Stem Cells: Getting to the heart of cardiovascular evolution in humans
Cardiovascular disease is one of the leading causes of death in both humans and captive chimpanzees. However, despite having similar heart anatomies, humans and chimpanzees are prone to different types of cardiovascular disease. In humans, a build-up of fatty materials in the arteries (a condition called atherosclerosis) can restrict blood flow to the heart, which can cause myocardial ischemia. On the other hand, great apes, including chimpanzees, are more likely to suffer heart disease from myocardial fibrosis (Lowenstine et al., 2016). This condition, which rarely leads to heart disease in humans, involves increased deposition of collagen in heart tissue (Lammey et al., 2008).
Both genetic and environmental factors could be responsible for these differences. Interestingly, cholesterol is a major risk factor for atherosclerosis in humans, yet captive chimpanzees manage to avoid this condition despite having higher cholesterol levels than humans (Varki et al., 2009). However, a lack of appropriate model systems has made it difficult to investigate how genetic differences in disease susceptibility evolved between these two species.
Recent studies have shown that cardiomyocytes (heart muscle cells derived from stem cells) recapitulate many of the features of normal human and chimpanzee hearts, although there are important differences in metabolism and maturation state (Pavlovic et al., 2018). Since it is possible to grow human and chimpanzee cardiomyocytes under common environmental conditions, this provides an opportunity to study differences in the genetics of the two systems (Gallego Romero et al., 2015). Now, in eLife, Michelle Ward and Yoav Gilad from the University of Chicago report how cardiomyocytes can be used to compare the genomic consequences of myocardial ischemia in humans and chimpanzees (Ward and Gilad, 2019).
A characteristic symptom of myocardial ischemia is oxygen deprivation, also known as hypoxia, which is caused by a reduction in the flow of blood to the heart. To simulate hypoxia, Ward and Gilad first cultured cardiomyocytes in normal oxygen levels, then exposed them to a ten-fold decrease in oxygen for six hours, before returning them to normal oxygen levels. Depriving heart tissue of oxygen typically leads to increased production of reactive oxygen species (such as peroxides) that can cause DNA damage and lipid degradation. Hypoxia induced similar effects in both human and chimpanzee cardiomyocytes, suggesting that such experiments can recapitulate the effects of myocardial ischemia.
RNA sequencing revealed that nearly one third of the genes that are expressed in cardiomyocytes respond to hypoxia. Moreover, 75% of these responded in the same way in both species, suggesting that hypoxia has a widespread and largely conserved effect on gene expression. In contrast, previous work has shown that the immune responses in humans and chimpanzees are quite different (Barreiro et al., 2010).
Next, Ward and Gilad investigated how patterns of gene expression were influenced by hypoxia within these two species. Despite the similarities revealed in the RNA sequencing experiments, several hundred genes still differed in their responses. One possible explanation for these differences is that hypoxia-related transcription factors bind to response genes to varying degrees. Consistent with this possibility, hypoxia-related factors were shown to frequently bind to, or near to, conserved response genes, but not to genes with chimpanzee-specific responses. This suggests that changes in transcription factor binding, and possibly changes to the sites they bind to, may be responsible for the several hundred genes that respond differently to hypoxia in the two species.
Another notable difference is the response of a protein called RASD1, which is upregulated during hypoxia in human cardiomyocytes but not in chimpanzee cardiomyocytes. Interestingly, this gene is also upregulated in patients with myocardial ischemia and other diseases related to atherosclerosis. RASD1 could therefore have a role in regulating hypoxia-induced tissue damage in humans.
Ward and Gilad then explored the role of genes called eQTL genes (where eQTL is short for expression quantitative trait loci). Genetic variation within these genes can explain some of the disparity in mRNA levels among individuals in a population. Intersecting eQTL genes with genetic association studies has become a popular strategy for identifying disease-risk genes (Albert and Kruglyak, 2015). However, several recent lines of evidence suggest that eQTL genes may reflect mostly neutral genetic variation and are not therefore associated with any specific diseases (Jasinska et al., 2017; Tung et al., 2015).
Ward and Gilad found that there was a significant overlap between the conserved hypoxia response genes and the genes that are upregulated in patients with myocardial ischemia. This overlap indicates that the response genes are relevant to disease: however, the overlap between these genes and eQTL genes was lower than expected (Figure 1). Therefore, identifying the genetic variants and genes that influence disease-relevant responses, such as hypoxia, is likely to be more informative than traditional baseline eQTL studies. This means that stem cell models, such as those studied by Ward and Gilad, could be used more generally to study how common variation and evolved genetic differences influence other disease-relevant responses.
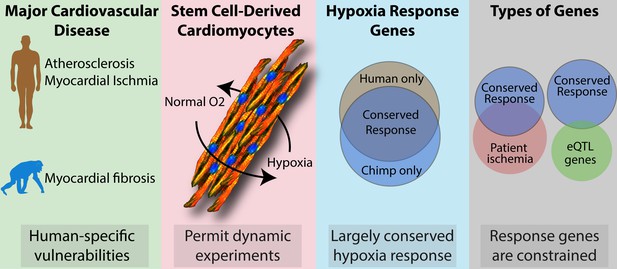
Using stem cell models to explore the genetic effects that influence susceptibility to cardiovascular disease.
Ward and Gilad explored the effects of hypoxia (that is, oxygen deprivation) on cardiomyocytes (heart cells derived from stem cells) from humans and chimpanzees. They found that 75% of the genes that respond to hypoxia respond in the same way in both species (third panel). They also observed a large overlap between these conserved response genes and genes that are upregulated in patients with ischemia (fourth panel; left), but little overlap with eQTL genes (right).
References
-
The role of regulatory variation in complex traits and diseaseNature Reviews Genetics 16:197–212.https://doi.org/10.1038/nrg3891
-
Interstitial myocardial fibrosis in a captive chimpanzee (Pan troglodytes) populationComparative Medicine 58:389–394.
Article and author information
Author details
Publication history
Copyright
© 2019, Pollen and Pavlovic
This article is distributed under the terms of the Creative Commons Attribution License, which permits unrestricted use and redistribution provided that the original author and source are credited.
Metrics
-
- 1,231
- views
-
- 101
- downloads
-
- 2
- citations
Views, downloads and citations are aggregated across all versions of this paper published by eLife.
Download links
Downloads (link to download the article as PDF)
Open citations (links to open the citations from this article in various online reference manager services)
Cite this article (links to download the citations from this article in formats compatible with various reference manager tools)
Further reading
-
- Evolutionary Biology
The majority of highly polymorphic genes are related to immune functions and with over 100 alleles within a population, genes of the major histocompatibility complex (MHC) are the most polymorphic loci in vertebrates. How such extraordinary polymorphism arose and is maintained is controversial. One possibility is heterozygote advantage (HA), which can in principle maintain any number of alleles, but biologically explicit models based on this mechanism have so far failed to reliably predict the coexistence of significantly more than 10 alleles. We here present an eco-evolutionary model showing that evolution can result in the emergence and maintenance of more than 100 alleles under HA if the following two assumptions are fulfilled: first, pathogens are lethal in the absence of an appropriate immune defence; second, the effect of pathogens depends on host condition, with hosts in poorer condition being affected more strongly. Thus, our results show that HA can be a more potent force in explaining the extraordinary polymorphism found at MHC loci than currently recognised.
-
- Ecology
- Evolutionary Biology
Understanding the origins of novel, complex phenotypes is a major goal in evolutionary biology. Poison frogs of the family Dendrobatidae have evolved the novel ability to acquire alkaloids from their diet for chemical defense at least three times. However, taxon sampling for alkaloids has been biased towards colorful species, without similar attention paid to inconspicuous ones that are often assumed to be undefended. As a result, our understanding of how chemical defense evolved in this group is incomplete. Here, we provide new data showing that, in contrast to previous studies, species from each undefended poison frog clade have measurable yet low amounts of alkaloids. We confirm that undefended dendrobatids regularly consume mites and ants, which are known sources of alkaloids. Thus, our data suggest that diet is insufficient to explain the defended phenotype. Our data support the existence of a phenotypic intermediate between toxin consumption and sequestration — passive accumulation — that differs from sequestration in that it involves no derived forms of transport and storage mechanisms yet results in low levels of toxin accumulation. We discuss the concept of passive accumulation and its potential role in the origin of chemical defenses in poison frogs and other toxin-sequestering organisms. In light of ideas from pharmacokinetics, we incorporate new and old data from poison frogs into an evolutionary model that could help explain the origins of acquired chemical defenses in animals and provide insight into the molecular processes that govern the fate of ingested toxins.