to classify these neurons based on the location of their cell bodies, or where in the brain their inputs come from, was not particularly conclusive: neurons with distinct shapes and neurotransmitter types can have adjacent cell bodies. However, grouping the descending neurons based on the areas of the ventral nerve cord they target led to more coherent results. One group of descending neurons innervates the area of the ventral nerve cord that controls the legs, while another targets the nerves that connect to the wing, and a smaller group connects to an intermediate region called the tectulum (Court et al., 2017). This organization of the descending neurons supports a model in which Drosophila has separate streams of control for behaviors associated with its legs (such as walking) and its wings (such as flying or producing courtship songs).
On average, descending neurons connect three regions of the brain to two regions of the ventral nerve cord. The fact that most descending neurons receive and make so few connections suggests that sensory information is processed before it reaches them. Furthermore, it suggests that complex motor outputs are put into action downstream from the descending neurons. These hypotheses are corroborated by the results reported by Cande et al., who used optogenetics and sophisticated behavioral analysis to explore the behaviors elicited by different descending neurons. Activating some individual descending neurons produced simple and repetitive behaviors, but the same general class of behavior (such as walking or grooming) could be evoked by multiple different descending neurons.
Surprisingly, Namiki et al. report that descending neurons do not project exclusively into the ventral nerve cord: the majority of them take a detour through a region of the brain called the subesophageal zone. This zone, which is located under the jaw, processes taste and contains the motor neurons for extending the proboscis (Ito et al., 2014; Kendroud et al., 2018). Circuits in the subesophageal zone of larvae appear to integrate inputs from multiple senses to control how the larvae stop and start as they navigate toward a goal (Tastekin et al., 2015). The anatomical observations of Namiki et al. suggest that this area of the fly brain deserves further study.
Do neurons convey ‘decisions’ from the brain that activate pre-determined motor programs in the ventral nerve cord? According to the debated command neuron model, specific descending neurons would trigger whole coherent behavioral programs, such as courtship or grooming (Kristan, 2008). Although some behaviors might be controlled in this way (Bidaye et al., 2014; von Reyn et al., 2014), such cases are rare and cannot explain the range of complex actions a fly exhibits. Premotor circuits forming central pattern generators in the ventral nerve cord are sufficient to enable decapitated flies and ‘brainless’ larvae to execute coordinated motor programs (Berni et al., 2012; Harris et al., 2015). It is likely that a fly combines the activity of different descending neurons to select and combine the behaviors executed by central pattern generators.
How then is the full repertoire of behaviors directed through the descending neurons? A skilled puppeteer can give ‘life’ to a marionette by pulling on a few strings connected to individual limb joints. The nervous system of an animal faces an analogous challenge: sensory information integrated in the brain has to be conveyed to the motor neurons and muscles through a limited number of descending neurons. Namiki et al. reveals a comprehensive look at these ‘strings’ in Drosophila, and Cande et al. demonstrates what can happen when you pull on them, giving us the first steps toward disentangling the logic of behavior control (Figure 1).
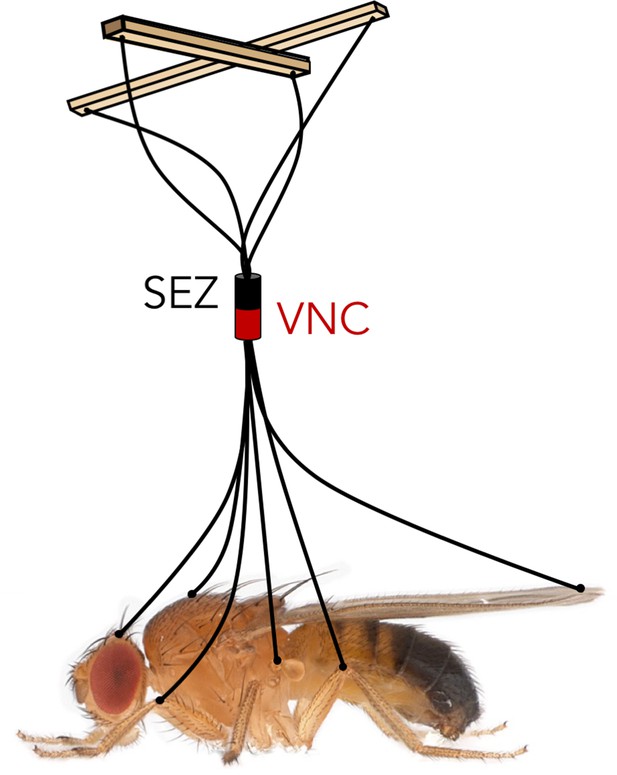
Like controlling the movements of a puppet using only a few strings, the fly brain must control the entire behavioral repertoire of the fly based on the information transmitted by a small set of descending neurons.
SEZ: subesophageal zone. VNC: ventral nerve cord.
IMAGE CREDIT: Matthieu Louis and Julie H Simpson, using a photograph of Drosophila melanogaster from Nicolas Gompel.
References
-
Organization of descending neurons in Drosophila melanogasterScientific Reports 6:20259.https://doi.org/10.1038/srep20259
-
Structure and development of the subesophageal zone of the Drosophila brain. II. sensory compartmentsJournal of Comparative Neurology 526:33–58.https://doi.org/10.1002/cne.24316
-
Neuronal decision-making circuitsCurrent Biology 18:R928–R932.https://doi.org/10.1016/j.cub.2008.07.081
-
A spike-timing mechanism for action selectionNature Neuroscience 17:962–970.https://doi.org/10.1038/nn.3741
Article and author information
Author details
Publication history
- Version of Record published: June 26, 2018 (version 1)
Copyright
© 2018, Louis et al.
This article is distributed under the terms of the Creative Commons Attribution License, which permits unrestricted use and redistribution provided that the original author and source are credited.
Metrics
-
- 3,061
- views
-
- 232
- downloads
-
- 2
- citations
Views, downloads and citations are aggregated across all versions of this paper published by eLife.
Download links
Downloads (link to download the article as PDF)
Open citations (links to open the citations from this article in various online reference manager services)
Cite this article (links to download the citations from this article in formats compatible with various reference manager tools)
Further reading
-
- Neuroscience
In most animals, the brain makes behavioral decisions that are transmitted by descending neurons to the nerve cord circuitry that produces behaviors. In insects, only a few descending neurons have been associated with specific behaviors. To explore how descending neurons control an insect’s movements, we developed a novel method to systematically assay the behavioral effects of activating individual neurons on freely behaving terrestrial D. melanogaster. We calculated a two-dimensional representation of the entire behavior space explored by these flies, and we associated descending neurons with specific behaviors by identifying regions of this space that were visited with increased frequency during optogenetic activation. Applying this approach across a large collection of descending neurons, we found that (1) activation of most of the descending neurons drove stereotyped behaviors, (2) in many cases multiple descending neurons activated similar behaviors, and (3) optogenetically activated behaviors were often dependent on the behavioral state prior to activation.
-
- Computational and Systems Biology
- Neuroscience
Normal aging leads to myelin alterations in the rhesus monkey dorsolateral prefrontal cortex (dlPFC), which are positively correlated with degree of cognitive impairment. It is hypothesized that remyelination with shorter and thinner myelin sheaths partially compensates for myelin degradation, but computational modeling has not yet explored these two phenomena together systematically. Here, we used a two-pronged modeling approach to determine how age-related myelin changes affect a core cognitive function: spatial working memory. First, we built a multicompartment pyramidal neuron model fit to monkey dlPFC empirical data, with an axon including myelinated segments having paranodes, juxtaparanodes, internodes, and tight junctions. This model was used to quantify conduction velocity (CV) changes and action potential (AP) failures after demyelination and subsequent remyelination. Next, we incorporated the single neuron results into a spiking neural network model of working memory. While complete remyelination nearly recovered axonal transmission and network function to unperturbed levels, our models predict that biologically plausible levels of myelin dystrophy, if uncompensated by other factors, can account for substantial working memory impairment with aging. The present computational study unites empirical data from ultrastructure up to behavior during normal aging, and has broader implications for many demyelinating conditions, such as multiple sclerosis or schizophrenia.