Notch signaling restricts FGF pathway activation in parapineal cells to promote their collective migration
Abstract
Coordinated migration of cell collectives is important during embryonic development and relies on cells integrating multiple mechanical and chemical cues. Recently, we described that focal activation of the FGF pathway promotes the migration of the parapineal in the zebrafish epithalamus. How FGF activity is restricted to leading cells in this system is, however, unclear. Here, we address the role of Notch signaling in modulating FGF activity within the parapineal. While Notch loss-of-function results in an increased number of parapineal cells activating the FGF pathway, global activation of Notch signaling decreases it; both contexts result in defects in parapineal migration and specification. Decreasing or increasing FGF signaling in a Notch loss-of-function context respectively rescues or aggravates parapineal migration defects without affecting parapineal cells specification. We propose that Notch signaling controls the migration of the parapineal through its capacity to restrict FGF pathway activation to a few leading cells.
https://doi.org/10.7554/eLife.46275.001eLife digest
Many animal cells must move through the body from the place they are born to where they are needed, for example, when embryos are developing or wounds are healing. Often cells migrate in groups, which helps them to navigate and co-ordinate more effectively. Cells typically migrate by sensing different signals across large areas, and groups of cells communicate with each other to co-ordinate their migration.
One group of cells that is studied to understand collective cell migration is found in the brain of the zebrafish. These cells are called parapineal cells and, in the developing fish, they move towards the left side of the brain under the influence of a signal called Fgf8. Although all parapineal cells can detect Fgf8, only those at the front of the migrating group respond to the signal. These are the cells that lead the migration. It was previously unclear how the capability of responding to Fgf8 is limited to only a few parapineal cells to ensure they all migrate correctly.
By studying parapineal cell migration, Wei et al. identified a different signaling system called Notch as a regulator of cell migration. When Notch signaling activity is artificially increased in the brain, parapineal cells do not respond to Fgf8 as efficiently as when levels of Notch are normal. Conversely, the number of parapineal cells that respond to Fgf8 increases when Notch signaling is lost. In both cases, migration of parapineal cells is affected. Therefore, Wei et al. showed that changing the balance of Notch signaling in the zebrafish brain modifies the ability of parapineal cells to respond to Fgf8 signal and stops parapineal cells from migrating correctly.
These results provide a general model for how cells migrate as a group. More studies are needed to see if similar mechanisms are involved in other examples of collective cell migration. This model of group migration could be applied to healthy processes such as embryonic development as well as examples of cell migration in illness such as cancer metastasis.
https://doi.org/10.7554/eLife.46275.002Introduction
Coordinated migration of cell collectives is a widespread phenomenon, being seen predominantly during embryonic development but also during tissue repair in adults, for example. The molecular and cellular mechanisms underlying collective cell migration have been studied in vivo in different model organisms (Friedl and Gilmour, 2009; Ochoa-Espinosa and Affolter, 2012; Pocha and Montell, 2014; Theveneau and Mayor, 2013). Recent progress in the analysis of mechanical forces together with the development of in vitro models and in silico modeling have improved our understanding of coordinated cell migration. Such studies have highlighted the variability in mechanisms from one model to another, indicating that collective migration is a highly adaptive and plastic process (Haeger et al., 2015; Theveneau and Linker, 2017).
Members of the FGF family of secreted signals have been implicated in many models of cell migration. For example, FGF signaling is described to promote migration of cell collectives, potentially through chemotaxis (Kadam et al., 2012), through the modulation of cell adhesiveness (Ciruna et al., 1997; McMahon et al., 2010) or by increasing random cell motility (Bénazéraf et al., 2010). In the lateral line primordium, the FGF pathway is required for Notch-dependent formation of neuromast rosettes at the trailing edge of the migrating primordium (Durdu et al., 2014; Kozlovskaja-Gumbrienė et al., 2017; Lecaudey et al., 2008; Nechiporuk and Raible, 2008) and for a leading-to-trailing signaling that prevents splitting of the primordium (Dalle Nogare et al., 2014), with both of these processes being required for proper lateral line primordium migration. Despite the widespread and iterative role of the FGF pathway in cell migration models, however, it is not clear how the dynamics of FGF signaling correlate with cell behaviors and how this can be modulated by other signals.
The parapineal is a small group of cells that segregates from the anterior part of the pineal gland at the midline of the zebrafish epithalamus and migrates in an FGF-dependent manner to the left side of the brain (Concha et al., 2000; Duboc et al., 2015; Roussigne et al., 2012). To characterize the dynamics of FGF pathway activation during parapineal migration, we recently analyzed the temporal and spatial activation of a previously described FGF pathway reporter transgene, Tg(dusp6:d2GFP) (Molina et al., 2007; Roussigné et al., 2018). Using this reporter, we showed that the FGF pathway is activated in an Fgf8-dependant manner in only a few parapineal cells located at the migration front and that experimentally activating the FGF pathway in a few parapineal cells restores parapineal migration in fgf8-/- mutant embryos. Taken together, these findings indicate that the restricted activation of FGF signaling in the parapineal promotes the migration of the parapineal cell collective. While the parapineal can receive Fgf8 signals from both sides of the midline, focal pathway activation is primarily detected on the left (Roussigné et al., 2018). This asymmetry in FGF pathway activation requires the TGFβ/Nodal signaling pathway, which is activated on the left side of the epithalamus prior to parapineal migration (Bisgrove et al., 1999; Concha et al., 2000; Liang et al., 2000; Roussigné et al., 2018). Although the Nodal pathway appears to bias the focal activation of FGF signaling to the left, after a significant delay the restriction of FGF activity still occurs in the absence of Nodal activity and the parapineal migrates (Roussigné et al., 2018).
All parapineal cells appear competent to activate the FGF pathway begging the question as to how the activation of the pathway is restricted to only a few cells. In this study, we address whether Notch signaling might modulate the activation of FGF pathway in the parapineal. We show that while loss-of-function of Notch leads to expanded FGF pathway activation in the parapineal, activating the Notch pathway causes a strong reduction in the expression of the FGF reporter transgene, with both contexts leading to defects in parapineal migration. Loss or gain of function for Notch signaling also interferes with the specification of parapineal cell identity; loss-of-function results in a significant increase in the number of gfi1ab and sox1a expressing parapineal cells, whereas gain of Notch activity results in the opposite phenotype. In contrast, the number of parapineal cells expressing tbx2b, a putative marker for parapineal progenitors cells (Snelson et al., 2008), is not affected in either loss or gain of function for Notch. Pharmacological inhibition of Notch pathway activity suggests that the roles of Notch in the specification and migration of parapineal cells can be uncoupled. Finally, a global decrease or an increase in the level of FGF signaling can respectively rescue or aggravate the parapineal migration defect caused by Notch loss-of-function but without affecting the specification of parapineal cells. Our data indicate that the Notch pathway regulates the specification and migration of parapineal cells independently and that the role of Notch signaling in promoting parapineal migration, but not specification, depends on its ability to restrict FGF pathway activation to a few parapineal cells.
Results
The parapineal of mindbomb mutant embryos display expanded FGF pathway activation
In models of cell migration during sprouting of tubular tissues, Notch-Delta mediated cell-cell communication contributes to tip cell selection by restricting the ability of followers cells to activate RTK signaling (Ghabrial and Krasnow, 2006; Ikeya and Hayashi, 1999; Riahi et al., 2015; Siekmann and Lawson, 2007a). To address whether Notch signaling could similarly restrict FGF pathway activation in the freely moving group of parapineal cells, and thus promote its migration, we analyzed the expression of an FGF pathway activity reporter transgene, Tg(dusp6:d2EGFP) (Molina et al., 2007), in embryos mutant for the mindbomb (mibta52b) gene, a well-described loss-of-function context for the Notch pathway (Itoh et al., 2003). At 32 hours post-fertilization (hpf), we observed a larger number of Tg(dusp6:d2EGFP) expressing cells in the parapineal of mib-/- mutant embryos (7 ± 3 d2EGFP+ cells) compared to siblings (4 ± 2 d2EGFP+ cells; p-value=3.845e-05) (Figure 1A–1B’ and E). While the number of Tg(dusp6:d2EGFP)+ cells increases, we found that the mean intensity of Tg(dusp6:d2EGFP) does not change significantly in mib-/- mutants indicating that it is the restriction of FGF activation to few parapineal cells rather than the level of FGF activity that is affected in mib-/- mutants (Figure 1—figure supplement 1). The increase in Tg(dusp6:d2EGFP) expressing cells was not accompanied by an increase in the number of parapineal cells expressing sox1a, the earliest described parapineal specific marker (detected from 28 hpf in the parapineal, Clanton et al., 2013) (Figure 1C–1D and G), although we observed a slight increase in the total number of parapineal cells as determined using nuclear staining to visualize the parapineal rosette (22 ± 7 in mib-/- mutants compared to 19 ± 5 in sibling control embryos; p-value=0.037) (Figure 1F).
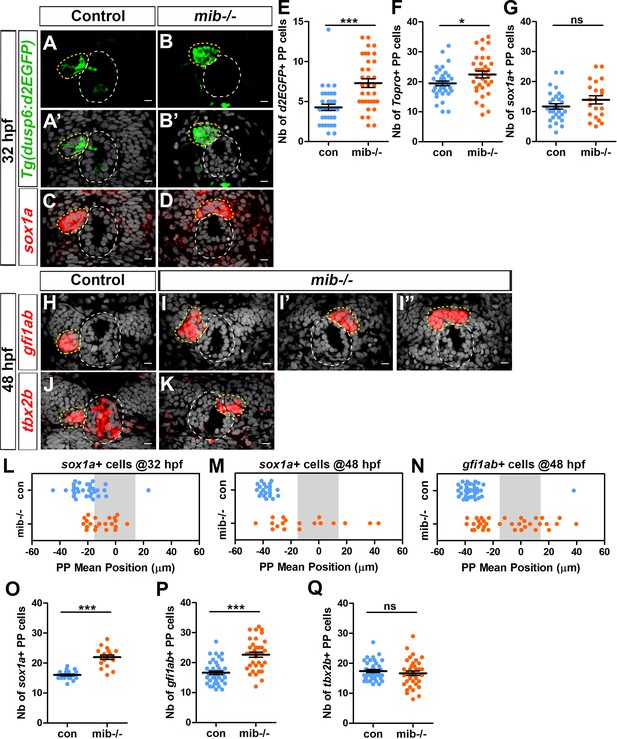
Increased activation of FGF signaling in mindbomb mutants correlates with defects in migration and specification of parapineal cells.
(A–D) Confocal sections showing the expression of the Tg(dusp6:d2EGFP) transgene (Green, (A–B’) or sox1a (red, (C–D) in the epithalamia of 32 hpf in control (A, A’, n = 36 and C, n = 30) and in mib-/- mutant embryos (B, B’, n = 34 and D, n = 21). Confocal sections are merged with a nuclear staining (gray, A’, B’, C, D) that makes the epiphysis (white circle) and parapineal (yellow circle) visible. Embryo view is dorsal, anterior is up; scale bar = 10 µm. (E–G) Dot plots showing the number (E–G) of Tg(dusp6:d2EGFP) (E), Topro-3 (F) and sox1a (G) positive parapineal cells in control (blue dots) or mib-/- mutant embryos (orange dots) at 32 hpf, with mean ± SEM. Tg(dusp6:d2EGFP) FGF reporter is expressed in more parapineal cells in mib-/- than controls (E; 7 ± 3 d2EGFP + cells in mib-/- mutant compared 4 ± 2 in siblings; p-value=3.845e-05, Welch t-test) while the expression of sox1a is similar in both contexts (G); the average number of parapineal cells counted with a nuclear marker increases slightly (F; 22 ± 7 in mib-/- mutants versus 19 ± 5 in sibling control embryos; p-value=0.037, Welch t-test). (H–I’’) Confocal sections showing the expression of gfi1ab (red) at 48 hpf in control embryos (H; n = 40) and in three examples of mib-/- mutant embryos (I-I’’; n = 35). (J–K) Confocal sections showing the expression of tbx2b (red) at 48 hpf in control embryos (J; n = 39) and in one example of mib-/- mutants embryo with the parapineal on the right (K; n = 36). (L–N) Dot plots showing the mean position of parapineal cells expressing sox1a at 32 hpf (L) sox1a at 48 hpf (M) or gfi1ab (N) (in µm distance to the brain midline (x = 0)) with each dot representing an embryo. Parapineal migration is usually delayed in mib-/- mutants at 32 hpf (L). At 48 hpf, the parapineal of mib-/- mutant embryos either did not migrate (n = 12/35 with a parapineal mean position between −15 µM and +15 µM (shaded zone) relative to brain midline (Reference 0)) or migrates either to the left (n = 17/35) or to the right (n = 6/35) (N, orange dots), while it usually migrated to the left in control embryos (N, n = 39/40, blue dots); p-value<0.0001, Welch t-test on absolute values. (O–Q) Number of parapineal cells expressing sox1a (O), gfi1ab (P) and tbx2b (Q) at 48 hpf in control (blue dots) or in mib-/- mutant embryos (orange dots). The number of sox1a and gfi1ab+ positive parapineal cells at 48 hpf is increased in mib-/- mutant embryos (p-value<0.0001 in Welch t-test) compared with controls (O, P) while the number of tbx2b expressing cells is unchanged (Q). Mean ± SEM are indicated as long and short bars. *** p-value<0.0001; * p-value<0.05 in Welch t-test. Data are representative of three experiments (H–I’’, N, P) or two independent experiments (A–G, J–M, O, Q). See also Figure 1—figure supplement 1, Figure 1—figure supplement 2 and Figure 1—figure supplement 3. Source files used for dot plots and statistical analysis are available in Figure 1—source data 1.
-
Figure 1—source data 1
Source files for data used to generate dot plots in Figure 1.
- https://doi.org/10.7554/eLife.46275.007
To confirm that the phenotypes observed in mib-/- mutants are caused by Notch pathway loss-of-function, we analyzed the parapineal in embryos injected with a morpholino (MO; antisense oligonucleotide) blocking translation of the two zebrafish orthologs of su(H)/rbpj (Echeverri and Oates, 2007); Rpbj proteins are transcription factors required for canonical Notch signaling (Fortini and Artavanis-Tsakonas, 1994; Hsieh et al., 1996). As observed in mib-/- mutants, the proportion of Tg(dusp6:d2EGFP) expressing cells was increased in the parapineal of embryos injected with rbpja/b MO (4 ng) (10 ± 6 d2EGFP+ cells) compared to controls (6 ± 4 d2EGFP+ cells; p-value=0,0016) (Figure 1—figure supplement 2, C). Altogether, our results indicate that the FGF signaling pathway is activated in more parapineal cells when Notch signaling is abrogated.
Components of the Notch pathway are expressed in parapineal cells
To address whether the Notch pathway is active in the parapineal, we checked the expression of a well-characterized Notch reporter transgene, Tg(Tp1bglob:EGFP) (Corallo et al., 2013; Parsons et al., 2009), in the epithalamus at 30 hpf. Although rare, cells expressing GFP could be detected in the parapineal at 30 hpf (n = 3/19; Figure 1—figure supplement 3, A–B’).
In parallel, we analyzed the expression of genes encoding Notch ligands. We found that deltaB is expressed in one or two parapineal cells at both 28 hpf (n = 6/12) and 32 hpf (n = 17/23) (Figure 1—figure supplement 3, C–D’); no expression could be detected in the parapineal of the remaining embryos (n = 6/12 at 28 hpf or n = 6/23 at 32 hpf), suggesting that deltaB expression is highly dynamic. We found that deltaA mRNA could also be detected at 32 hpf in 1–2 parapineal cell, although its expression is less robust than deltaB (n = 3/10) (not shown). When detected in the parapineal, deltaB expression was found to overlap with Tg(dusp6:d2EGFP) expression in between half (n = 3/6 at 28 hpf) and two thirds of the embryos (n = 11/17 at 32 hpf).
Therefore, although the expression of Tg(Tp1:EGFP) Notch reporter transgene is not robust in the parapineal, the mosaic expression of deltaA and deltaB ligands in a few parapineal cells supports the existence of Notch signaling activity within the parapineal.
Loss of Notch signaling results in defects in parapineal migration
Interestingly, while in controls the parapineal had usually initiated migration at 32 hpf, it was still detected at the midline in most stage-matched mib-/- mutant embryos (Figure 1A–D and L). To address the effect of Notch inhibition on parapineal migration further, we examined mib-/- mutant embryos at a later stage, when the parapineal had unambiguously migrated in all controls. Analyzing sox1a (Clanton et al., 2013) expression at 2 days post-fertilization revealed that the parapineal failed to migrate in about a third of mib-/- embryos (defined by parapineal mean position within −15 µm and +15 µm of the midline (gray-shaded zone)) (Figure 1M). We confirmed this result by using another marker, gfi1ab (Dufourcq et al., 2004), whose expression is detected at a later stage (from 36 to 40 hpf) than sox1a (from 28 hpf) in parapineal cells. Using gfi1ab, we found that the parapineal had not migrated in 35% of mib-/- embryos (n = 12 of 35 embryos; Figure 1I” and N), while it migrated to the left in more than 95% of control embryos (n = 39/40, Figure 1H and N p-value<0.0001); the parapineal in mib-/- mutant embryos also migrated to the right more often than in controls (17%, n = 6/35; Figure 1I’ and N).
In embryos injected with rbpja/b MO (4 ng), the mean position of parapineal cells at 32 hpf was closer to the midline, suggesting a similar defect in parapineal migration as observed in mib-/- mutant embryos (Figure 1—figure supplement 2, D). Using gfi1ab as a marker at 48 hpf, we found that both parapineal migration per se and the orientation of migration were significantly affected in rbpja/b morphants (Figure 1—figure supplement 2, A–B’, E). While the parapineal migrated to the left in most uninjected controls (94%, n = 31/33), in rbpja/b morphants migration was blocked (13% of the embryos, n = 6/46; p-value=0.0001) or its orientation was partially randomized (left in 67% of the embryos, n = 31/46; right in 20% of the embryos, n = 9/46; p-value=0.0002) (Figure 1—figure supplement 2, A–B’, E).
Taken together, our data show that loss of Notch signaling results in defects both in parapineal migration per se (distance from the midline) and in the laterality of migration (left orientation), and that this correlates with the FGF signaling pathway being activated in more parapineal cells.
Loss of Notch signaling results in an increase in the number of certain parapineal cell subtypes
While quantifying parapineal mean position at 48 hpf, we observed an overall increased in the number of sox1a expressing cells in mib-/- mutants at this stage (Figure 1O) despite finding no significant change at 32 hpf (Figure 1G). Similarly, we found that the number of gfi1ab-positive cells at 48 hpf was significantly increased in mib-/- mutants (23 ± 5) compared to control embryos (17 ± 4; p-value=3.901e-07) (Figure 1H–1I’’ and P). In embryos injected with 4 ng of rbpj a/b MO, we also observed that the number of gfi1ab expressing parapineal cells at 48 hpf was significantly increased (19 ± 6) compared to control embryos (14 ± 3; p-value<0.0001) (Figure 1—figure supplement 2, A–B’, F). In contrast, the number of parapineal cells expressing tbx2b, a parapineal marker previously suggested to be required for the specification of parapineal cells (Snelson et al., 2008), was not increased in mib-/- mutants (Figure 1J–1K and Q).
Taken together, our data show that loss of Notch signaling results in defects in parapineal migration and, at later stages, an increase in the number of gfi1ab and sox1a expressing parapineal cells (putative differentiated parapineal cells) while the number of tbx2b expressing cells (putative parapineal progenitors) is unchanged.
The roles of Notch signaling in the specification and migration of parapineal cells can be uncoupled
Our data show that blocking Notch signaling leads to an expansion of FGF pathway activation in the parapineal, defects in parapineal migration and laterality, and an increase in the number of gfi1ab and sox1a expressing parapineal cells. With the aim of unraveling potential causative links between these different phenotypes, we used a pharmacological inhibitor of the γ-secretase complex, to block the Notch signaling pathway during different time windows (Romero-Carvajal et al., 2015; Rothenaigner et al., 2011); γ-secretase activity is required for the release of the intracellular domain of Notch, NICD, during activation of the canonical pathway (Geling et al., 2002).
We first treated wild-type embryos with LY411575 between 22 and 32 hpf, a time window corresponding to parapineal segregation from the pineal gland and the onset of its migration. While no change in gfi1ab expression was detected in embryos treated with 30 µM LY411575 (Figure 2C), a higher concentration of LY411575 (100 µM) resulted in an increase in the number of gfi1ab-positive cells in treated embryos (Figure 2A–2C); neither treatment resulted in defects in parapineal migration (Figure 2D). As this effect of LY411575 treatment was modest, we next treated embryos heterozygous for mib mutation (mib+/-) during the same time window thinking that this might provide a sensitized background for the drug. mib+/- embryos treated with the lower dose of LY411575 show a strong increase in the number of gfi1ab expressing parapineal cells (28 ± 5) compared to LY411575 treated wild-type controls (17 ± 3; p-value=1.0e-10) or DMSO-treated mib+/- (20 ± 2; p-value=2.2e-10) (Figure 2E–2H and I). As before, however, we did not detect a parapineal migration defect in LY411575-treated mib+/- (Figure 2E–2H and J), even when we increased the dose of LY411575 to 200 µM (Figure 2—figure supplement 1). Therefore, although LY411575 treatment from 22 to 32hpf can synergize with a mib+/- genetic background to promote an increase in the number of gfi1ab-positive parapineal cells, it does not affect parapineal migration. These data indicate that the role of Notch in controlling the specification of parapineal cells can be uncoupled from its function in parapineal migration.
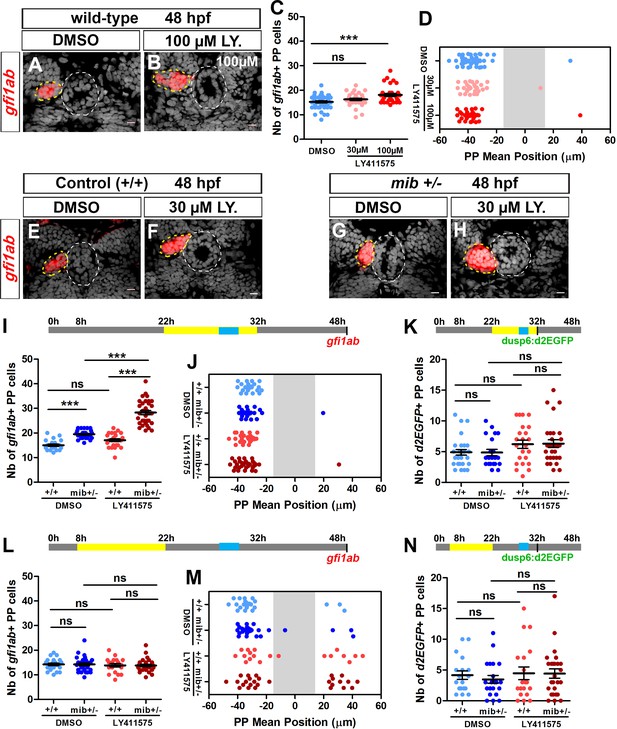
Uncoupled roles for the Notch pathway in the specification, laterality and migration of parapineal cells.
(A–B) Confocal sections showing the expression of gfi1ab (red) in embryos treated with DMSO (A; n = 24) or 100 µM LY411575 (B; n = 32) from 22 to 32 hpf and fixed at 48 hpf, merged with nuclear staining (gray). Embryo view is dorsal, anterior is up; epiphysis (white circle) and parapineal (yellow circle); scale bar = 10 µm. (C–D) Dot plots showing the number (C) and the mean position (D) of gfi1ab expressing parapineal (PP) cells at 48 hpf in embryos treated with DMSO (controls, blue dots, n = 47), with 30 µM LY411575 (light red dots, n = 31) or 100 µM LY411575 (red dots, n = 32) from 22 hpf to 32 hpf, with mean ± SEM; *** p-value=0.0003. (E–H) Confocal sections showing the expression of gfi1ab (red) merged with nuclear staining (gray) at 48 hpf, in wild-type (+/+) (E, F) or mib+/- embryos (G, H) treated with DMSO (E, n = 23 or G, n = 26) or with LY411575 (F, n = 25 and H, n = 34) from 22 to 32 hpf. (I–N) Upper panels show a schematic of the LY411575 treatment timeline (yellow box, 22 to 32 hpf for I-K or 8 to 22 hfp for dot plots L-N), and the time window corresponding to when the parapineal initiates its migration (blue box, 28 to 30 hpf). Dot plots showing the number (I, L) and the mean position (J, M) of gfi1ab expressing cells at 48 hpf, or the number of Tg(dusp6:d2EGFP) expressing cells at 32 hpf (K, N), in the parapineal of DMSO-treated wild-type (+/+, light blue dots; I-J, n = 23; L-M, n = 12; K, n = 28; N, n = 18), DMSO-treated mib+/- heterozygote (dark blue dots; I-J, ± = 26; L-M, n = 17; K, n = 21; N, n = 21), LY411575-treated wild-type ( light red dots; I-J, n = 25; L-M, n = 11; K, n = 23, N, n = 19) and LY411575-treated mib+/- (dark red dots, I-J, n = 34 or L-M, n = 16; K, n = 29, N, n = 26); each dot represents a single embryo. Mean ± SEM is shown in I, K, L, N; *** p-value<0.0001, in Wilcoxon test and Welch t-test. In J and M, there is no defect in migration per se (ns p-value in Welch t-test on absolute value) but LY411575 treatment from 8 to 22 hpf (M) triggers a laterality defect (increased number of embryos with a parapineal on the right). Data are representative of three (E–H, I–K) or two experiments (A–D and L–N). See also Figure 2—figure supplement 1, Figure 2—figure supplement 2 and Figure 2—figure supplement 3. Source files used for dot plots and statistical analysis are available in Figure 2—source data 1.
-
Figure 2—source data 1
Source files for data used to generate dot plots in Figure 2.
- https://doi.org/10.7554/eLife.46275.012
Finally, we saw no significant increase in the number of Tg(dusp6:d2EGFP) positive cells in mib+/- embryos treated with LY411575 during the 22 to 32 hpf time window (Figure 2K). This wild-type level of FGF pathway activation correlates with correct migration of the parapineal in LY411575-treated embryos and is consistent with our previous results suggesting that restricted FGF pathway activation is important for correct parapineal migration (Roussigné et al., 2018).
As the parapineal has usually started to migrate by 32 hpf, it is unlikely that the full parapineal migration defects observed in some mib-/- mutants is a consequence of a role of the Notch pathway after this stage; Notch signaling could nevertheless contribute to migration after 32 hpf. To address this possibility, we treated embryos with LY411575 during a later (32–36 hpf) or extended time window (22–48 hpf). We did not observe any effect of these treatments on the migration as assessed by gfi1ab expression at 48 hpf (Figure 2—figure supplement 2, A,B). Likewise, we did not observe changes in the number of gfi1ab+ cells upon these late treatments (Figure 2—figure supplement 2, C,D).
Notch activity is required early for unilateral activation of the Nodal pathway in the epithalamus
To determine whether the parapineal migration defects we observed in mib-/- mutant embryos and rbpja/b morphants might be an indirect consequence of an earlier role of Notch signaling, we also analyzed the epithalamus of embryos treated with LY411575 during an earlier 8 to 22 hpf time window. This early drug treatment did not interfere with the number of gfi1ab-positive cells (Figure 2L), or with migration in itself (Figure 2M), but led to a partial randomization of the direction of parapineal migration as shown by a significant increase in the number of embryos with a right parapineal (Figure 2M).
We hypothesized that the partial randomization of parapineal sidedness observed in mib-/- mutants, rbpja/b morphants or in embryos treated with LY411575 from 8 to 22 hpf could be caused by changes in the activation pattern of Nodal signaling in the epithalamus (Concha et al., 2000; Liang et al., 2000; Regan et al., 2009). To address this possibility, we analyzed the expression of pitx2c, a Nodal signaling target gene (Concha et al., 2000; Essner et al., 2000; Liang et al., 2000), in the different contexts of Notch loss-of-function. While pitx2c expression is detected in the left epithalamus in control embryos between 28 and 32 hpf (n = 26/28), we observed that its expression is bilateral in most mib-/- mutant embryos (n = 26/29) (Figure 2—figure supplement 3, A–C,D), in a majority of rbpja/b morphants (n = 13/23, Figure 2—figure supplement 3, E) and in approximately half of the embryos treated with LY411575 from 8 to 22 hpf, regardless of whether they were heterozygotes for the mib mutation (mib+/-, n = 6/10) or not (n = 4/13) (Figure 2—figure supplement 3, G). In contrast, the expression of pitx2c was indistinguishable from controls in embryos treated with LY411575 during the later time window (22–32 hpf, Figure 2—figure supplement 3, H) or in embryos expressing NICD from 26 hpf (Figure 2—figure supplement 3, F), which was expected given the absence of laterality defects in these contexts.
Despite triggering defects in parapineal laterality, the early time window of LY411575 treatment (8 to 22 hpf) did not affect parapineal migration per se and, as observed for the late time window (22–32 hpf), this correlates with no significant change in the Tg(dusp6:d2EGFP) expression pattern (Figure 2N). Our data indicate that the partial randomization of parapineal migration observed in mib-/- mutants or rbpj morphants is due to an early role of Notch pathway in restricting Nodal signaling to the left epithalamus and not to changes in the pattern of FGF activation.
Notch gain of function inhibits FGF pathway activation in the parapineal, and blocks the specification and migration of parapineal cells
When we abrogated Notch signaling, we observed an increase in the number of Tg(dusp6:d2EGFP)+ parapineal cells and correlated defects in parapineal migration (Figure 1E and L–M, Figure 1—figure supplement 2, C–E). To address further the role of the Notch pathway in modulating FGF activation, we analyzed the phenotypes associated with global activation of Notch signaling. For this, we used previously described transgenic lines, Tg(hsp70:gal4) and Tg(UAS:NICD-myc) (Scheer and Campos-Ortega, 1999), to induce widespread expression of the Notch Intracellular Domain (NICD) upon heat shock. In most embryos globally expressing NICD from 26 hpf, we observed a strong decrease in the number of Tg(dusp6:d2EGFP) expressing parapineal cells at 36 hpf (2.5 ± 2 cells) compared with the control embryos (5 ± 4; p-value=0.01) (Figure 3A–3B and G); the mean intensity of d2EGFP fluorescence was also significantly decreased in these embryos compared with the controls (p-value=0.0001) (Figure 3A–3B and H).
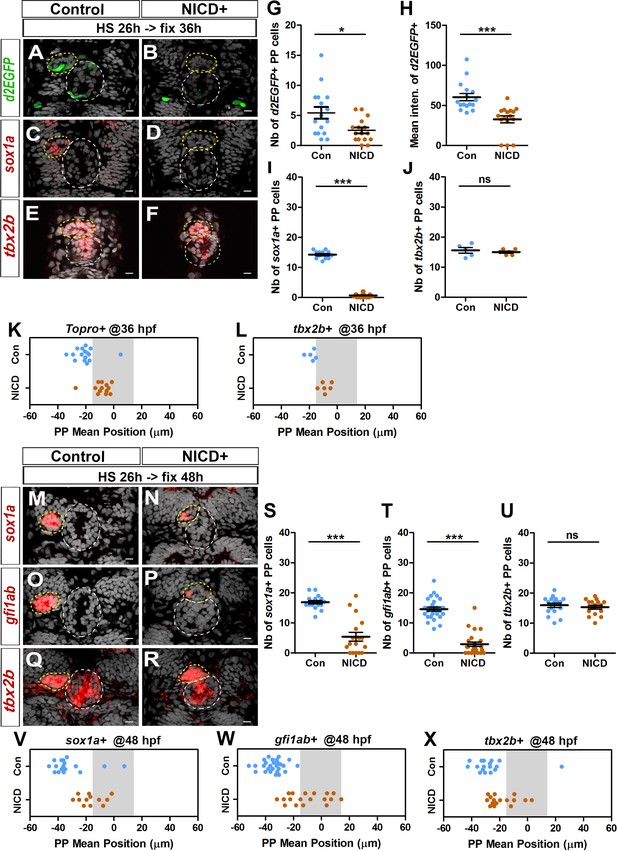
Ectopic Notch signaling triggers decreased FGF activation and defects in migration and specification of parapineal cells.
(A–F) Confocal sections showing the expression of Tg(dusp6 :d2EGFP) (A-B, green), sox1a (C-D, red) or tbx2b (E-F, red) at 36 hpf, in control embryos (A, n = 16; C, n = 12; E, n = 5) or in Tg(hsp70l:Gal4), Tg(UAS:myc-notch1a-intra) embryos (B, n = 16; D, n = 17; F, n = 6) following a heat-shock (HS) at 26 hpf; sections are merged with nuclei staining (gray). (G–L) Dot plots showing the number (G) and the mean intensity fluorescence (H) of Tg(dusp6 :d2EGFP) expressing parapineal cells, the number of sox1a (I) and tbx2b (J) expressing parapineal cells, or the mean position of parapineal cells highlighted by Topro-3 nuclei staining (K) and tbx2b + parapineal cells (L) in controls (blue dots) or in NICD expressing embryos (orange dots) at 36 hpf following heat shock at 26 hpf. (M–R) Confocal sections showing the expression of sox1a (M–N), gfi1ab (O–P) or tbx2b (Q–R) (red) merged with nuclei staining (gray), at 48 hpf, in the epithalamia of control (M, n = 17; O, n = 27; Q, n = 17) or Tg(hsp70l:Gal4);Tg(UAS:myc-notch1a-intra) double transgenic embryos (N, n = 17; P, n = 25; R, n = 16), following heat-shock (HS) at 26 hpf. The expression of sox1a and gfi1ab is lost or decreased while tbx2b expression is unchanged in the parapineal of NICD expressing embryos. (S–X) Dot plots showing the number of sox1a (S), gfi1ab (T) and tbx2b (U) expressing parapineal cells at 48 hpf in controls (blue dots) or in embryos expressing NICD after heat shock at 26 hpf (orange dots) and the corresponding mean position of the cells (V–X) when expression was detected (number of sox1a + or gfi1ab+ cells > 0). In confocal sections, embryo view is dorsal, anterior is up; epiphysis (white circle) and parapineal gland (yellow circle); scale bar = 10 µm. Mean ± SEM is indicated on dot plots G-J and S-U; *** p-value<0.0001, * p-value<0.05 in Welch t-test and Wilcoxon test. For migration dot plots, p-value<0.01 (L) or p-value<0.001 (K and V–X) in pairwise Wilcoxon test and Welch t-test on absolute values. Data are representative of three (O, P, T, W) or two experiments (A–D, G–I, M–N, Q–R, S, U, V, X); data based on tbx2b expression at 36 hpf (E–F, J, L) represents one experiment. See also Figure 3—figure supplement 1. Source files used to generate dot plots and for statistical analysis are available in Figure 3—source data 1.
-
Figure 3—source data 1
Source files for data used to generate dot plots in Figure 3.
- https://doi.org/10.7554/eLife.46275.015
To assess a potential correlation between the inhibition of FGF pathway activation and defects in parapineal migration, we sought to analyze the mean position of sox1a or gfi1ab expressing cells at 36 or 48 hpf as we had done for Notch loss-of-function embryos. However, following heat shock at 26 hpf, sox1a expression was lost in most 36 hpf embryos (Figure 3C–D and I) and was strongly decreased at 48 hpf (Figure 3M–3N and S). Similarly, although the number of gfi1ab positive cells did not vary significantly in Tg(hsp70:gal4); Tg(UAS:NICD-myc) embryos heat shocked at 22 and 24 hpf (Figure 3—figure supplement 1, A–D,I–J), it was strongly decreased in embryos expressing NICD beginning from 26, 28 and 32 hpf (Figure 3O–P and T and Figure 3—figure supplement 1E–H,K–L); in embryos heat shocked at 26 hpf or 28 hpf, gfi1ab staining was often completely lost in the parapineal (n = 7/25 or n = 8/26, respectively) or detected in less than 4 cells (n = 11/25 or n = 14/26, respectively) (Figure 3O–P and T and Figure 3—figure supplement 1, E–F,K). However, nuclear staining indicated that the parapineal rosettes can be detected in most of the embryos expressing NICD (Figure 3A–F and M–R, yellow circle), suggesting that the parapineal does form despite global activation of Notch. Moreover, tbx2b expression was not affected in NICD expressing embryos either at 36 hpf (Figure 3E–F and J) or at 48 hpf (Figure 3Q–R and U). This result suggests that global Notch activation inhibits the specification/differentiation of gfi1ab+ or sox1a+ cells from tbx2b+ progenitors.
As NICD expression led to a loss of Tg(dusp6:d2EGFP) and sox1a expression, we first relied on nuclear staining to assess parapineal cells mean position in this context at 36 hpf. We found that the parapineal was closer to the midline in embryos expressing NICD from 26 hpf, (−8.7 ± 6.3 µM; n = 16) compared to control embryos (−20.5 ± 8.3 µM; n = 16; p-value=0.001) (Figure 3A–F and K). This migration defect was also revealed by analyzing the mean position of tbx2b expressing parapineal cells at 36 hpf (p-value=0.0017) (Figure 3L). Defects in parapineal migration were confirmed at 48 hpf using sox1a, gfi1ab and tbx2b as markers to assess PP mean position (Figure 3M–R and V–X); for instance, when gfi1ab-positive cells were detected, their mean position was significantly closer to the midline in embryos expressing NICD from 26 hpf (−10.1 ± 14 µM; n = 18) compared to control embryos (−34.6 ± 7.5 µM; n = 27) (p-value<0.0001) (Figure 3O–P and W). Parapineal mean position was also affected in embryos with NICD induced just before parapineal formation (heat shock at 22 and 24 hpf), or after parapineal formation (heat shock at 28 and 32 hpf), although in the latter case, the penetrance varies (Figure 3—figure supplement 1, A–H,M–P).
Altogether, our data show that global activation of Notch signaling inhibits the migration of parapineal cells, and that this is correlated with a decrease in the level of FGF signaling detected in the parapineal. Ectopic Notch signaling also decreases the number of gfi1ab+ and sox1a+ parapineal cells, a phenotype opposite to that observed in Notch loss-of-function contexts.
Decreasing FGF signaling rescues the parapineal migration defects in loss of Notch context while increasing FGF signaling aggravates it
Inhibiting or activating the Notch pathway results in reciprocal effects on FGF pathway activation in the parapineal, as seen by an increase or decrease in the number of Tg(dusp6:d2EGFP) expressing parapineal cells, respectively. Both contexts are also associated with defects in parapineal migration, suggesting that Notch-dependent control of FGF activation in parapineal cells is important for their collective migration. To investigate the link between Notch and FGF signaling further, we analyzed whether the migration phenotype observed in mib-/- mutants could be rescued by decreasing FGF signaling, using a pharmacological inhibitor of the FGF pathway (Mohammadi et al., 1997). We have previously shown that treating wild-type embryos with 10 µM SU5402 interferes with parapineal migration (Regan et al., 2009) and with the expression of the Tg(dusp6:d2EGFP) FGF reporter (Roussigné et al., 2018). Treating embryos with 5 µM SU5402, however, does not affect parapineal migration (Figure 4A–4B and F); the parapineal migrates in all SU5402 treated embryos (n = 31/31) as well as in all DMSO-treated control embryos (n = 32/32) (Figure 4F, purple versus blue dots). Using this suboptimal dose, parapineal migration was partially rescued in mib-/- embryos, with 19% of SU5402-treated mib-/- embryos showing mean parapineal position between −15 and +15 µm (n = 8/41) compared to 52% of DMSO treated mib-/- embryos (n = 16/31) (Figure 4C–4D and F, yellow versus orange dots; p-value=0.01). Thus, decreasing the level of FGF signaling activity can partially restore parapineal migration in a context where FGF activation is expanded supporting the hypothesis that Notch promotes parapineal migration through restricting FGF pathway activation.
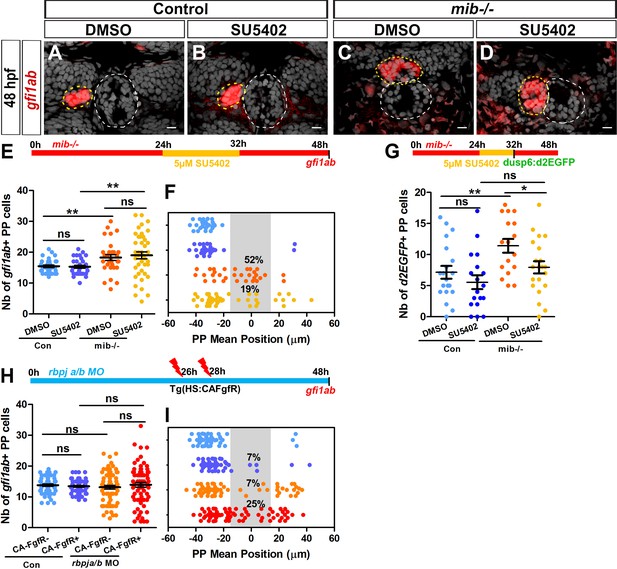
Decreasing or increasing FGF signaling rescues or aggravates the parapineal migration defect in Notch loss-of-function.
(A–D) Confocal sections showing the expression of gfi1ab (red) merged with nuclei staining (gray) at 48 hpf in representative control sibling (A–B) or mib-/- mutant embryos (C–D) treated from 24 to 32 hpf with DMSO (A, C) or 5 µM SU5402 (B, D). Parapineal migration is not affected in controls embryos treated with 5 µM of SU5402 (A–B); in C-D, examples show a parapineal that failed to migrate in a DMSO treated mib-/- mutant embryos (C) or that migrated to the left in a SU5402 treated mib-/- mutant embryos (D). Embryo view is dorsal, anterior is up; epiphysis (white circle) and parapineal (yellow circle); scale bar = 10 µm. (E–F) Upper panel show a schematic of the SU5402 (or DMSO) treatment timeline (24–32 hpf) in control and mib-/- mutant embryos. Dot plots showing the number (E) and the mean position (F) of gfi1ab expressing parapineal (PP) cells at 48 hpf in control embryos treated with DMSO (light blue dots; n = 32) or with 5 µM SU5402 (dark blue dots, n = 31), and in mib-/- mutant embryos treated with DMSO (orange dots, n = 31) or with SU5402 (yellow dots, n = 41). The number of gfi1ab-positive cells is increased in mib-/- mutants embryos, regardless of whether they were treated with SU5402 or DMSO (DMSO control versus DMSO mib-/-, ** p-value=0.0042; SU5402 control versus SU5402 mib-/-, ** p-value=0.0086, Welch t-test). The parapineal fails to migrate in 52% of DMSO treated mib-/- mutant embryos (PP mean position between −15 µm to +15 µm, gray-shaded area), and this proportion decreases to 19% of SU5402 treated mib-/- mutant embryos; p-value=0.0139 on a Chi-square test and p=0.0103 on a Welch t-test on absolute value. (G) Upper panel shows a schematic of the SU5402 (or DMSO) treatment timeline (24–32 hpf) in control and mib-/- mutant embryos. Dot plot showing the number of Tg(dusp6:d2EGFP) expressing parapineal cells at 32 hpf in control embryos treated with DMSO (light blue; n = 20) or with 5 µM SU5402 (dark blue, n = 18), and in mib-/- mutant embryos treated with DMSO (orange, n = 17) or with SU5402 (yellow, n = 21). The number of Tg(dusp6:d2EGFP)+ cells is increased in DMSO treated mib-/- mutants versus controls (p-value=0.0077) but is decreased in SU5402 treated mib-/- mutants compared to DMSO treated mib-/- mutants (p-value=0.0248 in Welch’s t-test). (H–I) Upper panel in H shows a schematic of heat shock timeline in Tg(hsp70:ca-fgfr1) embryos injected with rbpja/b morpholinos (MO). Dot plots showing the number (H) and the mean position (I) of gfi1ab expressing parapineal cells in control embryos that carry (purple dots; n = 55) or do not carry the Tg(hsp70:ca-fgfr1) transgene (light blue dots; n = 54), or in rbpja/bMO injected embryos that carry (dark red dots; n = 73) or do not carry the Tg(hsp70:ca-fgfr1) transgene (orange dots; n = 67). In rbpja/b morphants expressing Tg(hsp70:ca-fgfr1), the parapineal failed to migrate in 25% of embryos (n = 18/73; p-value=0.0007 Welch’s t-test on absolute value and p-value=0.0232 in Chi-square test), a defect significantly higher than expected from merely adding the effects of activated receptor transgene and rbpja/b MO injections alone (p-value=0.0001 in Welch t-test on absolute value and p-value=0.0003 in Chi-square test). Data are representative of four (H–I), three (A–F) or two experiments (G). Source files used to generate dot plots and for statistical analysis are available in Figure 4—source data 1.
-
Figure 4—source data 1
Source files for data used to generate dot plots in Figure 4.
- https://doi.org/10.7554/eLife.46275.017
Suboptimal SU5402 treatment had no effect on parapineal cells specification, either in mib-/- mutant embryos or siblings (Figure 4E). As previously observed, we detected an increase in the number of gfi1ab expressing parapineal cells in DMSO-treated mib-/- embryos (18 ± 5) compared to controls (15 ± 2; p-value=0.0042). The number of gfi1ab positive cells at 48 hpf was also increased in SU5402 treated mib-/- embryos (19 ± 7) compared to SU5402-treated controls (15 ± 3; p-value=0.0086); however, there was no significant difference in the number of gfi1ab expressing cells between DMSO-treated mib-/- embryos and SU5402 treated mib-/- embryos (p-value=0.5687). These data support further that the role of Notch signaling in controling the number of parapineal cells is independent from its function in parapineal migration and does not involve Notch mediated modulation of FGF signaling.
To address the connection between Notch and FGF signaling in an alternative way, we asked whether ectopic activation of the FGF pathway could elicit a more severe phenotype in a loss-of-function context for Notch. To achieve this, we used a transgenic line that expressed a constitutively activated Fgf receptor after heat shock, Tg(hsp70l:Xla.Fgfr1,cryaa:DsRed) (Marques et al., 2008), in embryos injected with rbpja/b MO (4 to 8 ng rbpja/b MO); we chose this Notch context as parapineal migration defects are more modest than in mib-/- mutants. As previously described (Roussigné et al., 2018), widespread expression of constitutively activated receptor (CA-FgfR1) prevented parapineal migration in a small number of embryos (7% of embryos, n = 4/55, with a parapineal mean position between −15 and +15 µm; p-value=0.0011), while the parapineal consistently migrates in heat shocked control embryos not carrying the transgene (n = 54/54 with n = 51/54 leftwards and n = 3/54 toward the right) (Figure 4I). In the absence of the CA-FgfR transgene, rbpja/b morphant embryos displayed a migration defect at low frequency (7%; n = 5/67) (Figure 4I). However, in rbpja/b morphants expressing the activated receptor, the frequency of embryos in which the parapineal failed to migrate increased significantly (25% of embryos; n = 18/73) (Figure 4I, red versus orange and purple dots). Notch and FGF pathways appear to interact in this context as the increase in parapineal migration defects observed in rbpja/b morphant embryos expressing the activated Fgf receptor is significantly higher than expected from adding the effects of activated receptor transgene and rbpja/b MO injections alone (p-value=0.0001 in Welch t test on absolute value and p-value=0.0003 in Chi-square test). The increased frequency of parapineal migration defects occurs in the absence of significant changes in parapineal cell-type specification (Figure 4H); the mean number of gfi1ab expressing parapineal cells did not vary significantly between heat shocked rbpja/b morphant embryos with or without the CA-FgfR transgene (14 ± 6 versus 13 ± 5; p-value=0.39).
Taken together, our loss-of-function, gain-of-function and epistasis experiments argue that the Notch pathway is required for parapineal migration, and that it acts by restricting FGF activation in parapineal cells.
Discussion
In this study, we address how activation of the FGF pathway is restricted within parapineal cells. We show that expression of the Tg(dusp6:d2EGFP) reporter transgene is broader in Notch loss-of-function contexts and is reduced following global activation of the Notch pathway. These changes in FGF activation correlate with defect in parapineal migration. Epistasis experiments show that decreasing or increasing FGF signaling can respectively rescue or aggravate parapineal migration defects in Notch loss-of-function contexts. We conclude that the Notch pathway participates in restricting the activation of FGF signaling to few cells of the parapineal cluster, thus promoting its migration.
Notch effects on parapineal cell specification can be uncoupled from migration
Studies addressing the link between cell specification and migration are rare and, as such, our knowledge of whether and how these two processes are coordinated is limited. In the lateral line primordium (LLP) model, proper morphogenesis of the future neuromast at the trailing edge of the primordium is required for migration (Durdu et al., 2014; Kozlovskaja-Gumbrienė et al., 2017; Lecaudey et al., 2008; Nechiporuk and Raible, 2008). In embryos treated with LY411575 from 22 to 32 hpf, the number of gfi1ab and sox1a expressing parapineal cells is strongly increased but neither parapineal migration nor Tg(dusp6:d2EGFP) expression are affected. Therefore, our data reveals that cell-type specification and migration can be uncoupled in the parapineal. Our results resemble previous observations describing uncoupling of specification and migration of cardiac cells during in heart development (Davidson et al., 2005).
Notch-mediated cell-cell communication is well described for its role on cell fate restriction and progenitors maintenance (Cau and Blader, 2009). In neural tissues or in the pancreas, for instance, inhibition of the Notch signaling pathway causes premature differentiation of the progenitor cells into mature differentiated cells (Li et al., 2015). In the lateral line system, Notch signaling is required to restrict sensory hair cell progenitor fate to a central cell in the forming pro-neuromast (Matsuda and Chitnis, 2010). Similarly, in the parapineal, the number of gfi1ab and sox1a expressing parapineal cells is affected in loss or gain of function for Notch. However, in both contexts, the parapineal rosette is formed and a normal number of tbx2b expressing parapineal cells is detected. Therefore, while in previous studies it was assumed that all described parapineal markers (tbx2b, sox1a, gfi1ab) label the same cells, our data indicate that the pool of tbx2b+ parapineal cells probably differs from a sox1a/gfi1ab+ pool. Our results also suggest that Notch signaling acts downstream of Tbx2b to control the transition from tbx2b expressing putative progenitors to differentiated parapineal cells expressing sox1a/gfi1ab.
Notch acts upstream of FGF signaling to promote parapineal migration
In most models describing cross-talk between the Notch and FGF pathways, Notch signaling is described to act downstream of the FGF pathway. In the zebrafish LLP, for instance, ectopic activation of Notch signaling can rescue the formation of neuromast rosettes in absence of FGF pathway activity, indicating that Notch signaling is required downstream of Fgf signals to promote apical constriction and rosette morphogenesis (Kozlovskaja-Gumbrienė et al., 2017). Notch signaling is also described to be a downstream effector of the FGF pathway for epithelial proliferation in the pancreas in mammalian embryos (Hart et al., 2003). Our epistasis experiments suggest that the Notch pathway acts upstream of FGF signaling in parapineal cells to restrict FGF pathway activation and promote migration. Therefore, although in both the parapineal and the lateral line system, Notch signaling plays a similar role in restricting the number of cells with a particular fate (as mentioned above), the crosstalk with the FGF pathway appears to differ between the two models; Notch signaling acts upstream or downstream of FGF pathway to promote parapineal migration or neuromast morphogenesis, respectively.
Notch signaling restricts activation of the FGF pathway to promote parapineal migration
Our data show that gain or loss of function for Notch respectively result in an increase or decrease in the number of Tg(dusp6:d2EGFP)+ cells in the parapineal, with both correlating with parapineal (PP) migration defects. The fact that the number of Tg(dusp6:d2EGFP)+ cells and not the mean d2GFP intensity of Tg(dusp6:d2EGFP)+ cells is affected in mib-/- mutants strongly suggests that it is the restriction of FGF activation to few parapineal cells that is important for correct migration rather than a specific absolute level of FGF activity in leading cells. These data, together with the fact that partial rescue of migration in SU5402-treated mib-/- mutants correlates with a decrease in the number of Tg(dusp6:d2EGFP)+ cells, indicate that the Notch pathway promotes migration by restricting the activation of FGF signaling to a few parapineal cells.
Notch signaling has previously been implicated in the migration of epithelial cells sheets (Riahi et al., 2015), trachea cells in Drosophila (Ghabrial and Krasnow, 2006; Ikeya and Hayashi, 1999) and in developing vertebrate blood vessels (Siekmann and Lawson, 2007b). In all these models of sheet or sprouting morphogenesis, migrating cells remains attached to the bulk of the tissue. The parapineal is thus the first described model of an isolated cluster of migrating cells in which Notch signaling modulates RTK signaling to define leading cells.
In both the tracheal and vascular systems, Notch-Delta signaling contributes to the selection of the tip cells by restricting the ability of follower cells to activate RTK signaling. The molecular mechanisms underlying Notch mediated restriction of RTK signaling in these two models are not clear but could possibly involve the transcriptional control of RTK ligands or receptors (Ghabrial and Krasnow, 2006; Ikeya and Hayashi, 1999; Siekmann and Lawson, 2007b). As FgfR4, the only Fgf receptor found to be expressed in the parapineal, does not display restricted expression in the parapineal (Regan et al., 2009), it is unlikely that the Notch pathway acts through the control of this receptor.
How Notch signaling could modulate FGF pathway activity in parapineal cells remains an open question. The fact that some rbpja/b morphants display parapineal migration defects similar to mib-/- mutants strongly supports a role for the canonical Notch pathway rather than a Notch-independent role of the Mindbomb ubiquitin ligase in parapineal migration, although we cannot exclude that Mindbomb also regulates migration independently of the Notch pathway, for instance through modulation of Rac1 (Mizoguchi et al., 2017). As all parapineal cells are competent to migrate and to activate the FGF pathway (Concha et al., 2003; Roussigné et al., 2018), a parsimonious mechanism would be that lateral inhibition based cell-cell communication between parapineal cells within the rosette would modulate the capacity of a cell to activate/maintain or to inhibit FGF pathway activity. As described for the C. elegans vulva (Berset et al., 2001; Yoo et al., 2004), for instance, Notch signaling could directly promote the transcription of Ras/MAPK pathway inhibitors. Alternatively, Notch signaling could be required outside the parapineal to trigger mosaic FGF pathway activation in parapineal cells. Finally, there could be both parapineal-intrinsic and extrinsic requirements for Notch. In any case, as the ectopic expression of the constitutive activated FGF receptor (CA-FgfR1) does not completely block parapineal migration in a wild-type background and can rescue migration in fgf8-/- mutants (Roussigné et al., 2018), it appears that the restriction mechanism acts downstream of the receptor rather than at the level of receptor gene expression.
When and where is Notch signaling required?
Given that LY411575 does not block migration in any of the tested time windows, we cannot be sure about the timing of the Notch requirement for migration per se. However, the fact that ectopic Notch signaling at late stages (26–28 hpf) can efficiently block migration argues for a role of Notch at the time when the parapineal initiates its migration and not earlier in development. While expression of the Tg(Tp1:EGFP) Notch reporter transgene is not robustly detected in parapineal cells, the fact that the expression of the Notch ligand deltaB, and occasionally deltaA, can be detected in 1–2 parapineal cells after 28 hpf argues further for a role of Notch signaling within parapineal cells at the time migration starts.
If Notch signaling is indeed required at the time of migration initiation, it is unclear why LY411575 treatment (22 to 32 hpf) does not affect migration while it is able to trigger an increase in the number of gfi1ab and sox1a-positive parapineal cells. Classically, Notch signaling is thought to act through a lateral inhibition mechanism leading to a Notch-ON or Notch-OFF outcome between neighboring cells. Recent work, however, suggests that Notch acts in a level-dependent rather than an all-or-nothing manner (Ninov et al., 2012). In light of this, the differential effect of LY411575 on specification and migration of parapineal cells could reflect a different requirement in Notch signaling threshold, with a lower threshold of Notch signaling being required for migration and a higher one being required to control fate specification of parapineal cells. If LY411575 does not completely block Notch signaling, then residual Notch activity could be sufficient to promote the restriction of FGF activity and parapineal migration while not to limit the specification of gfi1ab and sox1a-positive cells. Consistent with the hypothesis that LY411575 treatment might be partially effective, we observed that the average number of Tg(dusp6:d2EGFP)+ cells increases slightly in LY411575 treated embryos from 22 to 32 hpf, without reaching statistical significance. Given the high variability observed in the number of Tg(dusp6:d2EGFP)+ cells in wild-type contexts, we might expect that parapineal migration is robust enough to tolerate a moderate increase in the number of Tg(dusp6:d2EGFP)+ cells.
Synergistic or parallel role of the Nodal and Notch pathways in restricting FGF pathway activation
The Notch signaling pathway has previously been implicated in regulating the expression of Nodal/TGF-β signal around the node and subsequently in the left lateral plate mesoderm (LPM) (Krebs et al., 2003; Raya et al., 2003). In zebrafish, expression of a nodal related gene (ndr3/southpaw) in the left LPM is required for the later expression of a second nodal gene (ndr2/cyclops) in the left epithalamus, which is required for left-biasing parapineal migration (Concha et al., 2000; Liang et al., 2000; Regan et al., 2009). In mib-/- mutants, rbpj morphant embryos or in embryos treated with LY411575 from 8 to 22 hpf, the Nodal pathway is activated on both sides of the epithalamus. This requirement for Notch signaling in unilateral activation of the Nodal pathway in the epithalamus is consistent with an early role of Notch signaling in establishing the initial left right asymmetry and explains the partial randomization of parapineal migration we observe in contexts of early Notch loss-of-function.
Our previous results indicate that Nodal signaling contributes to restricting FGF pathway activation, as well as biasing it to the left (Roussigné et al., 2018). Here, we show that the restriction of FGF activity requires a functional Notch pathway. As mentioned above, the Nodal pathway is bilateral in the epithalamus of embryos with compromised Notch signaling. However, it is unlikely that the role of Notch in restricting FGF pathway activation depends on its ability to control Nodal signaling. Indeed, in other contexts of bilateral Nodal signaling, such as in embryos injected with morpholinos against notail, we have shown that FGF pathway activation ultimately becomes restricted to a few parapineal cells and parapineal eventually migrates (Roussigné et al., 2018); Tg(dusp6:d2EGFP) reporter expression in this context is no longer left lateralized, which correlates with the parapineal migrating either to the left or the right. In absence of Nodal signaling, as in spaw morphants, Tg(dusp6:d2EGFP) expression is generally less restricted within the parapineal and this correlates with delayed parapineal migration, indicating that the restriction of FGF activity is influenced by Nodal signaling as well as by the Notch pathway. How these two pathways interact to restrict the activation of FGF signaling is not known and future investigations will be needed to address whether Nodal and Notch pathway act in a synergistic way or in parallel to restrict the FGF pathway.
Conclusion
Our study shows that Notch signaling is required for parapineal migration through its capacity to trigger cell state differences in FGF signaling and to restrict FGF activity to a few leading cells. As the function and cross-regulation of the FGF and Notch pathways might be conserved during the migration of invading cancerous cells, our results could help to understand these pathway interaction during metastasis.
Materials and methods
Reagent type (species) or resource | Designation | Source or reference | Identifiers | Additional information |
---|---|---|---|---|
Genetic reagent (D. rerio) | mibta52b | Itoh et al., 2003 | RRID:ZFIN_ZDB-GENO-071218-1 | |
Genetic reagent (D. rerio) | fgf8ti282a (ace) | Reifers et al., 1998 | RRID:ZFIN_ZDB-GENO-980202-822 | |
Genetic reagent (D. rerio) | Tg(hsp70:Gal4)kca4 | Scheer et al., 2001 | RRID:ZFIN_ZDB-ALT-020918-6 | |
Genetic reagent (D. rerio) | Tg(UAS:myc-Notch1a-intra)kca3 | Scheer and Campos-Ortega, 1999 | RRID:ZFIN_ZDB-ALT-020918-8 | |
Genetic reagent (D. rerio) | Tg(hsp70:ca-fgfr1; cryaa:DsRed)pd3 | Marques et al., 2008 Neilson and Friesel, 1996 | RRID:ZFIN_ZDB-GENO-090127-1 | |
Genetic reagent (D. rerio) | Tg(dusp6:d2EGFP)pt6 | Molina et al., 2007 | RRID:ZFIN_ZDB-GENO-071017-5 | |
Genetic reagent (D. rerio) | Tg(Tp1bglob:EGFP)ia12 | Corallo et al., 2013 | RRID:ZFIN_ZDB-ALT-130115-3 | |
Sequence-based reagent | rbpja/su(H)1; rbpjb/su(H)2 | Echeverri and Oates, 2007 | ZFIN ID : ZDB-FISH-150901–13686 | |
Antibody | Rabbit anti-GFP | Torrey Pines Biolabs | TP-401 RRID:AB_10013661 | 1/1000 |
Antibody | Goat anti-rabbit IgG Alexa 488-conjugated | Molecular probe | A11034 | 1/1000 |
Chemical compound, drug | LY411575 | MedChem Express | HY-50752 | Rothenaigner et al., 2011 |
Chemical compound, drug | SU5402 | Calbiochem | 572630 | Mohammadi et al., 1997 |
Other | Fast Red | Sigma Aldrich | F46-48 | |
Other | ToPro-3 | Molecular probe | T3605 | 1/1000 |
Fish lines
Request a detailed protocolEmbryos were raised and staged according to standard protocols (Westerfield, 2000). Embryos homozygous for mindbomb (mibta52b) (Itoh et al., 2003) and fgf8 mutations (fgf8 ti282a/acerebellar/ ace; Reifers et al., 1998) were obtained by inter-crossing heterozygous carriers. Carriers of the fgf8ti282a allele were identified by PCR as described previously (Roussigné et al., 2018). mibta52b+/- carriers were identified by PCR genotyping using primers 5’-GGTGTGTCTGGATCGTCTGAAGAAC-3’ and 5’-GATGGATGTGGTAACACTGATGACTC-3’ followed by enzymatic digestion with NlaIII. Tg(hsp70:Gal4)kca4 and Tg(UAS:myc-Notch1a-intra)kca3 transgenic lines have been described previously (Scheer et al., 2001; Scheer and Campos-Ortega, 1999) and identified by PCR genotyping following ZIRC genotyping protocols. Embryos carrying the Tg(hsp70:ca-fgfr1; cryaa:DsRed)pd3 transgene (Marques et al., 2008; Neilson and Friesel, 1996) were identified by the presence of DsRed expression in the lens from 48 hpf or, by PCR at earlier stages as described previously (Gonzalez-Quevedo et al., 2010). Tg(dusp6:d2EGFP)pt6 (Molina et al., 2007) and Tg(Tp1bglob:EGFP)ia12 (Corallo et al., 2013; Parsons et al., 2009) lines were used as reporters for the FGF and Notch pathways, respectively. Embryos were fixed overnight at 4°C in BT-FIX (Westerfield, 2000), after which they were dehydrated through an ethanol series and stored at −20°C until use.
Ethics statement
Request a detailed protocolFish were handled in a facility certified by the French Ministry of Agriculture (approval number A3155510). The project has received an agreement number APAFIS#3653–2016011512005922. Anaesthesia and euthanasia procedures were performed in Tricaine Methanesulfonate (MS222) solutions as recommended for zebrafish (0.16 mg/ml for anaesthesia, 0.30 mg/ml for euthanasia). All efforts were made to minimize the number of animals used and their suffering, in accordance with the guidelines from the European directive on the protection of animals used for scientific purposes (2010/63/UE) and the guiding principles from the French Decret 2013–118.
LY411575 treatment
Request a detailed protocolEmbryos collected from Tg(dusp6:d2GFP)pt6 carriers outcrossed with wild-type fish or with heterozygous mibta52b were dechorionated and treated from 8 to 22 hpf or 22 to 32 hpf with 30 µM, 100 µM or 200 µM of LY411575 (MedChem; Rothenaigner et al., 2011); control embryos were treated with an equal volume of DMSO diluted in E3 medium. Tg(dusp6:d2GFP) expressing embryos were incubated in LY411575 at 28°C and fixed at 32 hpf for immune-staining against EGFP; sibling embryos, not carrying the Tg(dusp6:d2GFP) transgene, were fixed at indicated time (32 hpf, 36 hpf or 48 hpf) for in situ hybridization against different parapineal markers.
SU5402 drug treatment
Request a detailed protocolEmbryos collected from in-crosses between mibta52b and Tg(dusp6:d2EGFP); mibta52b mutants were dechorionated and treated with 5 µM SU5402 (Calbiochem; Mohammadi et al., 1997) by diluting a 10 mM DMSO based stock solution in E3 medium; control embryos were treated with an equal volume of DMSO diluted in E3 medium. All embryos were incubated in SU5402 at 28°C from 24 hpf to 32 hpf. Tg(dusp6:d2EGFP) embryos were fixed at 32 hpf to analyze the d2EGFP expression pattern in both mibta52b mutants and sibling embryos; the remaining half of embryos were fixed at 48 hpf to analyze parapineal migration.
Morpholino injections
Request a detailed protocolMorpholino oligonucleotides targeting both rbpja/su(H)one and rbpjb/su(H)2 (Echeverri and Oates, 2007) were dissolved in water at 3 mM. The resulting stock solution was diluted to working concentrations (0.3 mM, 2.5 ng/nl) in water and Phenol Red before injection of 1.5 nl (about 4 ng) or 3 nl (8 ng) into embryos at the one-cell stage. Embryos were subsequently fixed and processed for In situ hybridizations or antibody labeling.
Heat shock procedure
Request a detailed protocolEctopic expression of the intracellular domain of Notch receptor (NICD) was induced in Tg(hsp70:gal4); Tg(UAS:myc-Notch1a-intra) double transgenic embryos by incubating them at 39°C for 45 min starting at different time points (22 hpf, 24 hpf, 26 hpf, 28 hpf or 32 hpf). Embryos were then incubated at 28.5°C and fixed at 36 hpf to analyze Tg(dusp6:d2EGFP) expression or at 48 hpf for in situ hybridizations against indicated parapineal markers. Ectopic expression of CA-FgfR1 was induced in Tg(hsp70:ca-FgfR1; cryaa:DsRed)pd3 heterozygote embryos by performing a first heat shock at 25–26 hpf (39°C, 45 min) and a second short heat shock (39°C, 15 min) 3 hours later (28–29 hpf) in order to cover the entire period of parapineal migration. Control embryos are non-transgenic, or carry only Tg(UAS:myc-Notch1a-intra) or Tg(hsp70:Gal4) transgene, and were heat-shocked under the same conditions as the Tg(hsp70:ca-FgfR1) transgenic or Tg(hsp70:gal4); Tg(UAS:myc-Notch1a-intra) double transgenic embryos.
In situ hybridization and immunostaining
Request a detailed protocolIn situ hybridizations were performed as described previously (Roussigné et al., 2018), using antisense DIG labeled probes for gfi1ab (Dufourcq et al., 2004), sox1a (Clanton et al., 2013), pitx2c (Essner et al., 2000), tbx2b (Snelson et al., 2008), deltaA and deltaB (Haddon et al., 1998). In situ hybridizations were completed using Fast Red (from Sigma Aldrich) as an alkaline phosphatase substrate. Immunostainings were performed in PBS containing 0.5% triton using anti-GFP (1/1000, Torrey Pines Biolabs) and Alexa 488-conjugated goat anti-rabbit IgG (1/1000, Molecular Probes). For nuclear staining, embryos were incubated in ToPro-3 (1/1000, Molecular Probes) for 1 hr as previously described (Roussigné et al., 2018).
Image acquisition
Request a detailed protocolConfocal images of fixed embryos were acquired on an upright Leica SP8 confocal microscope using the resonant fast mode and either an oil x63 (aperture 1.4) or x20 (aperture 1.4) objective. Confocal stacks were analyzed using ImageJ software. Figures were prepared using Adobe Photoshop software.
Quantification of the number and position of Tg(dusp6:d2EGFP) positive parapineal cells
Request a detailed protocolThe position and number of parapineal cells positive for the Tg(dusp6:d2GFP) transgene were analyzed using ROI Manager tool on ImageJ software as previously described (Roussigné et al., 2018). The mean intensity of the d2EGFP staining was quantified in an area corresponding to the cell nucleus and the same intensity threshold was used in the different experimental contexts to determine if a cell was Tg(dusp6:d2EGFP) positive or not. The total number of parapineal cells was estimated at 32 hpf by counting nuclei in the parapineal rosettes using Topro-3 nuclear staining.
Quantification of the number and position of gfi1ab, sox1a, tbx2b positive parapineal cells
Request a detailed protocolThe position and number of gfi1ab, sox1a or tbx2b positive parapineal cells were analyzed using the Multipoint tool on ImageJ software and determined as the centre of the cell nucleus detected with the Topro-3 nuclear staining as previously described (Roussigné et al., 2018). The position of each parapineal cell was measured relative to the brain midline (reference origin = 0) as determined by a line passing along the lumen of the epiphysis. For each embryo, we calculated the number of labeled parapineal cells and their mean position. To avoid any bias, data in Figure 4E–F were analyzed blind for DMSO versus SU5402 treatment and data describing LY411575 treatment (Figure 2I–N, Figure 2—figure supplement 1) were analyzed blind for mib genotypes (mib ± heterozygotes versus +/+ controls).
Statistical analysis
Request a detailed protocolStatistical comparisons of datasets were performed using R Studio or GraphPad Prism software. For each dataset, we tested the assumption of normality with Shapiro-Wilks tests and variance homogeneity with F tests. As datasets on the number of parapineal cells were usually normal and often of unequal variances, they were compared using unpaired Welch t-tests; means (± SEM) are indicated as horizontal bars on dot plots. Data on parapineal mean position usually did not distribute normally (because of few embryos with the parapineal on the right) and were compared using the Wilcoxon rank sum non-parametric tests; we also compared parapineal mean position datasets with Welch t-tests using absolute values to discriminate between a defect in laterality (i.e. left-right randomization of parapineal migration) and a defect in migration per se (i.e. distance from the midline only). Most data are representative of at least two and more often three independent experiments and the number of biological replicates is mentioned in the figure legends for each graph. Means (± SD) are indicated in the text.
Data availability
All informations on datasets are provided in the eLife transparent reporting form and all data generated or analysed during this study are included in the manuscript and supporting files. Source data files required to reproduce dot plots and statistical analysis have been provided as an excel file for all main Figures 1-4.
References
-
Regulation of midline development by antagonism of lefty and nodal signalingDevelopment 126:3253–3262.
-
Chimeric analysis of fibroblast growth factor receptor-1 (Fgfr1) function: a role for FGFR1 in morphogenetic movement through the primitive streakDevelopment 124:2829–2841.
-
Asymmetry of the brain: development and implicationsAnnual Review of Genetics 49:647–672.https://doi.org/10.1146/annurev-genet-112414-055322
-
Parapineal specific expression of gfi1 in the zebrafish epithalamusGene Expression Patterns 4:53–57.https://doi.org/10.1016/S1567-133X(03)00148-0
-
Mesendoderm and left-right brain, heart and gut development are differentially regulated by pitx2 isoformsDevelopment 127:1081–1093.
-
Collective cell migration in Morphogenesis, regeneration and CancerNature Reviews Molecular Cell Biology 10:445–457.https://doi.org/10.1038/nrm2720
-
Neuronal regulation of the spatial patterning of neurogenesisDevelopmental Cell 18:136–147.https://doi.org/10.1016/j.devcel.2009.11.010
-
Multiple Delta genes and lateral inhibition in zebrafish primary neurogenesisDevelopment 125:359–370.
-
Collective cell migration: guidance principles and hierarchiesTrends in Cell Biology 25:556–566.https://doi.org/10.1016/j.tcb.2015.06.003
-
Truncated mammalian Notch1 activates CBF1/RBPJk-repressed genes by a mechanism resembling that of Epstein-Barr virus EBNA2Molecular and Cellular Biology 16:952–959.https://doi.org/10.1128/MCB.16.3.952
-
Interplay of notch and FGF signaling restricts cell fate and MAPK activation in the Drosophila TracheaDevelopment 126:4455–4463.
-
Notch signaling regulates left-right asymmetry determination by inducing nodal expressionGenes & Development 17:1207–1212.https://doi.org/10.1101/gad.1084703
-
Notch signaling in pancreatic developmentInternational Journal of Molecular Sciences 17:E48.https://doi.org/10.3390/ijms17010048
-
Asymmetric nodal signaling in the zebrafish diencephalon positions the pineal organDevelopment 127:5101–5112.
-
Ligand-independent activation of fibroblast growth factor receptors by point mutations in the extracellular, transmembrane, and kinase domainsJournal of Biological Chemistry 271:25049–25057.https://doi.org/10.1074/jbc.271.40.25049
-
Branching morphogenesis: from cells to organs and backCold Spring Harbor Perspectives in Biology 4:a008243.https://doi.org/10.1101/cshperspect.a008243
-
Notch-responsive cells initiate the secondary transition in larval zebrafish pancreasMechanisms of Development 126:898–912.https://doi.org/10.1016/j.mod.2009.07.002
-
Fgf8 is mutated in zebrafish acerebellar (ace) mutants and is required for maintenance of midbrain-hindbrain boundary development and somitogenesisDevelopment 125:2381–2395.
-
Breaking symmetry: the zebrafish as a model for understanding left-right asymmetry in the developing brainDevelopmental Neurobiology 72:269–281.https://doi.org/10.1002/dneu.20885
-
An instructive function for notch in promoting gliogenesis in the zebrafish retinaDevelopment 128:1099–1107.
-
Use of the Gal4-UAS technique for targeted gene expression in the zebrafishMechanisms of Development 80:153–158.https://doi.org/10.1016/S0925-4773(98)00209-3
-
Notch signalling and the regulation of angiogenesisCell Adhesion & Migration 1:104–105.https://doi.org/10.4161/cam.1.2.4488
-
Tbx2b is required for the development of the parapineal organDevelopment 135:1693–1702.https://doi.org/10.1242/dev.016576
-
Collective cell migration of epithelial and mesenchymal cellsCellular and Molecular Life Sciences 70:3481–3492.https://doi.org/10.1007/s00018-012-1251-7
-
A Guide for the Laboratory Use of Zebrafish (Danio RerioThe Zebrafish Book, A Guide for the Laboratory Use of Zebrafish (Danio Rerio, Fourth Edition, Eugene, University of Oregon Press.
Article and author information
Author details
Funding
Fondation pour la Recherche Médicale (DEQ20131029166)
- Patrick Blader
Fondation ARC pour la Recherche sur le Cancer (PJA 20131200173)
- Patrick Blader
Agence Nationale de la Recherche (ANR-16-CE13-0013-01)
- Patrick Blader
China Scholarship Council (CSC No.201504910807)
- Lu Wei
Centre National de la Recherche Scientifique
- Myriam Roussigné
Université Toulouse III - Paul Sabatier
- Myriam Roussigné
Inserm
- Patrick Blader
The funders had no role in study design, data collection and interpretation, or the decision to submit the work for publication.
Acknowledgements
We are grateful to all members of the Blader lab, especially Elise Cau and Julie Batut for critical reading of the manuscript and Aurélie Quillien for insightful discussions. We also thank Eric Theveneau and Matthias Carl for critical reading of the manuscript, as well as Xiaobo Wang and Steve W Wilson for their input. We thank Brice Ronsin from the Toulouse RIO Imaging platform and Aurore Laire for fish care. This work was supported by the Centre National de la Recherche Scientifique (CNRS), the Institut National de la Santé et de la Recherche Médicale (INSERM), Université de Toulouse III (UPS), the Fondation pour la Recherche Médicale (FRM; DEQ20131029166), the Fondation ARC (PJA 20131200173), the Agence nationale de la recherche (ANR-16-CE13-0013-01) and the China Scholarship Council (CSC No.201504910807) for PhD funding for Lu Wei.
Ethics
Animal experimentation: Fish were handled in a facility certified by the French Ministry of Agriculture (approval number A3155510). The project has received an agreement number APAFIS#3653-2016011512005922. Anaesthesia and euthanasia procedures were performed in Tricaine Methanesulfonate (MS222) solutions as recommended for zebrafish (0,16mg/ml for anaesthesia, 0,30 mg/ml for euthanasia). All efforts were made to minimize the number of animals used and their suffering, in accordance with the guidelines from the European directive on the protection of animals used for scientific purposes (2010/63/UE) and the guiding principles from the French Decret 2013-118.
Copyright
© 2019, Wei et al.
This article is distributed under the terms of the Creative Commons Attribution License, which permits unrestricted use and redistribution provided that the original author and source are credited.
Metrics
-
- 1,429
- views
-
- 171
- downloads
-
- 6
- citations
Views, downloads and citations are aggregated across all versions of this paper published by eLife.
Citations by DOI
-
- 6
- citations for umbrella DOI https://doi.org/10.7554/eLife.46275