Tropical Diseases: Can CRISPR help in the fight against parasitic worms?
Parasitic worms, such as roundworms and flatworms, are amongst the most complex pathogens on Earth. They infect people and animals across the globe, and are the sixth leading cause of morbidity worldwide, being responsible for multiple neglected tropical diseases (World Health Organization, 2018; Hotez et al., 2008). There are no vaccines to prevent these infections, and while drug treatments are available, they have a narrow spectrum of action, their efficiency is poor, and there are problems with drug resistance (Hewitson and Maizels, 2014; Geary, 2012). New treatments are urgently needed, but the parasites are so complex that it is challenging to find tools to explore their biology, which hinders the development of drugs and vaccines.
The flatworms Opisthorchis viverrini and Schistosoma mansoni are two species responsible for human disease. S. mansoni causes schistosomiasis, which kills over 200,000 people every year (Lewis and Tucker, 2014). Waterborne S. mansoni larvae burrow into human skin and find their way into the bloodstream. Their eggs can become embedded in tissues and release molecules that cause inflammation and chronic disease, such as the omega-1 ribonuclease (coded by the Sm-omega-1 gene). People get infected with O. viverrini when they eat undercooked fish that carry the larvae. The parasites settle into the liver, where they can trigger cholangiocarcinoma, a type of bile duct cancer that has one of the highest mortality rates of any cancer (Sripa et al., 2012). An O. viverrini protein called granulin, which is coded by the Ov-grn-1 gene, may encourage liver cells to multiply abnormally.
The past decade has seen a concerted effort to sequence the genomes of parasitic worms, and more than 150 are available on the community-driven website WormBase ParaSite (Howe et al., 2017). Genomic tools that help dissect the roles of the parasites’ genes are now required to effectively exploit these datasets. In some species, RNA interference methods can silence gene transcripts, but these techniques can only knock down a gene — they cannot increase its expression or incorporate a new piece of genetic information. This limits the range of phenotypes that can be obtained by manipulating a given target (Dalzell et al., 2012). Also, while it is possible to introduce new sequences into schistosome genomes, these changes remain transient (Beckmann and Grevelding, 2012).
CRISPR/Cas9 is a new genetic tool that has revolutionized functional genomics in many organisms by triggering precise, heritable changes to a genome. In 2017, a team at UCLA used CRISPR to manipulate the genetic information of human-parasitic roundworms (Gang et al., 2017). Now, in two papers in eLife, researchers report having harnessed CRISPR/Cas9 to edit the genomes of O. viverrini and S. mansoni flatworms (Arunsan et al., 2019; Ittiprasert et al., 2019).
From a technical perspective, CRISPR methods require several components to get inside the target cell or tissue. These elements include an enzyme (often Cas9) that can cut DNA, and a single-guide RNA that helps bring Cas9 to the right cleavage site. There, the enzyme snips both strands of DNA, creating a break that can be repaired through a non-homologous end joining mechanism. This error-prone process can introduce mutations in the target site, potentially deactivating a gene. If desired, CRISPR/Cas9 can also be used to introduce new DNA. In this case, a DNA template is provided alongside Cas9 and the single-guide RNA. The sequence is formatted so that it can be inserted into the cleavage site using homology-directed repair mechanisms.
The two latest papers demonstrate that both non-homologous end joining and homology-directed repair appear active in flatworms. In one paper, Paul Brindley of George Washington University, Thewarach Laha of Khon Kaen University and colleagues – including Patpicha Arunsan, Wannaporn Ittiprasert and Michael Smout as joint first authors – describe how they used electroporation to introduce Cas9 and a single guide RNA into O. viverrini cells. The Ov-grn-1 gene was targeted, and edited through non-homologous end joining (Figure 1A; Arunsan et al., 2019).
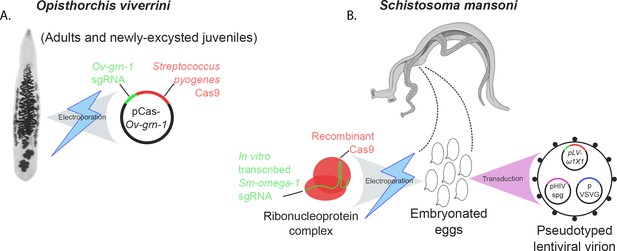
Transfection methods used to generate gene-edited flatworm parasites.
(A) CRISPR-Cas9 editing of the parasitic flatworm Opisthorchis viverrini (left) involved using a technique called electroporation. This forced cells to accept a bacterial plasmid construct (black circle) that encodes a Cas9 enzyme (red) and a guide RNA (green) that targets the Ov-grn-1 gene in the flatworm. The gene codes for a protein that may help trigger liver cancer in infected individuals. (B) The adult female Schistosoma mansoni (thinner dark gray worm of the pair) produces eggs while embraced by the male worm (broader light gray worm of the pair). Two methods were used to introduce CRISPR-related materials into these eggs. Electroporation helps the pre-complexed Cas9 protein (red) and strand of guide RNA (green) to get inside the eggs. Alternatively, a lentiviral virion (a particle derived from a virus; circle with black spots) can encapsulate and deliver these elements into cells. The virion also carries several plasmids – pLV-ω1 × 1 (green and red), pHIV spg (magenta), VSVG (blue) – which help the virus package and insert Cas9 and the single guide RNA inside cells. Both approaches target the Sm-omega-1 gene, which codes for a protein that may be involved in damaging the organs of people who carry the worm.
In the other paper, Brindley and co-workers at various institutes in the United States, United Kingdom, Thailand and the Netherlands – including Ittiprasert as first author – report using two methods, electroporation and a viral vector, to deliver CRISPR-related materials to S. mansoni eggs (Figure 1B; Ittiprasert et al., 2019). These included a DNA template that encoded a string of stop codons, to be incorporated into Sm-omega-1 through homology-directed repair.
In both studies, interrupting target genes reduced transcription and led to aberrant phenotypes, even though editing efficiency was very low. For example, in O. viverrini, CRISPR-induced mutations were found in fewer than 2% of the targeted sites, yet the levels of Ov-grn-1 transcript and protein were reduced within 48h of transfection. The livers of animals infected with edited parasites were less swollen, and their biliary ducts had less thickening and scarring compared to the organs of individuals carrying normal worms.
In S. mansoni, analyses of genetic variations showed that mutations due to non-homologous end joining were present in less than 4.5% of Sm-omega-1 target sites, and that the template of stop codons had been incorporated only 0.19% of the time. Despite such low editing efficiency, immune cells reacted less to manipulated eggs than to the non-manipulated ones. Mouse tissues that carried these edited eggs also showed reduced signs of inflammation.
According to both papers, an interplay between gene expression patterns and Cas9 penetrance explains how low editing efficacy can lead to seemingly disproportionate changes in phenotype. The next task could be to determine whether it is possible to improve the editing process, and evaluate how this would influence phenotypic outcomes. Alternatively, homology-directed repair mechanism could be used to label genetically manipulated organisms with a marker (e.g., green fluorescent proteins), which would help separate edited and non-edited animals before examining them for changes in phenotype. Overall, this work shows for the first time how to edit flatworm genomes using CRISPR/Cas9. This will likely initiate a step-change in research into these organisms: it may become possible to create genetically modified worm lines in which to study the biology of the parasites, how they cause disease, and ultimately, how they could be controlled.
References
-
Paving the way for transgenic schistosomesParasitology 139:651–668.https://doi.org/10.1017/S0031182011001466
-
Targeted mutagenesis in a human-parasitic nematodePLOS Pathogens 13:e1006675.https://doi.org/10.1371/journal.ppat.1006675
-
Are new anthelmintics needed to eliminate human helminthiases?Current Opinion in Infectious Diseases 25:709–717.https://doi.org/10.1097/QCO.0b013e328359f04a
-
Vaccination against helminth parasite infectionsExpert Review of Vaccines 13:473–487.https://doi.org/10.1586/14760584.2014.893195
-
Helminth infections: The great neglected tropical diseasesJournal of Clinical Investigation 118:1311–1321.https://doi.org/10.1172/JCI34261
-
WormBase ParaSite - a comprehensive resource for helminth genomicsMolecular and Biochemical Parasitology 215:2–10.https://doi.org/10.1016/j.molbiopara.2016.11.005
-
Digenetic TrematodesAdvances in Experimental Medicine and Biology, Digenetic Trematodes, 766, New York, Springer.
-
The tumorigenic liver fluke Opisthorchis viverrini -- multiple pathways to cancerTrends in Parasitology 28:395–407.https://doi.org/10.1016/j.pt.2012.07.006
-
BookGlobal Health Estimates 2016: Disease Burden by Cause, Age, Sex, by Country and by RegionGeneva: WHO.
Article and author information
Author details
Publication history
Copyright
© 2019, McVeigh and Maule
This article is distributed under the terms of the Creative Commons Attribution License, which permits unrestricted use and redistribution provided that the original author and source are credited.
Metrics
-
- 1,990
- views
-
- 217
- downloads
-
- 17
- citations
Views, downloads and citations are aggregated across all versions of this paper published by eLife.
Citations by DOI
-
- 17
- citations for umbrella DOI https://doi.org/10.7554/eLife.44382
Download links
Downloads (link to download the article as PDF)
Open citations (links to open the citations from this article in various online reference manager services)
Cite this article (links to download the citations from this article in formats compatible with various reference manager tools)
Further reading
-
- Microbiology and Infectious Disease
Infection with the food-borne liver fluke Opisthorchis viverrini is the principal risk factor (IARC Working Group on the Evaluation of Carcinogenic Risks to Humans, 2012) for cholangiocarcinoma (CCA) in the Lower Mekong River Basin countries including Thailand, Lao PDR, Vietnam and Cambodia. We exploited this link to explore the role of the secreted growth factor termed liver fluke granulin (Ov-GRN-1) in pre-malignant lesions by undertaking programmed CRISPR/Cas9 knockout of the Ov-GRN-1 gene from the liver fluke genome. Deep sequencing of amplicon libraries from genomic DNA of gene-edited parasites revealed Cas9-catalyzed mutations within Ov-GRN-1. Gene editing resulted in rapid depletion of Ov-GRN-1 transcripts and the encoded Ov-GRN-1 protein. Gene-edited parasites colonized the biliary tract of hamsters and developed into adult flukes, but the infection resulted in reduced pathology as evidenced by attenuated biliary hyperplasia and fibrosis. Not only does this report pioneer programmed gene-editing in parasitic flatworms, but also the striking, clinically-relevant pathophysiological phenotype confirms the role for Ov-GRN-1 in virulence morbidity during opisthorchiasis.