Tuberculosis: Breaking down walls
The rise of antimicrobial resistance has significantly reduced the options available for treating tuberculosis (TB) and other diseases caused by bacteria, threatening to plunge humanity back into the pre-antibiotic era. Mycobacterium tuberculosis is a rod-shaped bacterium that is responsible for around 10 million new infections and 1.4 million deaths per year (Pai et al., 2016). With an estimated 0.5 million cases of drug-resistant TB every year, this disease now accounts for a substantial fraction of the global burden of antimicrobial resistance (WHO, 2018). The current treatment for drug-resistant TB involves taking a combination of antibiotics over a period of up to 24 months, or six months for drug-sensitive TB. Such extensive treatment periods, with associated side effects, including deafness, create an urgent need for new drugs that shorten the duration of treatment, reduce the daily pill burden, and are effective against drug-resistant strains.
As the bacterial cell wall has formed the basis of successful antibiotic therapy for decades, the search for new TB drugs has led to renewed interest in the mycobacterial cell wall as a drug target (Alderwick et al., 2015). Mycobacteria, a genus that also includes the bacteria that cause leprosy and Buruli ulcer, have a complex cell wall that consists of an outer capsule-like layer, anchored by long-chain fatty acids known as mycolic acids, and two polymers (Kieser and Rubin, 2014). One of these polymers is called arabinogalactan; the other one, peptidoglycan, is made from monomers that contain a sugar molecule and a short peptide chain that consists of three to five amino acids. The sugar molecules bind together to form a long chain that is further strengthened by the formation of crosslinks between neighboring peptide chains; mainly between the third amino acids on both peptide chains (to form a 3–3 crosslink), or between the fourth amino acid of one and the third amino acid of the other (to form a 4–3 crosslink) (Raghavendra et al., 2018).
Current tuberculosis drugs that target the cell wall affect the synthesis of the mycolic acids and the arabinogalactan layer, but none are directed at peptidoglycan. Paradoxically, this polymer (in particular the cross-linking process) has been a successful drug target in other bacterial infections, but treating tuberculosis with the same drugs has so far been fruitless. This is mainly due to inherent mechanisms in M. tuberculosis that inactivate certain types of antibiotics, and to the fact that we know relatively little about how this polymer is synthesized and remodeled as the bacteria spread (Story-Roller and Lamichhane, 2018). Now, in eLife, two independent groups of researchers report results that advance our understanding of these processes (García-Heredia et al., 2018; Baranowski et al., 2018).
As bacteria grow and divide, peptidoglycan needs to be constantly broken down and reassembled. In other rod-shaped bacteria, such as Escherichia coli, growth is achieved by the insertion of new peptidoglycan units along the sidewall. However, the way mycobacteria grow is very different. Their growth happens at the polar regions (that is, at each end of the bacterium) through the addition of new units of peptidoglycan to the sub-polar region of the cell wall. Mycobacteria further lack homologues of E. coli proteins that direct peptidoglycan growth to the sidewall. Consequently, in mycobacteria, rapidly changing peptidoglycan segregates to the cell poles, while inactive peptidoglycan remains at the sidewall (Figure 1). However, preliminary observations have suggested an active sidewall peptidoglycan 'metabolism', but definitive evidence of this phenomenon, together with a detailed mechanistic explanation, has been lacking.
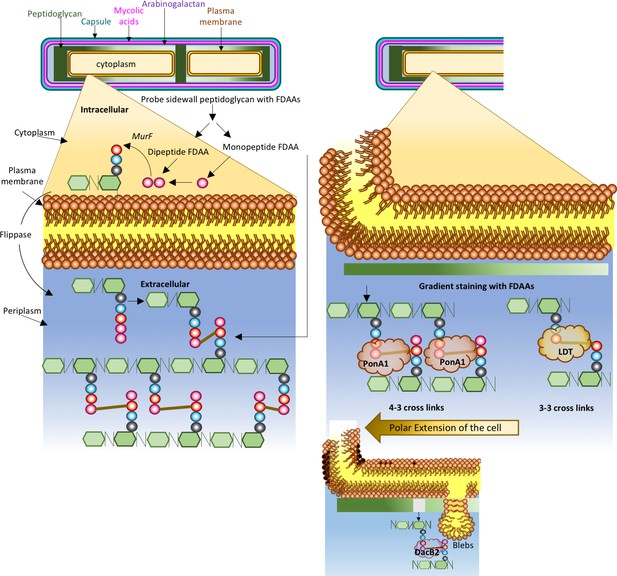
The synthesis and remodeling of peptidoglycan in mycobacteria during growth.
The cell wall of a mycobacterium consists of an outer capsule-like layer (teal line), mycolic acids (magenta line), arabinogalactan (purple line), peptidoglycan (green lines) and a bilayer of fatty acids comprising the cell membrane (orange line and circles with tails). These protect the cytoplasm, which is surrounded by the periplasm. Peptidoglycan is made from monomers that contain a sugar molecule (green hexagon) and a chain of three to five amino acids (different colored circles). Precursor peptidoglycan units are synthesized inside the cell and linked to a lipid carrier. These units are transported into the periplasm by an enzyme called a flippase. Crosslinks (brown lines) between the monomers lead to the formation of a rigid matrix that encases the cell. Garcia-Heredia et al. (left) used monopeptide and dipeptide FDAA probes (one and two pink circles respectively) to study both the synthesis of new peptidoglycan and the remodeling of existing peptidoglycan: the monopeptide probes can be incorporated into peptidoglycan (in the place of the amino acid D-Alanine) both inside and outside the cell, whereas the dipeptide probes can only be incorporated inside the cell. Previously, it was thought that growth in mycobacteria only happened at the poles, where peptidoglycan is active. However, the work of Garcia-Heredia et al. suggests that there is a gradient of peptidoglycan activity along the sidewall of the dividing mycobacterium, with a maximum (dark green) near the poles and a minimum (pale green) near the center, and that the synthesis and the remodeling can both occur at the sidewall. Baranowski et al. (right) confirm that the side wall contains active peptidoglycan. Moreover, they show that the crosslinks change from the 4–3 confirmation at the poles to the 3–3 confirmation at the sidewall. The 3–3 crosslinks are formed by enzymes called L,D-transpeptidases (LDTs); deleting these enzymes affects the activity of DacB2, an endopeptidase that can break down peptidoglycan, which leads to the formation of blebs (see inset) and damage to the cell wall. The 4–3 crosslinks are formed by an enzyme called PonA1, which is a D,D-transpeptidase. Taken together, these two studies provide a revised paradigm for peptidoglycan remodeling in mycobacteria that could help with the development of new drugs to target the mycobacteria that cause diseases such as tuberculosis.
Sloan Siegrist of the University of Massachusetts and colleagues – including Alam García-Heredia and Amol Arunrao Pohane as joint first authors – report the results of experiments on M. tuberculosis and its close relative, M. smegmatis, where they explore peptidoglycan biology further (García-Heredia et al., 2018). The team used reagents called fluorescent D-amino acids (FDAAs) to monitor the arrival and distribution of new peptidoglycan units both inside and outside the cell, as well as the remodeling of peptidoglycan outside the cell (Figure 1). They found that the proteins involved in the synthesis of new peptidoglycan units and remodeling of existing polymers can be found at both cell poles and at the sidewall. The amount of polymer incorporated into the sidewall decreased gradually from the poles to the middle of the cell. The cells only grew from the poles, suggesting that the remodeling process in the sidewalls may have a different purpose.
García-Heredia et al. then used antibiotics to either block the synthesis of new peptidoglycan units or the remodeling process, and found that both processes affected the incorporation of FDAAs into the cell wall differently. This indicated that peptidoglycan precursors are produced and remodeled along the sidewall of mycobacteria. Moreover, a group of enzymes called L,D-transpeptidases appeared to play a crucial role during peptidoglycan remodeling or repair when the bacterium responded to peptidoglycan damage. To back this up further, FDAAs marking the new production sites were predominantly incorporated at the sidewall when the bacteria were exposed to peptidoglycan-damaging antimicrobials.
In independent work, Hesper Rego of Yale, Eric Rubin of Harvard and colleagues – including Catherine Baranowski as first author – report results on how the structural properties of peptidoglycan change as the polymer ages during bacterial growth (Baranowski et al., 2018). Mycobacteria distinguish themselves from other bacteria by having a large proportion of 3–3 crosslinks in their peptidoglycan, whereas bacteria such as E. coli tend to have a very high proportion of 4–3 crosslinks.
Baranowski et al. also used FDAAs and found that the amount of probe incorporated into the sidewall decreased from the cell poles to the middle of the cell, as García-Heredia et al. had reported. However, when they removed all L,D-transpeptidases, the enzymes that drive the formation of 3–3 crosslinks, FDAA uptake was reduced, the bacteria lost their rod shape, and bulges called 'blebs' started to appear in the cell wall. Atomic force microscopy then revealed that the cell wall at a bleb was weaker than it was elsewhere.
Baranowski et al. propose that peptidoglycan at the cell pole most likely consists of 4–3 crosslinks. However, as the cell grows, peptidoglycan ages and moves to the sidewall, where it is remodeled into the 3–3 conformation (Figure 1). Consistent with this, D,D-transpeptidases, the enzymes that help to form 4–3 crosslinks, preferred to stay at the cell pole, while L,D-transpeptidases were predominantly found on the sidewall.
These observations confirm that the sidewall of the mycobacteria undergoes continuous restructuring, a process that possibly renews aged peptidoglycan. Moreover, the remodeling process relies on the careful coordination and distinct spatial patterning of various proteins, including the enzymes for the formation of 4–3 crosslinks and 3–3 crosslinks. The change from the 4–3 to the 3–3 confirmation is needed to increase the strength of peptidoglycan in the side wall and to maintain the rod shape of cells as they grow.
Collectively, the two studies provide unique mechanistic insights into the metabolism of peptidoglycan and how mycobacteria coordinate their growth. FDAA markers in combination with some of the genetic tools used provide an ideal starting point from which to develop screens for candidate drugs that target peptidoglycan biosynthesis and remodeling. Further study of these processes will undoubtedly drive the identification of new antimicrobials that could meaningfully add to the existing arsenal of tuberculosis drugs. As peptidoglycan can be found in many pathogenic bacteria, the new drugs that emerge from such efforts might also be able to treat other infectious diseases.
References
-
The mycobacterial cell wall--peptidoglycan and arabinogalactanCold Spring Harbor Perspectives in Medicine 5:a021113.https://doi.org/10.1101/cshperspect.a021113
-
How sisters grow apart: mycobacterial growth and divisionNature Reviews Microbiology 12:550–562.https://doi.org/10.1038/nrmicro3299
-
Peptidoglycan in mycobacteria: chemistry, biology and interventionGlycoconjugate Journal 35:421–432.https://doi.org/10.1007/s10719-018-9842-7
Article and author information
Author details
Publication history
Copyright
© 2018, Shaku et al.
This article is distributed under the terms of the Creative Commons Attribution License, which permits unrestricted use and redistribution provided that the original author and source are credited.
Metrics
-
- 4,248
- views
-
- 203
- downloads
-
- 3
- citations
Views, downloads and citations are aggregated across all versions of this paper published by eLife.
Download links
Downloads (link to download the article as PDF)
Open citations (links to open the citations from this article in various online reference manager services)
Cite this article (links to download the citations from this article in formats compatible with various reference manager tools)
Further reading
-
- Biochemistry and Chemical Biology
- Microbiology and Infectious Disease
Rod-shaped mycobacteria expand from their poles, yet d-amino acid probes label cell wall peptidoglycan in this genus at both the poles and sidewall. We sought to clarify the metabolic fates of these probes. Monopeptide incorporation was decreased by antibiotics that block peptidoglycan synthesis or l,d-transpeptidation and in an l,d-transpeptidase mutant. Dipeptides complemented defects in d-alanine synthesis or ligation and were present in lipid-linked peptidoglycan precursors. Characterizing probe uptake pathways allowed us to localize peptidoglycan metabolism with precision: monopeptide-marked l,d-transpeptidase remodeling and dipeptide-marked synthesis were coincident with mycomembrane metabolism at the poles, septum and sidewall. Fluorescent pencillin-marked d,d-transpeptidation around the cell perimeter further suggested that the mycobacterial sidewall is a site of cell wall assembly. While polar peptidoglycan synthesis was associated with cell elongation, sidewall synthesis responded to cell wall damage. Peptidoglycan editing along the sidewall may support cell wall robustness in pole-growing mycobacteria.
-
- Epidemiology and Global Health
- Genetics and Genomics
Alzheimer’s disease (AD) is a complex degenerative disease of the central nervous system, and elucidating its pathogenesis remains challenging. In this study, we used the inverse-variance weighted (IVW) model as the major analysis method to perform hypothesis-free Mendelian randomization (MR) analysis on the data from MRC IEU OpenGWAS (18,097 exposure traits and 16 AD outcome traits), and conducted sensitivity analysis with six models, to assess the robustness of the IVW results, to identify various classes of risk or protective factors for AD, early-onset AD, and late-onset AD. We generated 400,274 data entries in total, among which the major analysis method of the IVW model consists of 73,129 records with 4840 exposure traits, which fall into 10 categories: Disease, Medical laboratory science, Imaging, Anthropometric, Treatment, Molecular trait, Gut microbiota, Past history, Family history, and Lifestyle trait. More importantly, a freely accessed online platform called MRAD (https://gwasmrad.com/mrad/) has been developed using the Shiny package with MR analysis results. Additionally, novel potential AD therapeutic targets (CD33, TBCA, VPS29, GNAI3, PSME1) are identified, among which CD33 was positively associated with the main outcome traits of AD, as well as with both EOAD and LOAD. TBCA and VPS29 were negatively associated with the main outcome traits of AD, as well as with both EOAD and LOAD. GNAI3 and PSME1 were negatively associated with the main outcome traits of AD, as well as with LOAD, but had no significant causal association with EOAD. The findings of our research advance our understanding of the etiology of AD.