Patterning: Fishing for ancestry
The tough scales found on alligators, the colourful feathers of peacocks and the thick fur of polar bears are all examples of skin appendages – self-contained mini-organs that form a barrier between an organism and its environment (Chuong et al., 2013). These skin appendages are essential for providing protection, retaining heat, sensing movement, attracting mates and, in some animals, flying. How these immensely complex and diverse structures have evolved, and how they develop, has fascinated researchers for decades.
Due to the sheer variety of skin appendages in both living and fossilised animals, it is still unclear whether they evolved independently or from a common ancestor (Dhouailly et al., 2017). However, as some of the earliest processes involved in the development of feathers and hair are very similar, the basic genetic mechanisms underlying their formation could have shared evolutionary origins (Musser et al., 2015). Indeed, researchers recently found that the cell signalling events involved in the development of scales in reptiles and the events regulating the patterning of appendages in birds and mammals have many similarities (Di-Poï and Milinkovitch, 2016). For example, slightly altering gene expression in reptile scales or the scaly foot of a chicken can lead to the formation of feather buds and primitive feathers respectively, akin to those found in dinosaurs (Wu et al., 2018).
Therefore, it seems likely that the skin appendages of reptiles, birds and mammals – collectively known as amniotes – have a shared evolutionary origin. Fish scales appear to be different in that they are made of bone instead of keratin, and also derive from a different layer of skin. However, is it possible that amniotes and fish share a common, ancestral skin appendage that existed even further back in time than was previously thought? Now, in eLife, Andrew Aman, Alexis Fulbright and David Parichy of the University of Virginia report the results of experiments on zebrafish which suggest that this might be the case (Aman et al., 2018).
Through beautifully detailed live imaging of developing scales and the manipulation of key signalling pathways during their development, Aman et al. were able to study how zebrafish scales form and arrange themselves correctly on the skin. The first hint that appendage patterning in fish is similar to that of amniotes came from close observation of scale development, which occurred sequentially in a hexagonal grid pattern to cover the fish, a patterning strategy shared with the land animals.
Aman et al. then investigated whether the cell signalling mechanisms underpinning these morphological events might also be conserved at the molecular level. In all amniote species investigated so far, the earliest signalling event in appendage formation is the activation of the Wnt signalling pathway, which regulates many aspects of cell development and behaviour. Using genetically modified fish to highlight Wnt-signalling cells, it was possible to identify clusters of cells congregating at sites that would later form scales. Aman et al. showed that Wnt signalling and the subsequent activation of another signalling pathway, the Eda pathway, were both necessary for the scales to develop, and that blocking either pathway caused a complete loss of scale formation. This skin appendage loss is consistent with the one observed in fish, bearded lizards, chicks, mice and humans with defective Eda signalling (Harris et al., 2008; Sennett and Rendl, 2012; Drew et al., 2007; Di-Poï and Milinkovitch, 2016).
Next, Aman et al. disrupted combinations of these and other signalling systems known to be involved in the development of skin appendages in amniotes, to expand our knowledge of appendage development. These experiments revealed that, similar to birds and mammals, another signalling system that is important for the development and repair of cells, the fibroblast growth factor system, was required for the scales to differentiate further (Mandler and Neubüser, 2004; Huh et al., 2013). Moreover, the researchers discovered a new role for another well-known signalling system, the Sonic hedgehog system, which instructed the top layer of the skin to fold over the scales, similar to the skin movements that occur as hair follicles develop (St-Jacques et al., 1998). These similarities hint at the existence of a single, ancient origin of appendage patterning in vertebrates (Figure 1).
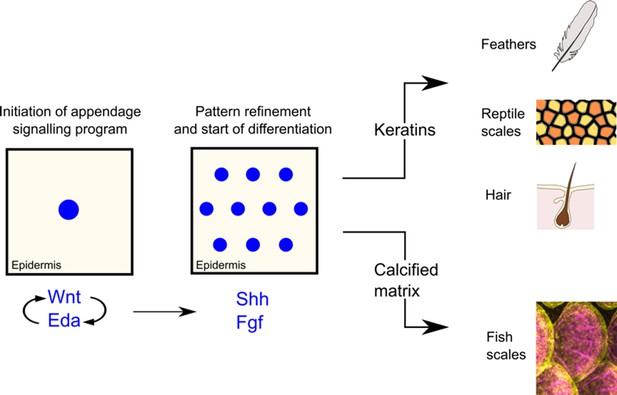
Simplified schematic illustrating the development of skin appendages.
The development of skin appendages is initiated by the activation of the Wnt signalling pathway in the top layer of the skin (the epidermis), and this signal is refined and maintained by the Eda signalling pathway. Both systems activate two further pathways that are important for cell growth and development: fibroblast growth factor (Fgf) signalling is necessary to ensure that the patterning of the appendages happens correctly and that scales are able to form; the Sonic hedgehog pathway ensures that the epidermis folds properly over the scales. Fish scales are formed from bone, whereas feathers, reptile scales and hair are formed from keratin. However, over time a common ancestor may have stopped using bone and started using keratin to build skin appendages in amniotes.
Discovering such conserved gene regulatory networks in the zebrafish – an organism that is so readily amenable to high-resolution imaging and genetic manipulation – is very exciting. In amniotes, these fleeting events mainly occur in the womb or egg, which makes it difficult to collect stage-matched animals and use live imaging – a challenge in identifying key signalling processes (Sennett and Rendl, 2012).
If we could further untangle the contribution of various genes involved in the development of skin appendages, we might be able to apply this knowledge to tissue engineering (by, for example, regulating hair patterning on skin grafts). Also, by identifying new genes or functions of genes in appendage development, zebrafish could help us understand and ultimately treat skin disorders in humans.
References
-
Module-based complexity formation: periodic patterning in feathers and hairsWiley Interdisciplinary Reviews: Developmental Biology 2:97–112.https://doi.org/10.1002/wdev.74
-
The Edar subfamily in feather placode formationDevelopmental Biology 305:232–245.https://doi.org/10.1016/j.ydbio.2007.02.011
-
Mesenchymal-epithelial interactions during hair follicle morphogenesis and cyclingSeminars in Cell & Developmental Biology 23:917–927.https://doi.org/10.1016/j.semcdb.2012.08.011
-
Sonic hedgehog signaling is essential for hair developmentCurrent Biology 8:1058–1069.https://doi.org/10.1016/S0960-9822(98)70443-9
-
Multiple regulatory modules are required for Scale-to-Feather conversionMolecular Biology and Evolution 35:417–430.https://doi.org/10.1093/molbev/msx295
Article and author information
Author details
Publication history
Copyright
© 2018, Brunsdon et al.
This article is distributed under the terms of the Creative Commons Attribution License, which permits unrestricted use and redistribution provided that the original author and source are credited.
Metrics
-
- 1,790
- views
-
- 135
- downloads
-
- 1
- citations
Views, downloads and citations are aggregated across all versions of this paper published by eLife.
Download links
Downloads (link to download the article as PDF)
Open citations (links to open the citations from this article in various online reference manager services)
Cite this article (links to download the citations from this article in formats compatible with various reference manager tools)
Further reading
-
- Cell Biology
- Developmental Biology
Fibroblast growth factor (FGF) signaling elicits multiple downstream pathways, most notably the Ras/MAPK cascade facilitated by the adaptor protein Grb2. However, the mechanism by which Grb2 is recruited to the FGF signaling complex remains unresolved. Here, we showed that genetic ablation of FGF signaling prevented murine lens induction by disrupting transcriptional regulation and actin cytoskeletal arrangements, which could be reproduced by deleting the juxtamembrane region of the FGF receptor and rescued by Kras activation. Conversely, mutations affecting the Frs2-binding site on the FGF receptor or the deletion of Frs2 and Shp2 primarily impact later stages of lens vesicle development involving lens fiber cell differentiation. Our study further revealed that the loss of Grb2 abolished MAPK signaling, resulting in a profound arrest of lens development. However, removing Grb2’s putative Shp2 dephosphorylation site (Y209) neither produced a detectable phenotype nor impaired MAPK signaling during lens development. Furthermore, the catalytically inactive Shp2 mutation (C459S) only modestly impaired FGF signaling, whereas replacing Shp2’s C-terminal phosphorylation sites (Y542/Y580) previously implicated in Grb2 binding only caused placental defects, perinatal lethality, and reduced lacrimal gland branching without impacting lens development, suggesting that Shp2 only partially mediates Grb2 recruitment. In contrast, we observed that FGF signaling is required for the phosphorylation of the Grb2-binding sites on Shc1 and the deletion of Shc1 exacerbates the lens vesicle defect caused by Frs2 and Shp2 deletion. These findings establish Shc1 as a critical collaborator with Frs2 and Shp2 in targeting Grb2 during FGF signaling.
-
- Developmental Biology
- Evolutionary Biology
The insect brain and the timing of its development underwent evolutionary adaptations. However, little is known about the underlying developmental processes. The central complex of the brain is an excellent model to understand neural development and divergence. It is produced in large parts by type II neuroblasts, which produce intermediate progenitors, another type of cycling precursor, to increase their neural progeny. Type II neuroblasts lineages are believed to be conserved among insects, but little is known on their molecular characteristics in insects other than flies. Tribolium castaneum has emerged as a model for brain development and evolution. However, type II neuroblasts have so far not been studied in this beetle. We created a fluorescent enhancer trap marking expression of Tc-fez/earmuff, a key marker for intermediate progenitors. Using combinatorial labeling of further markers, including Tc-pointed, we characterized embryonic type II neuroblast lineages. Intriguingly, we found nine lineages per hemisphere in the Tribolium embryo while Drosophila produces only eight per brain hemisphere. These embryonic lineages are significantly larger in Tribolium than they are in Drosophila and contain more intermediate progenitors. Finally, we mapped these lineages to the domains of head patterning genes. Notably, Tc-otd is absent from all type II neuroblasts and intermediate progenitors, whereas Tc-six3 marks an anterior subset of the type II lineages. Tc-six4 specifically marks the territory where anterior-medial type II neuroblasts differentiate. In conclusion, we identified a conserved pattern of gene expression in holometabolan central complex forming type II neuroblast lineages, and conserved head patterning genes emerged as new candidates for conferring spatial identity to individual lineages. The higher number and greater lineage size of the embryonic type II neuroblasts in the beetle correlate with a previously described embryonic phase of central complex formation. These findings stipulate further research on the link between stem cell activity and temporal and structural differences in central complex development.