Targeting the vascular-specific phosphatase PTPRB protects against retinal ganglion cell loss in a pre-clinical model of glaucoma
Abstract
Elevated intraocular pressure (IOP) due to insufficient aqueous humor outflow through the trabecular meshwork and Schlemm’s canal (SC) is the most important risk factor for glaucoma, a leading cause of blindness worldwide. We previously reported loss of function mutations in the receptor tyrosine kinase TEK or its ligand ANGPT1 cause primary congenital glaucoma in humans and mice due to failure of SC development. Here, we describe a novel approach to enhance canal formation in these animals by deleting a single allele of the gene encoding the phosphatase PTPRB during development. Compared to Tek haploinsufficient mice, which exhibit elevated IOP and loss of retinal ganglion cells, Tek+/-;Ptprb+/- mice have elevated TEK phosphorylation, which allows normal SC development and prevents ocular hypertension and RGC loss. These studies provide evidence that PTPRB is an important regulator of TEK signaling in the aqueous humor outflow pathway and identify a new therapeutic target for treatment of glaucoma.
Introduction
A leading cause of blindness worldwide, glaucoma is a devastating disease with no cure. Elevated intraocular pressure (IOP) caused by defects in the aqueous humor outflow (AHO) pathway is the most important risk factor for disease progression and vision loss (Coleman and Miglior, 2008; Becker, 1961). Indeed, IOP reduction is currently the only therapeutic intervention proven to slow glaucoma progression in patients. In both humans and mice, the majority of AHO occurs through the conventional route (Johnson et al., 2017; Toris et al., 1999; Toris et al., 2000; Millar et al., 2011; Millar et al., 2015), comprised of the trabecular meshwork (TM) and the large, lymphatic-like Schlemm’s canal (SC) located in the iridocorneal angle (Johnson et al., 2017; Park et al., 2014; Aspelund et al., 2014; Kizhatil et al., 2014). Aqueous humor from the anterior chamber enters SC through the TM and is drained through a series of collector channels into the episcleral veins and systemic circulation. Recent studies have identified the importance of endothelial signaling molecules in development and maintenance of SC and the conventional outflow pathway, and critical roles have been described for the endothelial transcription factor PROX1 (Park et al., 2014) as well as the VEGFR2/3 (Kizhatil et al., 2014; Aspelund et al., 2014) and TEK (Thomson et al., 2014; Kim et al., 2017; Souma et al., 2016; Thomson et al., 2017) receptor tyrosine kinase signaling pathways.
TEK (Tunica interna endothelial cell kinase, also known as TIE2) is the tyrosine kinase receptor for the angiopoietin (Angpt) ligands ANGPT1, ANGPT2 and ANGPT4 (Saharinen et al., 2017). The Angpt-TEK pathway is essential for SC development and maintenance, and loss of function mutations in TEK or the gene encoding its primary ligand ANGPT1 have been identified in patients with primary congenital glaucoma, a severe form of glaucoma characterized by early/childhood onset, buphthalmos and optic neuropathy (Souma et al., 2016; Thomson et al., 2017; Kabra et al., 2017). Furthermore, recent genome-wide association studies have identified risk variants linked to the TEK signaling pathway in adults with elevated IOP and open angle glaucoma, the most common form of glaucoma worldwide (Khawaja et al., 2018; Gao et al., 2018; MacGregor et al., 2018).
In mice, post-natal Tek deletion leads to complete failure of SC development and rapidly progressing glaucoma (Thomson et al., 2014; Kim et al., 2017; Souma et al., 2016). A similar disease is observed in mice lacking the TEK ligand ANGPT1, confirming that ANGPT-TEK signaling is essential for canal development (Thomson et al., 2017). Importantly, while Tek knockout mice exhibit complete loss of SC, a hypomorphic canal characterized by focal narrowing and convolutions is observed in haploinsufficient animals (Tek+/- mice) (Souma et al., 2016). This hypomorphic SC is associated with moderate IOP elevation, indicating a clear dose-dependent effect of TEK signaling in development and function of the aqueous outflow pathway and suggesting that TEK activation using genetic or pharmacological approaches might provide novel treatments for patients with high-pressure glaucoma. Indeed, the clear dose-dependent relationship between ANGPT-TEK signaling and severity of disease presentation in rodent models supports the argument that therapeutic modulation of this pathway will be efficacious in patients (Plenge et al., 2013).
Activation of the TEK receptor has been achieved in vitro and in vivo either by increasing activity of endogenous ANGPT ligands, providing ANGPT recombinant proteins (Kim et al., 2005; Souma et al., 2018), or by suppression of the phosphatase PTPRB (also known as the Vascular Endothelial Protein Tyrosine Phosphatase, VE-PTP) (Winderlich et al., 2009; Shen et al., 2014; Carota et al., 2019), which strongly dephosphorylates TEK (Souma et al., 2018; Fachinger et al., 1999). PTPRB inhibition results in ligand-independent increased TEK phosphorylation at all phosphorylated tyrosine residues, and leads to a dramatic increase in downstream signaling (Souma et al., 2018; Carota et al., 2019). Here, we show that developmental deletion of a single Ptprb allele in mice is sufficient to reduce PTPRB expression and leads to increased TEK activation in vivo. Furthermore, in Tek+/- haploinsufficient mice, this increased TEK activation is sufficient for normal SC development, preventing both ocular hypertension and retinal ganglion cell (RGC) loss.
Results
To increase the level of TEK phosphorylation in vivo, we utilized a PtprbNLS-LacZ knock-in reporter allele (Bäumer et al., 2006) to delete a single allele of the Ptprb gene. This construct incorporates a β-Galactosidase cDNA tagged with a nuclear localization signal in place of the first exon of Ptprb, preventing production of PTPRB protein. As previously described, heterozygous PtprbNLS-LacZ/WT mice are born normally (Bäumer et al., 2006), although expression of PTPRB was reduced by approximately 50% (Figure 1A, uncropped images presented as Figure 1—figure supplement 1). Likewise, Tek heterozygosity resulted in approximately 50% reduction in TEK protein detected in lung lysate (Figure 1A). Reductions in phosphatase abundance had a direct effect on TEK activation and PtprbNLS-LacZ/WT mice showed approximately a 118% increase in phosphorylated TEK when measured in lung tissue using an immunoprecipitation assay (Figure 1B, uncropped images presented as Figure 1—figure supplement 2), confirming our hypothesis that changes in PTPRB expression would have a direct impact on TEK phosphorylation.
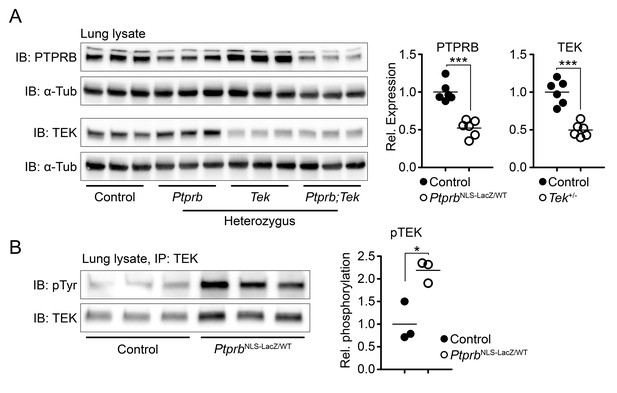
Deletion of one Ptprb allele leads to increased TEK phosphorylation.
(A) Western blot of lung lysate from P5 Control, Tek+/-, PtprbNLS-LacZ/WT and Tek+/-;PtprbNLS-LacZ/WT mice revealed a 50% reduction in PTPRB expression in PtprbNLS-LacZ/WT (heterozygous) and Tek+/-;PtprbNLS-LacZ/WT mice. Likewise, TEK expression was reduced approximately 50% in Tek+/- and Tek+/-;PtprbNLS-LacZ/WT mice. (B) Immunoprecipitation of lung lysates from adult control and PtprbNLS-LacZ/WT using anti-TEK antibody followed by western blotting with anti-phospho tyrosine antibody revealed a marked elevation of TEK phosphorylation in PtprbNLS-LacZ/WT animals compared to littermate controls. Horizontal lines indicate population means. *p<0.05, ***p<0.001 as determined by Student’s t-test.
The finding that PtprbNLS-LacZ/WT haploinsufficient mice exhibited markedly increased TEK phosphorylation suggested that these animals would provide a powerful tool to study the effect of PTPRB blockade on SC development in the context of reduced TEK signaling. Therefore, we crossed mice carrying the PtprbNLS-LacZ allele with a previously-described mouse model of Tek heterozygosity, creating a constitutive model of Tek+/-;PtprbNLS-LacZ/WT double haploinsufficency. Adult Tek haploinsufficient mice have been reported to exhibit a hypomorphic SC insufficient for normal AHO, leading to moderate IOP elevation (Souma et al., 2016). To test our hypothesis that ~50% reduction of PTPRB activity might prevent the glaucoma phenotype in Tek+/- mice without the need to increase ligand availability, Tek+/-;PtprbNLS-LacZ/WT double heterozygous mice were generated, and enucleated eyes were collected. After fixation, eyes were prepared for whole-mount immunostaining and visualized using confocal microscopy. Consistent with previous findings (Souma et al., 2016), analysis of CD31-positive SC area revealed a hypomorphic canal phenotype in adult Tek heterozygous mice when compared to littermate controls (Control: 29,974 ± 2145, Tek+/-: 17,457 ± 1040 μm2/20x field; Figure 2A). This phenotype was abrogated by deletion of a single Ptprb allele in Tek+/-;PtprbNLS-LacZ/WT animals, which exhibited normal SC area (Tek+/-;PtprbNLS-LacZ/WT: 23,848 ± 1574 μm2/20x field). Importantly, despite elevated TEK activation, PtprbNLS-LacZ/WT animals displayed a normal SC (PtprbNLS-LacZ/WT: 28,175 ± 1668 μm2/20x field), suggesting reduction of PTPRB function is well tolerated during SC development in the absence of other mutations.
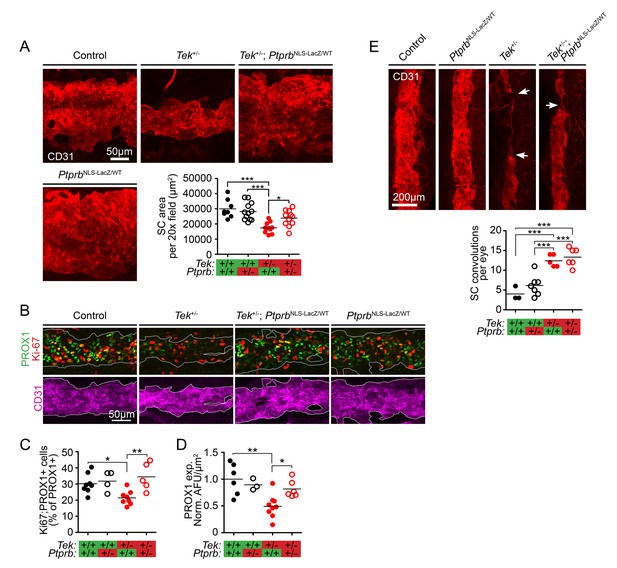
TEK signaling has a dose-dependent effect on Schlemm’s canal (SC) area and development.
(A) Confocal microscopy of whole mount eyes revealed reduced CD31+ SC area in adult Tek+/- haploinsufficient mice. This phenotype was blunted in Tek+/-;PtprbNLS-LacZ/WT double heterozygous animals, confirming the importance of TEK activation in canal development. PtprbNLS-LacZ/WT heterozygous controls had normal SC area (n = 8 WT, 12 PtprbNLS-LacZ/WT, 11 Tek+/- and 11 Tek+/-;PtprbNLS-LacZ/WT mice). 20x fields shown represent an area of 65,536 μm2. Images were captured as 10-frame Z stacks with a step size of 1.67 μm and a pinhole of 1.2 Airy units, and are shown as maximum intensity projections. (B, quantified in C) At postnatal day 5 (P5), confocal microscopy of the developing SC in eye whole mounts revealed reduced numbers of proliferating Ki-67-positive SC ECs (Ki67-PROX1 double positive cells) in Tek haploinsufficient animals compared to littermate WT or PtprbNLS-LacZ/WT controls. Normal proliferation was observed in Tek+/-;PtprbNLS-LacZ/WT animals. (D) Compared to control and PtprbNLS-LacZ/WT mice, PROX1 expression was reduced in Tek+/- littermate eyes. Expression was normal in Tek+/-;PtprbNLS-LacZ/WT double heterozygotes. n = 8 (WT), 4 (PtprbNLS-LacZ/WT), 8 (Tek+/-) and 5 (Tek+/-;PtprbNLS-LacZ/WT) Shown are maximum intensity projections from 8-frame confocal Z stacks captured using a 20x objective, step size of 1 μm and pinhole of 1.2 Airy units. Norm. AFU: Normalized, background subtracted arbitrary fluorescence units. (E) Compared to control and PtprbNLS-LacZ/WT littermates, confocal analysis of adult SC revealed a marked increase in the number of focal convolutions and narrowings in the eyes of Tek+/- and Tek+/-;PtprbNLS-LacZ/WT mice. N = 4 (WT), 7 (PtprbNLS-LacZ/WT), 5 (Tek+/-) and 6 (Tek+/-;PtprbNLS-LacZ/WT). Horizontal lines indicate population means. *p<0.05, **p<0.01, ***p<0.001 as determined by 1-way ANOVA followed by Bonferroni’s correction.
Developmental haploinsufficency of Tek and Ptprb had clear effects on adult SC area in our model. To determine if this phenotype was due to altered proliferation of SC endothelial cells (ECs), we next analyzed eyes collected at P5 when SC development is underway. In Tek+/- eyes, we observed a marked reduction in the proportion of PROX1+ SC ECs which were also positive for Ki-67 (Control: 0.302 ± 0.022, Tek+/-: 0.214 ± 0.016), suggesting that the hypomorphic canal phenotype in these animals may be due to reduced proliferation during canal development (Figure 2B, quantified in C). Strikingly, normal proliferation of PROX1+ SC cells was observed in Tek+/-;PtprbNLS-LacZ/WT mice (Tek+/-;PtprbNLS-LacZ/WT: 0.345 ± 0.039), providing a potential mechanism for the observed effects on canal area in adulthood. A similar effect was observed on PROX1 expression, which was dramatically decreased in the SC of Tek+/- eyes at P5 and appeared normal in the eyes of Tek+/-;PtprbNLS-LacZ/WT littermates (Norm. AFU: Control: 1.0 ± 0.12, Tek+/-: 0.49 ± 0.07, Tek+/-;PtprbNLS-LacZ/WT: 0.82 ± 0.065, Figure 2D).
In addition to decreased area, Tek+/- SCs are characterized by focal thinning and convolutions which are not present in WT littermates (Souma et al., 2016) (Figure 2E). Interestingly, although Ptprb haploinsufficency resulted in increased proliferation and expanded canal area in Tek+/-;PtprbNLS-LacZ/WT animals, these focal defects were still observed—suggesting that directional signaling may play a role in proper canal formation which cannot be recapitulated by nonspecific TEK activation.
We next examined the effect of altered SC area in Tek+/- and Tek+/-;PtprbNLS-LacZ/WT mice on aqueous humor homeostasis. Tek heterozygous mice on an outbred background were found to have elevated IOP at 30 weeks of age (Figure 3A, Control: 13.7 ± 0.23, Tek+/- 18.15±0.33 mmHg). Consistent with observations of SC morphology, while PtprbNLS-LacZ/WT mice had normal IOP (13.81 ± 0.82 mmHg), incorporation of this allele into the Tek+/- model was beneficial and blunted the ocular hypertension associated with Tek haploinsufficiency, despite the presence of focal morphological defects (Tek+/-;PtprbNLS-LacZ/WT IOP: 14.92 ± 0.31 mmHg). To confirm the impact of the PtprbNLS-LacZ-induced IOP reduction on the retina, we counted BRN3B positive ganglion cells in an additional cohort of mice at 19 weeks of age (Figure 3B). While Tek heterozygous mice showed a marked reduction in RGCs, this loss did not occur in Tek+/;PtprbNLS-LacZ/WT mice.
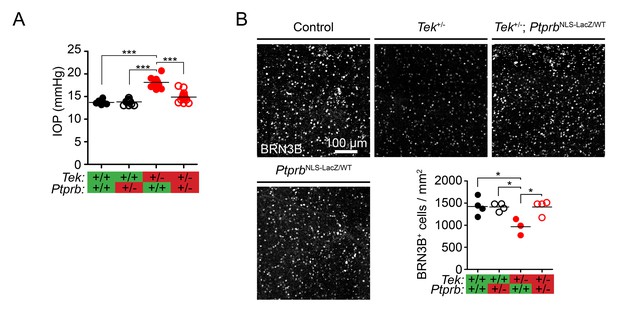
Ptprb heterozygosity prevents ocular hypertension and RGC loss in Tek haploinsufficient mice.
(A) Elevated intraocular pressure (IOP) was observed in Tek+/- haploinsufficient mice at 30 weeks of age when measured by rebound tonography. As in Figure 2, this phenotype was prevented in Tek+/-;PtprbNLS-LacZ/WT double heterozygous animals, confirming the importance of TEK activation in IOP homeostasis (n = 6 WT, 14 PtprbNLS-LacZ/WT, 12 Tek+/- and 14 Tek+/-;PtprbNLS-LacZ/WT mice). (B) BRN3B staining in retinal flat-mounts from a second group of mice revealed loss of retinal ganglion cells by 19 weeks in Tek+/- mice. Littermate Tek+/-;PtprbNLS-LacZ/WT animals were protected, correlating with the reduced IOP observed (n = 4 WT, 4 PtprbNLS-LacZ/WT, 3 Tek+/- and 4 Tek+/-;PtprbNLS-LacZ/WT mice). Horizontal lines indicate population means. *p<0.05, ***p<0.001 as determined by 1-way ANOVA followed by Bonferroni’s correction.
TEK signaling is critical for retinal angiogenesis, and to exclude the possibility that vascular defects and subsequent ischemia were responsible for RGC loss in Tek+/- mice, we examined vascular morphology in our animals. We first examined retinas collected at P5, when development of the superficial vasculature is ongoing. At this timepoint, CD31 staining revealed normal progress of the angiogenic sprouting front in Tek+/-, PtprbNLS-LacZ/WT, and Tek+/;PtprbNLS-LacZ/WT mice when compared to littermate controls (Figure 3—figure supplement 1A). At P20, when development of the mature retinal vasculature is complete, we observed normal patterning in all three retinal vascular layers in mice of all genotypes (Figure 3—figure supplement 1B). By this timepoint, hyaloid vessels were not observed in animals of any genotype. Taken together, these data suggest that blunted IOP elevation in Tek+/;PtprbNLS-LacZ/WT animals likely had a direct impact on glaucoma pathogenesis in our model and that retinal ischemia is unlikely to account for the RGC loss observed in Tek haploinsufficient mice.
Discussion
Ocular hypertension is the single most important risk factor for glaucoma in patients, and reducing IOP is the only therapeutic intervention proven to slow disease progression. Although aqueous humor flow through the conventional (trabecular) outflow pathway represents the majority of AHO, drugs targeting this outflow pathway were not available until the recent introduction of the Rho kinase inhibitors ripasudil and netarsudil. However, while these molecules provide exciting new treatment options, clinical trials have not shown them to be superior to current standard of care therapies for lowering IOP (Tanna and Johnson, 2018), underscoring the need for additional drug targets and treatment options.
Angpt-TEK signaling is essential for development (Thomson et al., 2014; Souma et al., 2016; Thomson et al., 2017) and maintenance (Kim et al., 2017) of SC, an essential component of the conventional AHO pathway. Dysregulation of Angpt-TEK signaling results in hypomorphic or failed SC formation, elevated IOP and glaucoma in mice and humans. Intriguingly, recent GWAS studies of subjects with ocular hypertension have identified common variants in members of the Angpt-TEK signaling pathway (Khawaja et al., 2018; Gao et al., 2018; MacGregor et al., 2018). These GWAS findings suggest that in addition to the complete loss-of-function variants previously described in PCG, less impactful variants may play a role in more common forms of adult-onset glaucoma. These reports, combined with the strong dose dependent response of SC development and function to TEK signaling observed in the mouse, suggest that the Angpt-TEK signaling axis is a sensitive and important regulator of SC and AHO and may provide a valuable IOP-lowering therapeutic target for treatment of glaucoma patients. Indeed, a recent study has demonstrated the effectiveness of targeting the Angpt-TEK signaling pathway in a mouse model of glaucoma using an antibody which increases the signaling ability of the context-dependent, weak TEK agonist ANGPT2 by clustering it into higher-order multimers (Kim et al., 2017; Park et al., 2016). ANGPT2-clustering antibody treatment was found to increase TEK phosphorylation in aged mice and lower IOP in an injury-induced model of ocular hypertension (Kim et al., 2017).
Here, we demonstrate an alternative approach targeting the endothelial phosphatase PTPRB, which acts on TEK (Souma et al., 2018; Winderlich et al., 2009; Bäumer et al., 2006) as well as VE-Cadherin, VEGFR2 and FGD5 (Nawroth et al., 2002; Broermann et al., 2011; Mellberg et al., 2009; Hayashi et al., 2013; Braun et al., 2019). Using a genetic approach to PTPRB ablation allowed us to achieve consistent reduction in protein levels, with deletion of a single Ptprb allele leading to approximately 50% reduction in protein expression. In Tek-WT lung tissue, this was sufficient to cause a marked elevation in TEK phosphorylation. PTPRB inhibition has been previously studied as a potential therapeutic intervention in vascular disease (Shen et al., 2014), and the PTPRB inhibitor AKB-9778 is currently undergoing clinical trials for treatment of diabetic macular edema, where it is well-tolerated by patients (Campochiaro et al., 2016). While mid-term results for the AKB-9778 TIME-2 trial failed to achieve the primary endpoint in diabetic macular edema, two important observations were reported from this clinical trial: the drug was well tolerated and safe in patients and IOP was lower in the treatment arm (Aerpio Pharmaceuticals Inc, 2019).
We have previously reported that defects in Angpt-TEK signaling cause a range of SC and IOP phenotypes corresponding to the degree of pathway dysfunction, ranging from complete absence of SC (Tek knockout or Angpt1;Angpt2 double knockout mice) to less severely hypomorphic canal morphology (Tek haploinsufficency) (Thomson et al., 2014; Kim et al., 2017; Souma et al., 2016; Thomson et al., 2017). Although the phenotype of Tek haploinsufficient mice is milder than that observed in PCG patients with heterozygous TEK loss-of-function mutations (Souma et al., 2016), we selected this model as Tek heterozygous mice are viable, fertile and exhibit a consistent SC phenotype without the need for Cre recombinase-mediated gene deletion. Furthermore, this mouse model has many features of glaucoma including ocular hypertension and loss of retinal ganglion cells which more faithfully recapitulate disease seen in patients with common forms of open angle glaucoma then the more severe model of total Tek deletion. Consistent with our previous findings, adult Tek+/- mice in this study displayed a hypomorphic SC phenotype due to decreased EC proliferation which was associated with elevated IOP and loss of BRN3B+ retinal ganglion cells. These defects were blunted by developmental Ptprb haploinsufficency in Tek+/-;PtprbNLS-LacZ/WT mice, likely due to increased basal TEK phosphorylation and resulting EC proliferation in PtprbNLS-LacZ/WT animals. In addition, expression of PROX1, a key marker of mature SC endothelial cells, was dramatically reduced in Tek+/- SCs at P5. Expression was normal in Tek+/-;PtprbNLS-LacZ/WT eyes, consistent with previous reports from our lab and elsewhere suggesting that Angpt1-TEK signaling may be critical for maintenance of this crucial transcription factor in the SC endothelium (Park et al., 2014; Kim et al., 2017; Thomson et al., 2017; Truong et al., 2014). However, our data do not indicate whether PROX1 expression is directly downstream of TEK activation or is otherwise dependent on a mature SC endothelial phenotype.
While these results highlight the potential of PTPRB as a therapeutic target for patients with TEK-associated PCG, the recent association of Angpt-TEK variants with ocular hypertension and the findings of Kim et al using an ANGPT2 clustering antibody suggest that this pathway may also provide a valuable therapeutic target for adult patients with primary open angle glaucoma, a significantly larger group then TEK-associated PCG. Future studies using targeted gene deletion or therapies that inhibit PTPRB such as siRNA, antibodies or small molecule inhibitors will provide additional insights and pave the way for translation of these findings into the clinic.
Materials and methods
Resource | Designation | Source or reference | Identifiers | Additional information |
---|---|---|---|---|
Genetic Reagent (M. musculus) | PtprbNLS-LacZ | Bäumer et al., 2006 | Maintained on a mixed background | |
Genetic Reagent (M. musculus) | Tek+/- | Thomson et al., 2017 | Tektm1.1Vlcg; MGI:5544795 | Maintained on a mixed background |
Antibody | anti-PTPRB (Rabbit polyclonal) | Nawroth et al., 2002 | Western blot: 1:2000 | |
Antibody | Anti-TEK (Rabbit polyclonal) | Santa Cruz Biotech | sc-324 | Western blot 1:2500 |
Antibody | anti-αTubulin (Mouse monoclonal) | Santa Cruz Biotech | sc-32293 | Western blot: 1:10,000 |
Antibody | 4G10 Platinum anti-phosphotyrosine (Mouse monoclonal) | Millipore | 05–1050 | Western blot: 1:2000 |
Antibody | anti-CD31 MEC13.3 (Rat monoclonal) | BD Biosciences | 55337 | IF: 1:100 |
Antibody | anti-PROX1 (Goat polyclonal) | R and D Systems | AF2727 | IF: 1:200 |
Antibody | anti-Ki-67 (Rabbit monoclonal) | ThermoFisher | MA5-14520 | IF: 1:200 |
Antibody | anti-BRN3b (Goat polyclonal) | Santa Cruz Biotech | sc-6026 | IF: 1:1000 |
Software, algorithm | ImageJ Fiji | Schindelin et al., 2012 | Version 1.52 p | Used for all image analysis |
Software, algorithm | Graphpad Prism | Graphpad.com | Version 5.0 | Used for statistical analysis and graph generation |
Software, algorithm | Adobe Indesign | Adobe.com | Version 14.01 × 64 | Used for figure creation |
Study approval
Request a detailed protocolThis study was performed in strict accordance with the recommendations in the Guide for the Care and Use of Laboratory Animals of the National Institutes of Health and the ARVO guidelines for care and use of vertebrate research subjects in eye research. All animal experiments were approved by the Animal Care Committee at the Center for Comparative Medicine of Northwestern University (Evanston, Illinois, USA).
Generation and breeding of Tek+/-;PtprbNLS-LacZ/WTmice
Request a detailed protocolPtprbNLS-LacZ/WT mice have been previously described (Winderlich et al., 2009; Bäumer et al., 2006) and were a generous gift of Dr. Dietmar Vestweber (Max Planck Institute, Münster, Germany). Tek+/- mice were generated by crossing TekCOIN (Thomson et al., 2014; Economides et al., 2013) mice with Rosa26rtTA;TetOnCre (Belteki et al., 2005) as previously described (Souma et al., 2016). After undergoing Cre-mediated gene deletion, animals were crossed with WT ICR mice to obtain Tek+/- animals which did not express the TetOnCre or Rosa26rtTA transgenes. Throughout the present study, animals were maintained on a mixed genetic background free of the retina degeneration mutations RD1 and RD8 and allowed unrestricted access to standard rodent chow (Harlan Teklad #7912; Envigo, Indianapolis IN) and water. As animals were maintained on a mixed background, littermate controls were used for all experiments and animals were included in the study on the basis of full litters (i.e. a control from one litter would not be included without their matching mutant littermates). To determine experimental group sizes, data from our previous studies of SC morphology (Souma et al., 2016; Thomson et al., 2017) were used to estimate required numbers. Breeding cages were then set up based on these estimates, and all resulting animals were included in the described studies.
Western blot
Request a detailed protocolLung samples from Tek+/-, PtprbNLS-LacZ/WT and Tek+/-, PtprbNLS-LacZ/WT mice with control littermates were homogenized in RIPA buffer (50 mM TRIS, 150 mM NaCl, 1% IGEPAL CA-630, 0.5% Na Deoxycholate, 0.1% SDS, pH 7.5) containing protease and phosphatase inhibitor cocktails (Sigma). Samples were lysed (30 min at 4°C) and centrifuged (10 min at 14,000*g, 4°C) before the supernatant was used for western blot and immunoprecipitation. For western blot, 100 μg lysate was separated on a 4–15% Tris-glycine gel (Bio-Rad) and transferred to PVDF membranes using standard methods. Membranes were then cut horizontally at 75 kDa and blocked in (5% BSA in Tris buffered saline containing 0.05% Tween-20, pH 7.5) before incubating with appropriate primary and HRP-conjugated secondary antibodies (Jackson Immunoresearch). After washing, membranes were incubated in ECL substrate (SuperSignal West Pico PLUS, Thermo Fisher) and imaged on an iBright 1500 digital camera system (Thermo Fisher-Life Technologies). Quantification was performed using ImageJ Fiji software (Schindelin et al., 2012). Values obtained from quantifying total TEK or PTPRB were normalized to matching alpha-tubulin bands obtained from the lower (<75 kDA) region of the same membrane. Primary antibodies used: Rabbit anti-TEK (Santa Cruz Biotech #sc-324, 1:2500), mouse anti-alpha tubulin (Santa Cruz Biotech #sc-32293, 1:10,000), rabbit anti-PTPRB (Nawroth et al., 2002).
For Immunoprecipitation assays of TEK phosphorylation, 1 mg of protein lysate was incubated with 1 μg rabbit anti-TEK antibody (C-20, Santa Cruz) before antibody-protein complexes were captured using Protein-A conjugated Dynabeads (Invitrogen). Proteins were then eluted by boiling in 2x Laemmli sample buffer containing 100 mM DTT, loaded on a 4–15% Tris-glycine gel and separated by SDS-PAGE as described above. Phosphorylated tyrosine was detected using mouse anti-phospho-tyrosine antibody (4G10 Platinum, Millipore #05–1050, 1:2000) before membranes were stripped using a commercial stripping solution (Restore, Thermo Fisher # 21059) and re-probed using anti-TEK antibody as described above. Bands were imaged using a ChemiDock imaging system (Bio-Rad) and quantified as above. Relative TEK phosphorylation is reported in the manuscript as a normalized ratio of pTyr:Total TEK signals obtained from the same membrane. All western blot and immunoprecipitation experiments were performed at least twice. Values and statistics reported are derived from the data shown in the manuscript.
Schlemm’s canal immunostaining and imaging
Request a detailed protocolWhole-mount imaging of SC was performed as described previously (Thomson et al., 2017; Thomson and Quaggin, 2018). Briefly, enucleated eye globes were immersion fixed (2% formaldehyde in 0.1M phosphate buffer pH 7.5, 12 hr at 4°C) before the lens and retina were removed and limbal flat mounts were prepared. Tissues were blocked (5% donkey serum, 2.5% bovine serum albumin in Tris buffered saline pH 7.5 containing 0.5% Triton X-100, overnight at 4°C) before incubating in appropriate primary and alexafluor-labled secondary antibodies (Thermo Fisher Scientific, Waltham, MA) diluted in additional blocking buffer. Antibodies used: Rat anti-mouse CD31 (Dilution 1:100. #55337, BD Biosciences, Franklin Lakes New Jersey), Rabbit anti-human Ki-67 (Dilution 1:200. #MA5-14520, ThermoFisher Scientific, Waltham, MA), goat anti-human PROX1 (Dilution 1:200. #AF2727, R and D Systems, Minneapolis, MN). After staining, tissues were washed (Tris buffered saline pH 7.5 containing 0.05% Tween-20) and mounted on microscope slides. Images were captured using a Nikon A1R confocal microscope at the Center for Advanced Microscopy at Northwestern University equipped with a 20x objective with a numerical aperture of 0.75. To measure SC area, 3 10-image Z stacks were captured using a step size of 1.67 μm and a pinhole of 1.2 Airy units at intervals around the circumference of SC. To prevent bias in image location selection, the episclaral vein was used as a landmark for the initial field and subsequent images were taken at 120° intervals. In the manuscript, images of SC are shown as maximum intensity projections of these 10-image Z stacks. Canal area was measured in each 65,536 μm2 field using Fiji software (Schindelin et al., 2012) and an average value was obtained for each eye. Likewise, quantification of Ki-67 and PROX1 expression was performed in three imaging zones from each eye and the results were averaged to obtain the reported value for the animal. eight image confocal Z stacks were obtained with a step size of 1 μm and pinhole of 1.2. Ki-67 positive nuclei were counted manually using ImageJ Fiji software. PROX1 expression was reported as mean background-subtracted fluorescence per μm2 CD31+ SC area as measured using ImageJ Fiji software. Quantification of convolutions and focal defects was performed from stitched images of the full SC circumference obtained using the objective described above with the pinhole set to 150 μm.
Intraocular pressure measurement
Request a detailed protocolIOP measurements were obtained from awake mice using a Tonolab rebound tonometer (iCare) as previously described (Thomson et al., 2014; John et al., 1997). Animals were restrained in a soft plastic cone, and average IOPs were recorded from 3 sets of 6 recordings performed by a blinded technician. Finding no difference between left and right eyes, we have reported all IOP measurements as single averaged values for each animal.
Retinal ganglion cell quantification
Request a detailed protocolEnucleated eyes from Tek+/-;PtprbNLS-LacZ/WT mice with littermate Tek+/-, PtprbNLS-LacZ/WT, and WT controls were collected at 19 weeks and fixed as above. Retinas were collected before blocking and staining as above. Antibodies used: Goat anti BRN3 (Dilution: 1:1000. Santa Cruz Biotechnology, sc-6026). Retinas were imaged in a standardized pattern as flat mounts using a Nikon A1R microscope as described above and BRN3+ ganglion cells were quantified by a blinded student.
Statistical analysis
Request a detailed protocolStatistical analysis was performed using Prism 5.0 software (Graphpad, La Jolla CA USA). Two-tailed Student’s t-test or ANOVA followed by use of Bonferroni’s method for multiple comparisons were used for statistical significance testing as appropriate. Throughout the text, values are reported as means ± standard error (SEM). In figures, plotted data points represent average values from individual animals with horizontal lines indicating the group mean. p-values<0.05 were considered significant and are indicated in figures using the following notation: *p<0.05, **p<0.01 and ***p<0.001.
Data availability
All data described have been included in the manuscript. No data sets were generated during the course of this study.
References
-
The schlemm's canal is a VEGF-C/VEGFR-3-responsive lymphatic-like vesselJournal of Clinical Investigation 124:3975–3986.https://doi.org/10.1172/JCI75395
-
Tonography in the diagnosis of simple (open angle) glaucomaTransactions - American Academy of Ophthalmology and Otolaryngology 65:156–162.
-
Dissociation of VE-PTP from VE-cadherin is required for leukocyte extravasation and for VEGF-induced vascular permeability in vivoThe Journal of Experimental Medicine 208:2393–2401.https://doi.org/10.1084/jem.20110525
-
Targeting VE-PTP phosphatase protects the kidney from diabetic injuryThe Journal of Experimental Medicine 216:936–949.https://doi.org/10.1084/jem.20180009
-
Risk factors for Glaucoma onset and progressionSurvey of Ophthalmology 53 Suppl1:S3–S10.https://doi.org/10.1016/j.survophthal.2008.08.006
-
Genome-wide association analyses identify new loci influencing intraocular pressureHuman Molecular Genetics 27:2205–2213.https://doi.org/10.1093/hmg/ddy111
-
Intraocular pressure in inbred mouse strainsInvestigative Ophthalmology & Visual Science 38:249–253.
-
Unconventional aqueous humor outflow: a reviewExperimental Eye Research 158:94–111.https://doi.org/10.1016/j.exer.2016.01.017
-
Oligomerization and multimerization are critical for angiopoietin-1 to bind and phosphorylate Tie2Journal of Biological Chemistry 280:20126–20131.https://doi.org/10.1074/jbc.M500292200
-
Impaired angiopoietin/Tie2 signaling compromises schlemm's canal integrity and induces glaucomaJournal of Clinical Investigation 127:3877–3896.https://doi.org/10.1172/JCI94668
-
Assessment of aqueous humor dynamics in the mouse by a novel method of Constant-Flow infusionInvestigative Opthalmology & Visual Science 52:685–694.https://doi.org/10.1167/iovs.10-6069
-
Strain and age effects on aqueous humor dynamics in the mouseInvestigative Opthalmology & Visual Science 56:5764–5776.https://doi.org/10.1167/iovs.15-16720
-
Lymphatic regulator PROX1 determines schlemm's canal integrity and identityJournal of Clinical Investigation 124:3960–3974.https://doi.org/10.1172/JCI75392
-
Validating therapeutic targets through human geneticsNature Reviews Drug Discovery 12:581–594.https://doi.org/10.1038/nrd4051
-
Therapeutic targeting of the angiopoietin-TIE pathwayNature Reviews Drug Discovery 16:635–661.https://doi.org/10.1038/nrd.2016.278
-
Fiji: an open-source platform for biological-image analysisNature Methods 9:676–682.https://doi.org/10.1038/nmeth.2019
-
Targeting VE-PTP activates TIE2 and stabilizes the ocular vasculatureJournal of Clinical Investigation 124:4564–4576.https://doi.org/10.1172/JCI74527
-
Angiopoietin receptor TEK mutations underlie primary congenital Glaucoma with variable expressivityJournal of Clinical Investigation 126:2575–2587.https://doi.org/10.1172/JCI85830
-
A lymphatic defect causes ocular hypertension and Glaucoma in miceJournal of Clinical Investigation 124:4320–4324.https://doi.org/10.1172/JCI77162
-
Angiopoietin-1 is required for Schlemm's canal development in mice and humansJournal of Clinical Investigation 127:4421–4436.https://doi.org/10.1172/JCI95545
-
BookMorphological Analysis of Schlemm’s Canal in MiceIn: Oliver G, Kahn M. L, editors. Lymphangiogenesis: Methods and Protocols. New York: Springer. pp. 153–160.https://doi.org/10.1007/978-1-4939-8712-2_10
-
Aqueous humor dynamics in the aging human eyeAmerican Journal of Ophthalmology 127:407–412.https://doi.org/10.1016/S0002-9394(98)00436-X
-
Aqueous humor dynamics in monkeys with laser-induced GlaucomaJournal of Ocular Pharmacology and Therapeutics 16:19–27.https://doi.org/10.1089/jop.2000.16.19
-
VE-PTP controls blood vessel development by balancing Tie-2 activityThe Journal of Cell Biology 185:657–671.https://doi.org/10.1083/jcb.200811159
Article and author information
Author details
Funding
National Institutes of Health (R01 HL124120)
- Susan E Quaggin
National Institutes of Health (R01 EY025799)
- Susan E Quaggin
National Institutes of Health (P30 DK114857)
- Susan E Quaggin
The funders had no role in study design, data collection and interpretation, or the decision to submit the work for publication.
Acknowledgements
We are grateful to Megan Kelly and Veronica Ramirez for technical assistance. This study was funded by NIH R01 HL124120, R01 EY025799 (to SEQ). Imaging was performed at the Northwestern University Center for Advanced Microscopy supported by NCI CCSG P30 CA060553 awarded to the Robert H Lurie Comprehensive Cancer Center. We also acknowledge support from the George M O’Brien Kidney Core Grant P30 DK114857.
Ethics
Animal experimentation: This study was performed in strict accordance with the recommendations in the Guide for the Care and Use of Laboratory Animals of the National Institutes of Health and the ARVO guidelines for care and use of vertebrate research subjects in eye research. All animal experiments were approved by the Animal Care Committee at the Center for Comparative Medicine of Northwestern University (Evanston, Illinois, USA) under animal protocols IS00002777, IS00006571 and IS00003091.
Copyright
© 2019, Thomson et al.
This article is distributed under the terms of the Creative Commons Attribution License, which permits unrestricted use and redistribution provided that the original author and source are credited.
Metrics
-
- 1,823
- views
-
- 290
- downloads
-
- 30
- citations
Views, downloads and citations are aggregated across all versions of this paper published by eLife.
Download links
Downloads (link to download the article as PDF)
Open citations (links to open the citations from this article in various online reference manager services)
Cite this article (links to download the citations from this article in formats compatible with various reference manager tools)
Further reading
-
- Cancer Biology
- Developmental Biology
Missense ‘hotspot’ mutations localized in six p53 codons account for 20% of TP53 mutations in human cancers. Hotspot p53 mutants have lost the tumor suppressive functions of the wildtype protein, but whether and how they may gain additional functions promoting tumorigenesis remain controversial. Here, we generated Trp53Y217C, a mouse model of the human hotspot mutant TP53Y220C. DNA damage responses were lost in Trp53Y217C/Y217C (Trp53YC/YC) cells, and Trp53YC/YC fibroblasts exhibited increased chromosome instability compared to Trp53-/- cells. Furthermore, Trp53YC/YC male mice died earlier than Trp53-/- males, with more aggressive thymic lymphomas. This correlated with an increased expression of inflammation-related genes in Trp53YC/YC thymic cells compared to Trp53-/- cells. Surprisingly, we recovered only one Trp53YC/YC female for 22 Trp53YC/YC males at weaning, a skewed distribution explained by a high frequency of Trp53YC/YC female embryos with exencephaly and the death of most Trp53YC/YC female neonates. Strikingly, however, when we treated pregnant females with the anti-inflammatory drug supformin (LCC-12), we observed a fivefold increase in the proportion of viable Trp53YC/YC weaned females in their progeny. Together, these data suggest that the p53Y217C mutation not only abrogates wildtype p53 functions but also promotes inflammation, with oncogenic effects in males and teratogenic effects in females.
-
- Developmental Biology
Stem cell self-renewal often relies on asymmetric fate determination governed by niche signals and/or cell-intrinsic factors but how these regulatory mechanisms cooperate to promote asymmetric fate decision remains poorly understood. In adult Drosophila midgut, asymmetric Notch (N) signaling inhibits intestinal stem cell (ISC) self-renewal by promoting ISC differentiation into enteroblast (EB). We have previously shown that epithelium-derived Bone Morphogenetic Protein (BMP) promotes ISC self-renewal by antagonizing N pathway activity (Tian and Jiang, 2014). Here, we show that loss of BMP signaling results in ectopic N pathway activity even when the N ligand Delta (Dl) is depleted, and that the N inhibitor Numb acts in parallel with BMP signaling to ensure a robust ISC self-renewal program. Although Numb is asymmetrically segregated in about 80% of dividing ISCs, its activity is largely dispensable for ISC fate determination under normal homeostasis. However, Numb becomes crucial for ISC self-renewal when BMP signaling is compromised. Whereas neither Mad RNA interference nor its hypomorphic mutation led to ISC loss, inactivation of Numb in these backgrounds resulted in stem cell loss due to precocious ISC-to-EB differentiation. Furthermore, we find that numb mutations resulted in stem cell loss during midgut regeneration in response to epithelial damage that causes fluctuation in BMP pathway activity, suggesting that the asymmetrical segregation of Numb into the future ISC may provide a fail-save mechanism for ISC self-renewal by offsetting BMP pathway fluctuation, which is important for ISC maintenance in regenerative guts.