Clustered gamma-protocadherins regulate cortical interneuron programmed cell death
Abstract
Cortical function critically depends on inhibitory/excitatory balance. Cortical inhibitory interneurons (cINs) are born in the ventral forebrain and migrate into cortex, where their numbers are adjusted by programmed cell death. Here, we show that loss of clustered gamma protocadherins (Pcdhg), but not of genes in the alpha or beta clusters, increased dramatically cIN BAX-dependent cell death in mice. Surprisingly, electrophysiological and morphological properties of Pcdhg-deficient and wild-type cINs during the period of cIN cell death were indistinguishable. Co-transplantation of wild-type with Pcdhg-deficient interneuron precursors further reduced mutant cIN survival, but the proportion of mutant and wild-type cells undergoing cell death was not affected by their density. Transplantation also allowed us to test for the contribution of Pcdhg isoforms to the regulation of cIN cell death. We conclude that Pcdhg, specifically Pcdhgc3, Pcdhgc4, and Pcdhgc5, play a critical role in regulating cIN survival during the endogenous period of programmed cIN death.
Introduction
GABAergic cortical inhibitory interneurons (cINs) regulate neuronal circuits in the neocortex. The ratio of inhibitory interneurons to excitatory neurons is crucial for establishing and maintaining proper brain function (Rossignol, 2011; Chao et al., 2010; Marín, 2012; Hattori et al., 2017; Huang et al., 2007; Rubenstein and Merzenich, 2003). Alterations in the number of cINs have been linked to epilepsy (Dudek and Shao, 2003), schizophrenia (Beasley and Reynolds, 1997; Hashimoto et al., 2003; Enwright et al., 2016) and autism (Gao and Penzes, 2015; Cellot and Cherubini, 2014; Fatemi et al., 2009). During mouse embryonic development, the brain produces an excess number of cINs and ~40% of those are subsequently eliminated by apoptosis during early postnatal life, between postnatal day (P)1 and 15 (Southwell et al., 2012; Denaxa et al., 2018; Wong et al., 2018). What makes the death of these cells intriguing is its timing and location. In normal development, cINs are generated in the medial and caudal ganglionic eminences (MGE; CGE) of the ventral forebrain, far from their final target destination in the cortex. cINs migrate tangentially from their site of origin to reach the neocortex, where they become synaptically integrated and complete their maturation (Anderson et al., 1997; Wichterle et al., 2001; Butt et al., 2005; Nery et al., 2003). The ganglionic eminences are also an important source of interneurons in the developing human brain, where migration and differentiation extend into postnatal life (Hansen et al., 2013; Paredes et al., 2016; Ma et al., 2013). How is the final number of cINs regulated once these cells arrive in the cortex?
Since cINs play a pivotal role in regulating the level of cortical inhibition, the adjustment of their number by programmed cell death is a key feature of their development and essential for proper brain physiology. While recent work suggests that activity-dependent mechanisms regulate cIN survival through their connectivity to excitatory neurons (Wong et al., 2018; Denaxa et al., 2018; Duan et al., 2020; Priya et al., 2018) studies indicate that cIN survival is mediated by a population-autonomous (or cell-autonomous) mechanism (Southwell et al., 2012). Heterochronically transplanted MGE cIN precursors undergo a wave of apoptosis coinciding with their age, which is asynchronous from endogenous cINs. Whereas it is well established that neuronal survival in the peripheral nervous system (PNS) is regulated through limited access to neurotrophic factors secreted by target cells (Huang and Reichardt, 2001; Aloe and Chaldakov, 2013; Oppenheim and Carolanne, 2013), cIN survival is independent of TrkB, the main neurotrophin receptor expressed by neurons of the CNS (Southwell et al., 2012; Rauskolb et al., 2010). Moreover, the proportion of cINs undergoing apoptosis remains constant across graft sizes that vary 200-fold (Southwell et al., 2012). Taken together, this work suggests that cIN developmental death is intrinsically determined and that cell-autonomous mechanisms within the maturing cIN population contribute to the regulation of their survival.
The clustered protocadherins (Pcdh) (Wu and Maniatis, 1999) are a set of cell surface homophilic-binding proteins implicated in neuronal survival and self-avoidance in the spinal cord, retina, cerebellum, hippocampus, and olfactory bulb glomeruli (Ing-Esteves et al., 2018; Wang et al., 2002b; Lefebvre et al., 2012, Lefebvre et al., 2008; Katori et al., 2017; Mountoufaris et al., 2017; Chen et al., 2017). In the mouse, the Pcdh locus encodes a total of 58 isoforms that are arranged in three gene clusters, alpha, beta and gamma: Pcdha, Pcdhb, and Pcdhg (Wu et al., 2001). The Pcdha and Pcdhg isoforms are each composed of a set of variable exons, which are spliced to three common constant cluster-specific exons (Tasic et al., 2002; Wang et al., 2002a). Each variable exon codes for the extracellular, transmembrane and most-proximal intracellular domain of a protocadherin protein. The Pcdhb isoforms are encoded by single exon genes encoding both extracellular, transmembrane and cytoplasmic domains (Wu and Maniatis, 1999). Of the 58 Pcdh genes, it has been suggested that a combinatorial, yet stochastic, set of isoforms is expressed in each neuron (Esumi et al., 2005; Kaneko et al., 2006; Mountoufaris et al., 2017), suggesting a source for neuronal diversity in the CNS (Canzio et al., 2019). Interestingly, Pcdhg genes, and specifically isoforms Pcdhgc3, Pcdhgc4, and Pcdhgc5, are required for the postnatal survival of mice (Wang et al., 2002b; Hasegawa et al., 2016; Chen et al., 2012). Whether Pcdh genes are required for the regulation of cIN elimination remains unknown.
In the present study, we used a series of genetic deletions of the Pcdh gene locus to probe the role of clustered Pcdhs in the regulation of cIN cell death in mice. We show that Pcdhg, but not Pcdha or Pcdhb, are required for the survival of approximately 50% of cINs through a BAX-dependent mechanism. Using co-transplantation of Pcdhg-deficient and wild-type (WT) cINs of the same age, we show that they compete for survival in a mechanism that involves Pcdhg. Taking advantage of the transplantation assay, we show that removal of the three Pcdhg isoforms, Pcdhgc3, Pcdhgc4, and Pcdhgc5, is sufficient to increase cell death of MGE-derived cINs. Three-dimensional reconstructions and patch-clamp recordings indicate that the Pcdhg mutant cells have similar morphology, excitability and receive similar numbers of inhibitory and excitatory synaptic inputs compared to wild type cINs. We conclude that cIN cell death is regulated by all or some of the C-isoforms in the Pcdhg cluster and that this process is independent of the structural complexity or intrinsic physiological properties of the cell or the strength of its excitatory and inhibitory synaptic inputs.
Results
Pcdhg expression in developing cINs
Expression of clustered protocadherins (Pcdh) in the brain starts in the embryo and continues postnatally (Hirano et al., 2012; Frank et al., 2005; Wang et al., 2002b; Kohmura et al., 1998). RT-PCR analysis revealed the expression of each of the 58 isoforms in the Pcdh gene locus in the adult cortex (P30) (Figure 1A). Of the 58 Pcdh genes, those in the Pcdhg cluster are essential for postnatal survival (Hasegawa et al., 2016; Chen et al., 2012), and are implicated in cell death in the retina and spinal cord (Lefebvre et al., 2008; Prasad et al., 2008). We, therefore, determined whether Pcdhg genes are expressed in cINs during the period of cIN cell death. Using Gad1-GFP mice to label GABAergic cINs (Tamamaki et al., 2003), we FACS-sorted GFP-positive (GFP+) and GFP-negative (GFP-) cells from P7 mice at the peak of cIN cell death (Figure 1—figure supplement 1A). We confirmed that GABAergic cell markers (Gad1, Gad2) were enriched in the GFP+ population, while markers of excitatory neurons (Tbr1, Satb2, Otx1), astrocytes (Gfap, Aldh1l1), and oligodendrocytes (Olig2, Mbp) were enriched in the GFP- population (Figure 1—figure supplement 1B). With the exception of Pcdhga9 isoform, we detected the expression of all other 21 Pcdhg in cINs (Figure 1B). To determine the expression pattern of Pcdhg at different stages during the period of cell death, we measured the expression level of 8 Pcdhg mRNAs (Pcdhgc3, Pcdhgc4, Pcdhgc5, Pcdhga1, Pcdhga2, Pcdhga3, Pcdhgb6, and Pcdhgb7) at P2, P5, P8, P12 and P15 using qPCR (Figure 1C). All eight isoforms were expressed in cINs at each of the five ages studied. Interestingly, the expression of Pcdhgc5 increased dramatically between P8 and P15. An increase in expression of Pcdhg isoforms Pcdhga1, Pcdhga2 and PcdhgC4 was also observed at P12, compared to other ages, but this increase was less pronounced than that observed for Pcdhgc5. The above results show that all Pcdh isoforms are expressed in cINs and that the expression of Pcdhg isoforms Pcdhga1, Pcdhga2 and PcdhgC4 and Pcdhgc5 increases during the period of postnatal cell death.
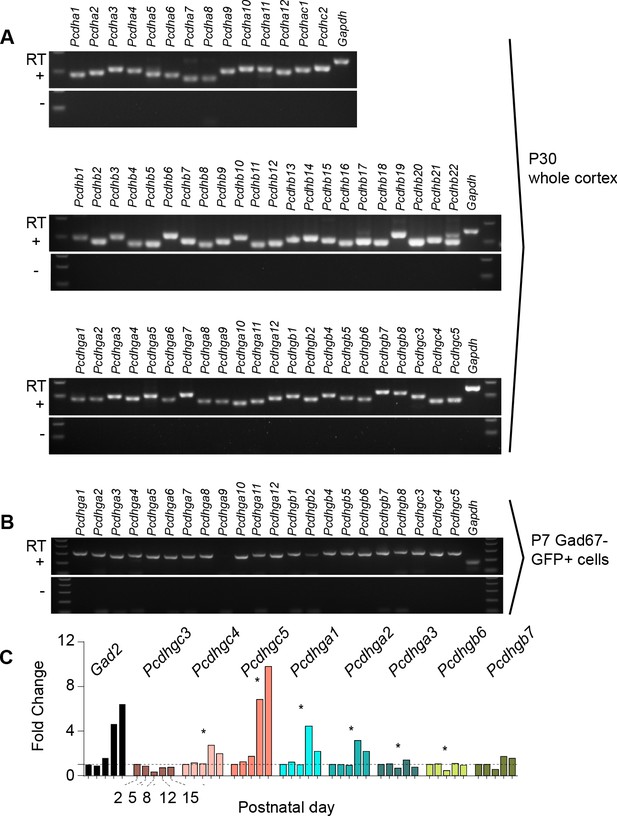
Expression of clustered Pcdhs in the mouse cortex and purified cortical GABAergic cells.
( A) PCR analysis of clustered Pcdh and Gapdh gene expression in P30 whole cortex extracts. (B) PCR analysis of Pcdhg and Gapdh gene expression in purified P7 cortical GABAergic cells. (C) Quantification of target gene mRNA levels at various postnatal stages (P2, P5, P8, P12, P15) in purified cortical GABAergic cells. P2 mRNA levels used as a reference for each gene (Kruskal-Wallis test, P value = 0.0007 [ Pcdhgc4], P value < 0.0001 [Pcdhgc5], P value = 0.015 [Pcdhga1], P value = 0.024[Pcdhga2], P value = 0.003[Pcdhga3], P value = 0.038[Pcdhgb6]; n = 3 technical replicas]. Significant p values are marked with*. See Figure 1—source data 1 for followup of comparisons.
-
Figure 1—source data 1
Pcdhg expression in cortex.
- https://cdn.elifesciences.org/articles/55374/elife-55374-fig1-data1-v2.xlsx
Reduced number of cINs in the cortex of Pcdhg mutants
Most cINs are produced between embryonic days (E) 10.5 and 16.5 by progenitors located in the medial and caudal ganglionic eminences (MGE and CGE) (Anderson et al., 1997; Wichterle et al., 2001; Nery et al., 2002; Miyoshi et al., 2010). To address the potential role of Pcdhg in cIN development, we used the Pcdhg conditional allele (Pcdhgfcon3) to block production of all 22 Pcdhg isoforms (Lefebvre et al., 2008). In the Pcdhgfcon3 allele, the third common exon shared by all Pcdhg isoforms contains the sequence coding for GFP and is flanked by loxP sites (Lefebvre et al., 2008; Figure 2A). In unrecombined Pcdhgfcon3 mice, all Pcdhg isoforms are thus fused to GFP. However, when these animals are crossed to a Cre driver line, expression of the entire Pcdhg cluster is abolished in Cre-expressing cells (Prasad et al., 2008). Robust GFP expression was detected throughout the brain in E13.5 embryos, including cells in the MGE and CGE (Figure 3B), indicating expression of Pcdhg isoforms in cIN progenitors. We crossed Pcdhgfcon3 mice to Gad2Cre mice (Taniguchi et al., 2011) to conditionally ablate all Pcdhg in GABAergic cells throughout the CNS at an early embryonic stage (E10.5) (Katarova et al., 2000). Recombined cells were visualized thanks to the conditional Ai14 (tdTomato) reporter expression (Figure 2A). Heterozygous Gad2Cre;Ai14;Pcdhgfcon3/+ mice were viable and fertile. However, homozygous Gad2Cre;Ai14 ;Pcdhgfcon3/fcon3 mice displayed growth retardation after birth, a hind limb paw-clasping phenotype when held by the tail and were infertile (Figure 2B). Brain size as well as cerebral cortex thickness of homozygous Gad2Cre;Ai14;Pcdhgfcon3/fcon3 was similar to those of control mice (Figure 2B’). However, the density of tdTomato positive cells in somatosensory and visual cortex was roughly halved in homozygous Gad2Cre;Ai14;Pcdhgfcon3/fcon3 animals, compared to wild type and heterozygous littermates (Figure 2C and C’). The density of cINs stained positive for parvalbumin (PV) and somatostatin (SST) (MGE-derived), vasoactive intestinal peptide (VIP )(CGE-derived) or reelin (RLN) (derived from both the MGE and CGE) was significantly reduced in the visual cortex of homozygous Gad2Cre;Ai14;Pcdhgfcon3/fcon3 mice (Figure 2D and Figure 2—figure supplement 1). Taken together, these experiments indicate that the embryonic loss of Pcdhg function in GABAergic progenitor cells leads to a drastically reduced number of cINs in the neocortex, affecting all cIN subtypes similarly.
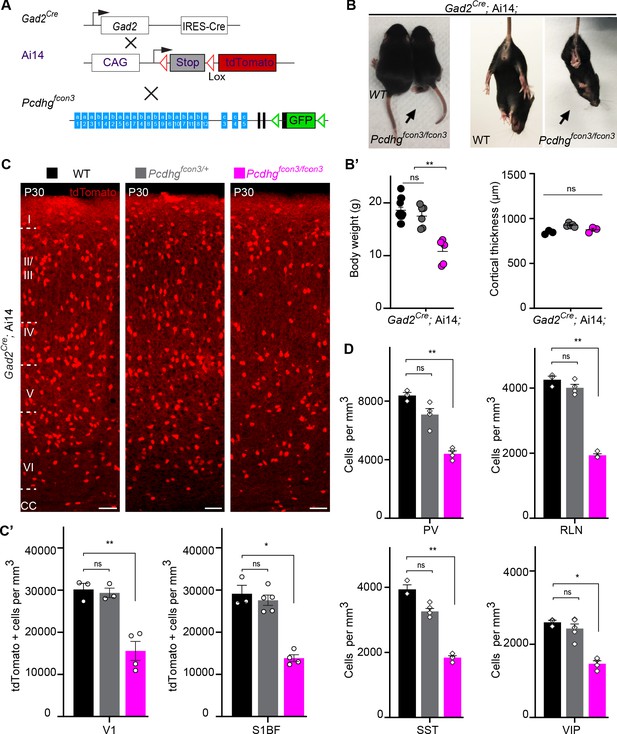
Reduced number of GABAergic cINs in Pcdhg-deficient mice.
(A) Mutant mice with loss of Pcdhg in GABAergic neurons were generated by crossing conditional Pcdhgfcon3 mice to Pan-GABAergic Cre driver (Gad2) mice. The conditional Ai14 reporter was used to fluorescently label Gad2-expressing cells. (B) Photographs of P21 Gad2Cre;Ai14 mice that are wild type (WT) or mutant (Pcdhgfcon3/fcon3) for Pcdhg. (B’) Body weight and cortical thickness measurements in P30 Gad2Cre;Ai14;Pcdhg+/+ (Pcdhg WT), Gad2Cre;Ai14;Pcdhgfcon3/+ (Pcdhg HET), and Gad2Cre;Ai14;Pcdhgfcon3/fcon3 (Pcdhg mutant) mice(Kruskal-Wallis test, P value=0.0027, adjusted p values **p=0.0017, n = 12 mice [Pcdhg WT], n = 7 mice [Pcdhg HET] and n = 5 mice [Pcdhg mutant]). (C) Photographs of coronal sections in primary visual cortex (V1) of P30 Gad2Cre;Ai14; Pcdhg WT (left), Pcdhg HET (middle) and Pcdhg mutant (right) mice. All cortical layers are similarly affected (Figure 2—figure supplement 2). Scale bar, 100 μm. (C’) Quantifications of tdTomato+ cell density in V1 and somatosensory (S1BF) cortex of P30 Gad2Cre;Ai14 Pcdhg WT(black), Pcdhg HET (grey), and Pcdhg mutant (magenta) mice (Kruskal-Wallis test; for V1 (P value = 0.006), for S1BF (P value = 0.009); adjusted p values **p=0.0180, *p=0.036, n = 3–5 mice of each genotype). (D) Quantifications of cIN subtype density in V1 cortex at P30. All four non-overlapping cIN subtypes (PV, SST, RLN, and VIP) were similarly reduced in numbers in Gad2Cre;Ai14;Pcdhgfcon3/fcon3 mice (Pcdhg mutant, magenta) compared to WT controls (Kruskal-Wallis test; for PV (P value = 0.0002), for SST (P value=0.0021), for RLN (P value = 0.0012), and for VIP (P value=0.0093); adjusted p values **p=0.004 (PV), **p=0.0073(SST), **p=0.0093(RLN), *p=0.0365 (VIP), n = 3–5 mice of each genotype).
-
Figure 2—source data 1
Quantification of GABAergic cINs, body weight and cortical thickness measurements in controls and Pcdhg deficient mice.
- https://cdn.elifesciences.org/articles/55374/elife-55374-fig2-data1-v2.xlsx
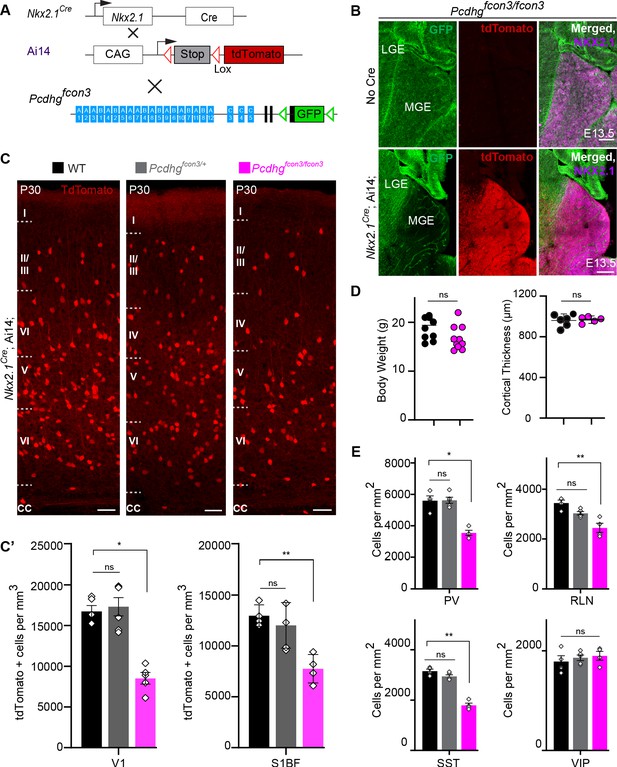
Loss of Pcdhg genes targeted to Nkx2.1 expressing cells results in selective loss of cIN derived from the MGE.
( A) Mutant mice with loss of Pcdhg in MGE-derived cIN were generated by crossing Pcdhgfcon3 mice to Nkx2.1Cre mice. The conditional Ai14 line was used to fluorescently label MGE-derived cells. (B) Pcdhgfcon3/fcon3 mice (at E13.5; top panels) carrying the Pcdhg mutant allele, but not Cre, show robust expression of GFP in the MGE. In contrast, in Nkx2.1Cre;Ai14;Pcdhgfcon3/fcon3 mice (at E13.5; bottom panels), carrying the Pcdhg mutant allele and expressing Cre, GFP expression was eliminated from the MGE. Note NKX2.1 staining (magenta) in the panels on the right delineates MGE/POA (preoptic area). The few cells left expressing GFP in the MGE are blood vessels and are tdTomato negative. (C) Photographs of coronal sections of the primary visual cortex (V1) in Nkx2.1Cre;Ai14;Pcdhg+/+ (Pcdhg WT), Nkx2.1Cre;Ai14;Pcdhgfcon3/+ (Pcdhg HET) and Nkx2.1Cre;Ai14;Pcdhgfcon3/fcon3 (Pcdhg mutant). Scale bar, 100 μm. (C’) Quantification of the density of tdTomato+ cells in V1 and S1BF cortex of P30 Nkx2.1Cre;Ai14;Pcdhg WT (black), Pcdhg HET (grey) and Pcdhg mutant (magenta) mice. The number of Nkx2.1-derived cells was significantly reduced in Nkx2.1Cre;Ai14;Pcdhg mutant mice compared to WT controls(Kruskal-Wallis test; for V1(P value=0.002), for S1BF (P value=0.0065), adjusted p values *p=0.0232, **p=0.0168 (S1), n = 4–6 mice of each genotype). (D) Body weight and cortex thickness measurements in Nkx2.1Cre;Ai14;Pcdhg WT (black) and Pcdhg mutant (magenta) mice at P30. Body weight and cortical thickness were not significantly affected by loss of Pcdhg (Mann-Whitney test, body weight (p=0.0547, n = 10 mice of each genotype), cortical thickness (p=0.2857, n = 4–5 mice of each genotype). (E) Quantification of tdTomato+ cIN subtypes in V1 mouse cortex at P30. Nkx2.1Cre;Ai14;Pcdhg mutant mice (magenta) had significantly reduced numbers of MGE-derived parvalbumin (PV)+, somatostatin (SST)+, and Reelin (RLN)+ cells compared to WT controls. In contrast VIP+ cells, which are derived from the CGE, were not significantly affected (Kruskal-Wallis test; for PV (P value = 0.0113), for SST (P value=0.0009), for RLN (P value = 0.0014), and for VIP (P value=0.636); adjusted p values *p=0.0113 (PV), **p=0.0055 (SST), **p=0.0055 (RLN), n = 4–5 mice of each genotype).
-
Figure 3—source data 1
Quantification of Nkx2.1-derived cINs, body weight and cortical thickness measurements in controls and Pcdhg-deficient mice.
- https://cdn.elifesciences.org/articles/55374/elife-55374-fig3-data1-v2.xlsx
The developmental defects observed in Gad2Cre;Ai14;Pcdhgfcon3/fcon3 mutant mice may indirectly affect the survival of cINs in a non-cell autonomous manner. We thus decided to restrict the Pcdhg loss of function to MGE/POA (preoptic area) progenitors by means of the Nkx2.1Cre mouse (Xu et al., 2008). MGE/POA progenitors give rise to the majority of mouse cINs, including PV and SST interneurons. NKX2.1 expression is detected in the ventral telencephalon from E9.5 (Sandberg et al., 2016; Shimamura et al., 1995) and is downregulated in most cINs as they migrate into the developing neocortex (Nóbrega-Pereira et al., 2008). Pcdhgfcon3 mice were crossed to Nkx2.1Cre mice. As described above, tdTomato expression was again used to visualize the recombined cells (Figure 3A). Homozygous Nkx2.1Cre;Ai14;Pcdhgfcon3/fcon3 embryos lost GFP expression specifically in in the MGE and the preoptic regions (Figure 3B), consistent with full recombination and loss of Pcdhg function in cells derived from the Nkx2.1 lineage.
At P30, the number of MGE-derived tdTomato+ cells in Nkx2.1Cre;Ai14;Pcdhgfcon3/fcon3 mice was dramatically reduced (~50%) in both the visual and somatosensory cortex (Figure 3C and C’). MGE-derived PV and SST interneuron number was similarly reduced in these animals. However CGE-derived VIP interneuron density was similar to that of control animals (Figure 3E and Figure 3—figure supplement 1). A smaller, but significant reduction in the RLN positive cIN population was observed, in agreement with the notion that a subpopulation of RLN cells is born in the MGE (Miyoshi et al., 2010). Consistently, layer 1 RLN+ cells, which are largely derived from the CGE (Miyoshi et al., 2010), were not affected by Pcdhg loss of function, but RNL cells in deeper layers 2–6 (many of which are MGE-derived and also positive for SST) showed reduced numbers (Figure 3—figure supplement 2). Interestingly, we observed that in our Nkx2.1Cre;Ai14;Pcdhgfcon3/fcon3 mice, the number of un-recombined PV and SST (PV+/tdTomato- and SST+/tdTomato-) cells was significantly increased compared to WT mice (Figure 3—figure supplement 4), a result consistent with recent observations (Carriere et al., 2020). PV+/tdTomato- and SST+/tdTomato- cells are likely derived from the most dorsal MGE at the interface with LGE expressing NKX6.2 in a region of low, or no expression of NKX2.1 (Hu et al., 2017a; Hu et al., 2017b; Fogarty et al., 2007; Sousa et al., 2009). We do not know if the presence of the conditional Pcdhgfcon3 allele results in increased production of these cells or if un-recombined cells from this domain increase their survival in compensation for the loss of cINs that lack Pcdhg function. If the latter is true, the behavior of these un-recombined PV and SST cINs differs from that observed for WT cells co-transplanted with MGE cells lacking Pcdhg function (see below). Together the above results show that embryonic loss of Pcdhg function in Nkx2.1-positive progenitors results in a significant reduction in the number of MGE/POA-derived cINs.
Pcdhg function is not required for the proliferation and migration of cIN precursors
The reduction in the number of cINs in Nkx2.1Cre;Ai14;Pcdhgfcon3/fcon3 mice was not a result of abnormal cortical thickness or abnormal layer distribution, as these measures were similar across genotypes in P30 mice (Figure 3C). Next, we asked whether migration or proliferation defects in the cIN progenitor population could lead to a reduced cIN density in Pcdhg mutant mice. Quantification of the number of dividing cells in the ventricular or subventricular zones at E13.5 and E15.5, using the mitotic marker Phosphohistone H3 (PH3), showed no difference in the number of mitotic cells in the MGE between Nkx2.1Cre;Ai14; Pcdhgfcon3/fcon3 mice and controls (Figure 4A and B). Migration of young cINs into cortex was also not affected in Nkx2.1Cre;Ai14;Pcdhgfcon3/fcon3 mice . The tdTomato+ cells in the cortex displayed a similar migratory morphology in Nkx2.1Cre;Ai14;Pcdhgfcon3/fcon3 embryos and controls. Consistent with the absence of an effect of Pcdhg on cIN migration, the number of migrating cells in cortex in the marginal zone (MZ), the subplate (SP), and the intermediate and subventricular zone (IZ/SVZ) was equivalent between Pcdhg mutant embryos and controls at E15.5 (Figure 4C and D). These findings indicate that loss of Pcdhg did not affect the proliferation of MGE progenitors or the migration of young MGE-derived cINs into the developing neocortex.
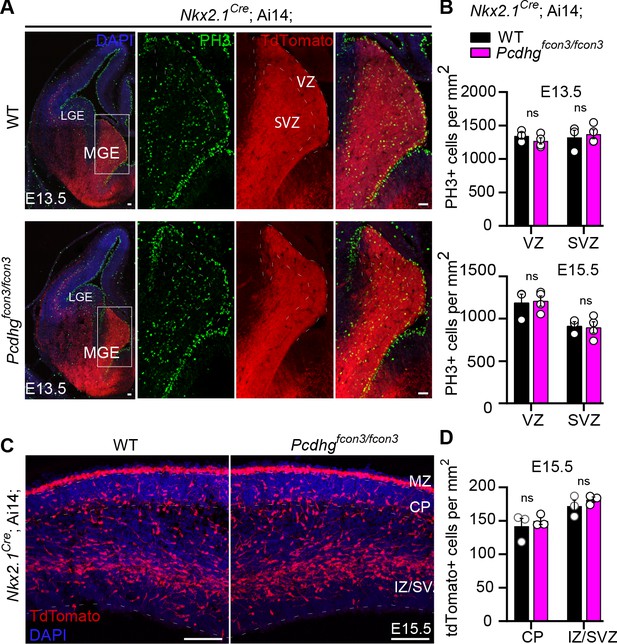
Proliferation and migration are not affected by the loss of Pcdhg in NKX2.1 expressing cells.
( A) Photographs of coronal sections through the embryonic forebrains of E13.5 Nkx2.1Cre;Ai14;Pcdhg+/+ (Pcdhg WT, top panels) and Nkx2.1Cre;Ai14;Pcdhgfcon3/fcon3 (Pcdhg mutant, bottom panels). Close-up photographs of the MGE from Nkx2.1Cre;Ai14;Pcdhg WT (right panels) and Pcdhg mutant (bottom, right panels) embryos. Robust reporter activity (tdTomato) was observed in the MGE. Dividing cells were labeled using the mitotic marker PH3. Note that the size and number of PH3+ cells in the MGE was similar in the mutant and control brains. Scale bars, 50 μm. (B) Quantification of PH3+ cells from MGE ventricular (VZ) and subventricular zone (SVZ) in E13.5 (top) and E15.5 (bottom) Nkx2.1Cre;Ai14;Pcdhg WT (black bars) and Pcdhg mutant (magenta bars) embryos (Mann-Whitney test, p=0.4000 (E13.5 VZ), p=0.8571 (E13.5 SVZ), p=0.8571 (E15.5 VZ), p>0.999 (E15.5 SVZ), n = 3–4 embryos of each genotype). (C) Photographs of coronal sections of dorsal cortex at E15.5 showing the migrating MGE-derived cIN in Nkx2.1Cre;Ai14;Pcdhg WT (left) and Pcdhg mutant (right) embryos. Note the robust migratory streams of young neurons in the SVZ and in the marginal zone (MZ). From these regions, cells disperse into the intermediate zone (IZ) and cortical plate (CP). Similar numbers of migrating cIN were observed in mutants and controls. Scale bar, 100 μm. (D) Quantifications of number of migrating MGE-derived cINs in the CP and in the IZ/SVZ of Nkx2.1Cre;Ai14;Pcdhg WT (black) and Pcdhg mutant (magenta) mice. No significant differences were detected in the number of tdTomato+ migrating cells in Pcdhg mutant and WT controls (Mann-Whitney test, p>0.990 (E15.5 CP), p=0.7000 (E15.5 IZ-SVA), n = 3 embryos of each genotype).
-
Figure 4—source data 1
Quantification of PH3 positive cells in the embryonic MGE and number of Nkx2.1-derived cINs in the embryonic dorsal cortex of controls and Pcdhg loss of function mice.
- https://cdn.elifesciences.org/articles/55374/elife-55374-fig4-data1-v2.xlsx
Accentuated cIN cell death in Pcdhg mutants
A wave of programmed cell death eliminates ~40% of the young cINs shortly after their arrival in the cortex (Southwell et al., 2012; Wong et al., 2018). This wave starts at ~P0, peaks at P7, and ends at ~P15. Next, we asked if the reduced cIN density observed in Pcdhg mutant mice could stem from a heightened number of mutant cINs undergoing apoptosis at the normal time. Such cells were immunolabeled using an antibody directed against cleaved-Caspase 3 (CC3). Since CC3 positive cells are relatively rare, our analysis was performed throughout the entire neocortex, at P0, 3, 7, 10, and 15. Similarly to their wild type littermates, Nkx2.1Cre;Ai14;Pcdhgfcon3/fcon3 homozygous mice displayed a wave of programmed cell death peaking at P7 (Figure 5A and B). However, Pcdhg mutant mice had significantly higher numbers of tdTomato+/CC3+ cells compared to controls. We also examined the proportion of CC3+ cells that were tdTomato negative (un-recombined cells that would notably include pyramidal cells, CGE-derived cINs, and glial cells). With the exception of a small, but significant increase observed at P0, we found no significant difference in the number of CC3+/tdTomato- cells between genotypes (Figure 5B, bottom graph). This suggests that the survival of neighboring Pcdhg-expressing cells is not impacted by the loss of Pcdhg-deficient MGE/POA-derived cINs. Importantly, the homozygous deletion of the pro-apoptotic Bcl-2-associated X protein (BAX) rescued cIN density in the Pcdhg mutant mice to levels similar to those observed in control Bax-/- ;Pcdhfcon3/+ mice or in mice carrying only the Bax mutation (Bax-/-) (Southwell et al., 2012; Figure 5C). The above results indicate that loss of Pcdhg in MGE/POA-derived cIN enhances their demise through programmed cell death during the developmental period when these cells are normally eliminated.
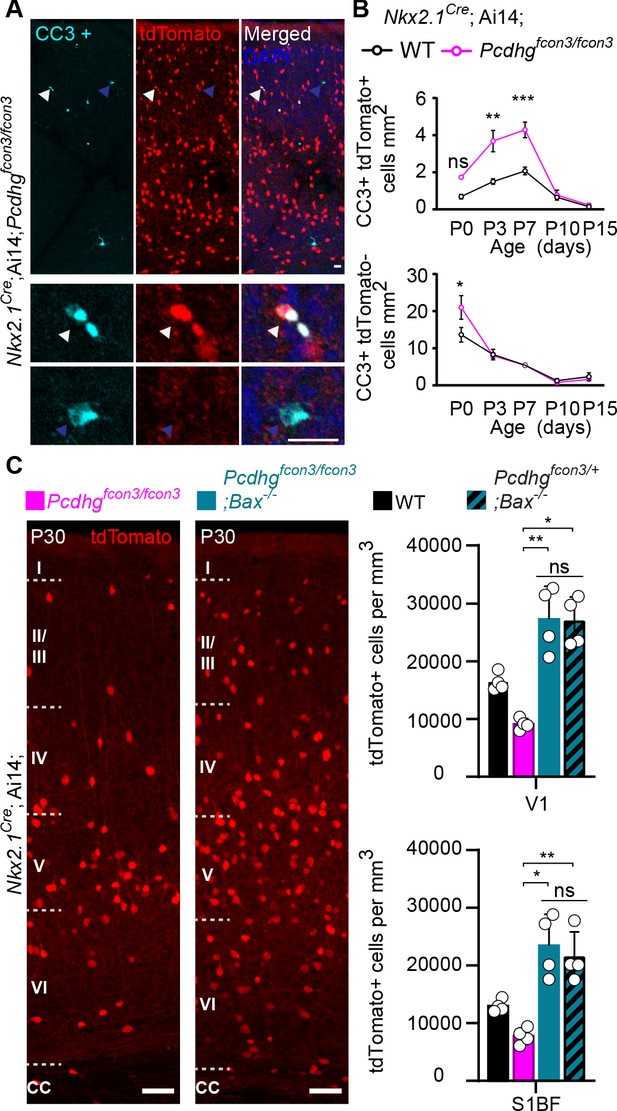
Increased programmed cell death in Pcdhg mutants is rescued in Pcdhg-bax null animals.
(A) Photographs of coronal sections through a Nkx2.1Cre;Ai14;Pcdhgfcon3/fcon3 (Pcdhg mutant) P7 mouse cortex (top), showing tdTomato+ cINs and cleaved caspase 3 positive cells (CC3+). Close-up photographs (bottom) of tdTomato+, CC3+ (white Arrowheads) and tdTomato-, CC3+ (blue Arrowheads) cells. Scale bar 25 μm. (B) Quantification of the density of tdTomato+,CC3+ (MGE-derived, top graph) cells from Nkx2.1Cre;Ai14;Pcdhg WT (black line) and Pcdhg mutant (magenta line) mice. Quantification of the density of tdTomato-,CC3+ (non-MGE-derived, bottom graph) cells from Nkx2.1Cre;Ai14;Pcdhg WT (black line) and Pcdhg mutant (magenta line) mice. Note that the number of CC3+ cells was significantly increased in the MGE-derived population in Pcdhg mutant mice, and coincides with the normal period of programmed cell death for cINs in WT mice (Each age was analyzed with a nested 1-way ANOVA (mouse ID nested within genotype), P value<0.0001. Significant comparisons are marked with *; *p=0.0004 **p=0.0014, ***p=0.0009, n = 3–5 mice of each genotype). (C) Coronal sections through the primary visual cortex (V1) of Nkx2.1Cre;Ai14;Pcdhgfcon3/fcon3 (Pcdhg mutant, left) and Nkx2.1Cre;Ai14;Pcdhgfcon3/fcon3;Bax-/- (Pcdhg mutant, Bax null, right) mice at P30. Quantifications of the density of cINs in V1 (top) and S1BF (bottom) cortex. Note that genetic removal of Bax in both Pcdhgfcon3/+ (Pcdhg HET) and Pcdhgfcon3/fcon3 (Pcdhg mutant) mice rescues cell death to similar levels (Kruskal-Wallis test, P value<0.001 (for V1 an S1BF), adjusted p values for V1 (*p=0.01 , **0.005 ) and for S1BF (**p=0.0109 , *p=0.0286); n = 4–5 mice of each genotype).
-
Figure 5—source data 1
Analysis of cIN programmed cell death in controls, Pcdhg mutant and Bax null mice.
- https://cdn.elifesciences.org/articles/55374/elife-55374-fig5-data1-v2.xlsx
Loss of Pcdhg does not affect survival of cINs after the period of programmed cell death
We then asked whether Pcdhg-expression is also required for the survival of cINs past the period of programmed cell death. To address this question we took advantage of the PVCre transgene (Hippenmeyer et al., 2005) that becomes activated specifically in PV interneurons starting at around ~P16 (Figure 6 and Figure 6—figure supplement 1). Quantifications of tdTomato+ cell density in PVCre;Ai14;Pcdhgfcon3/fcon3 and PVCre;Ai14 mice at P60-P100 revealed no significant differences between homozygous and control mice (V1 and S1BF) (Figure 6D and E). In contrast, the SstCre line, like Nkx2.1Cre, induces recombination at embryonic stages. The SstCre allele in Ai14; Pcdhgfcon3/fcon3 reduced the density of SST interneurons measured at P30 to the same level as was found using the Nkx2.1Cre line (Figure 6A–C). Together, our results demonstrate that Pcdhg loss of function reduces cIN survival specifically during the endogenous period of cIN cell death, resulting in a reduced cortical density of cINs.
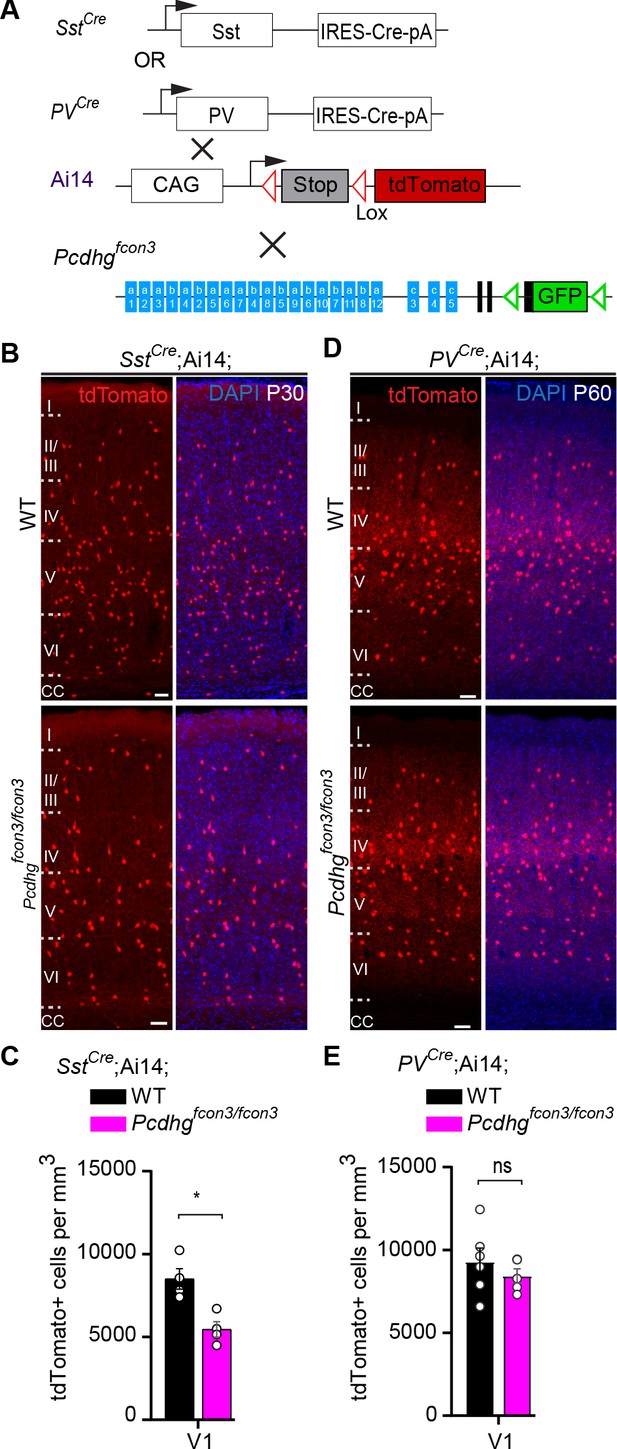
Pcdhg function is not required for the survival of PV cINs after the period of programmed cell death.
(A) Mutant mice with loss of Pcdhg in SST or PV cells were generated by crossing conditional Pcdhgfcon3 mice to mice carrying Cre under Sst (SstCre) or Pvalb (Parvalbumin, PVCre). The conditional Ai14 line was used to fluorescently label SST or PV cells. (B) Photographs of coronal sections of the primary visual cortex (V1) of P30 SstCre; Ai14;Pcdhg+/+ (Pcdhg WT, top left) and SstCre;Ai14;Pcdhgfcon3/fcon3 (Pcdhg mutant, bottom left) mice. Scale bars, 50 μm. (C) Quantifications of the density of tdTomato+ cINs in V1 cortex of Pcdhg WT (black) and Pcdhg mutant (magenta) SstCre;Ai14 mice at P30 (Mann-Whitney test, **p=0.0286, n = 4 mice of each genotype). (D) Photographs of coronal sections of V1 in PVCre;Ai14;Pcdhg+/+ (Pcdhg WT, top right) and PVCre;Ai14;Pcdhgfcon3/fcon3 (Pcdhg mutant, bottom right) mice at P60. Scale bars, 50 μm. (E) Quantifications of the density of tdTomato+ cIN in V1 cortex of Pcdhg WT and Pcdhg mutant PVCre;Ai14 mice at P60-100 (Mann-Whitney test, p=0.4206,n = 5 mice of each genotype).
-
Figure 6—source data 1
Analysis of PV and SST- derived cINs at P30 in controls and Pcdhg mice.
- https://cdn.elifesciences.org/articles/55374/elife-55374-fig6-data1-v2.xlsx
Pcdha and Pcdhb do not affect cIN survival
Previous studies indicate that Pcdhs form tetrameric units that include members of the alpha, beta, and gamma clusters (Schreiner and Weiner, 2010; Aye et al., 2014). We, therefore asked whether Pcdha and Pcdhb genes also contribute to cIN cell death. Mice that carry a conditional deletion of the entire alpha cluster (Pcdhaacon/acon) were crossed to the Nkx2.1Cre;Ai14 line, resulting in removal of the Pcdha genes specifically from MGE/POA progenitor cells (Figure 7A). Nkx2.1Cre;Ai14;Pcdhaacon/acon mice were viable, fertile, and displayed normal weight (Figure 7B, top graph). cIN density in the visual cortex of Nkx2.1Cre;Ai14;Pcdhaacon/acon mice at P30 was similar to that of Nkx2.1Cre;Ai14 mice (Figure 7B). To determine whether Pcdhb genes affected MGE/POA-derived cIN survival, constitutive Pcdhb gene cluster knockout (Pcdhbdel/del) mice were crossed to Nkx2.1Cre;Ai14 mice (Figure 7A). Mice carrying a deletion of the entire Pcdhb cluster were viable, fertile and of normal weight (Figure 7C, top graph) (Chen et al., 2017). The density of cINs was similar between mice lacking Pcdhb and controls (Figure 7C). The above results indicate that unlike the Pcdhg cluster, which is essential for the regulation of cIN elimination, the function of Pcdha or Pcdhb is dispensable for the survival of MGE/POA-derived cINs.
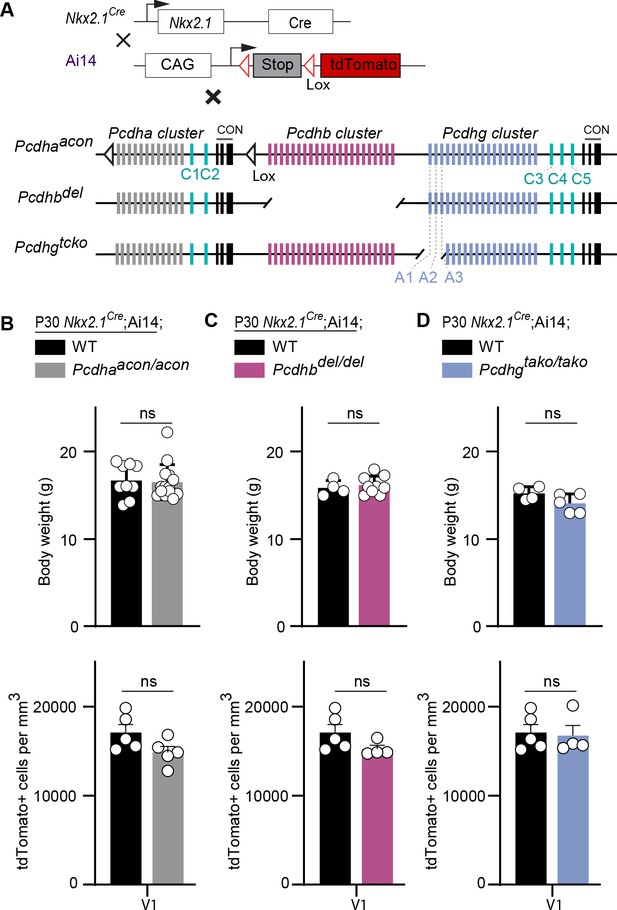
Loss of Pcdha, Pcdhb, or Pcdhga1, Pcdhga2, and Pcdhga3 genes does not affect the survival of MGE-derived cINs.
(A) Mutant mice with loss of Pcdha, Pcdhb or Pcdhga1, Pcdhga2, and Pcdhga3 genes in MGE-derived cINs were generated by crossing Pcdhaacon, Pcdhbdel, and Pcdhgtako mice to the Nkx2.1Cre mouse line. The conditional Ai14 line was used to fluorescently label MGE-derived cells. (B) Measurements of body weight (top graph) in P30 Nkx2.1Cre;Ai14;Pcdha+/+ (Pcdha WT, black bar) and Nkx2.1Cre;Ai14;Pcdhaacon/acon (Pcdha mutant, grey bar) mice (Mann-Whitney test, p=0.545, n = 9–14 mice of each genotype). Quantification of the density of MGE-derived cINs (bottom graph) in primary visual cortex (V1) of Pcdha WT (black bar) and Pcdha mutant (grey bar) P30 mice (Mann-Whitney test, p=0.9603, n = 4–5 mice of each genotype). (C) Measurements of body weight (top bar) in P30 Nkx2.1Cre;Ai14;Pcdhb+/+ (Pcdhb WT, black bar) and Nkx2.1Cre;Ai14;Pcdhbdel/del (Pcdhb mutant, pink bar) mice (Mann-Whitney test, p=0.712, n = 4–9 mice of each genotype). Quantification of the density of MGE-derived cIN (bottom graph) in primary visual cortex (V1) of Pcdhb WT (black bar) and Pcdhb mutant (pink bar) P30 mice (Mann-Whitney test, p=0.1111, n = 4–5 mice of each genotype). (D) Measurements of body weight (top graph) in Nkx2.1Cre;Ai14;Pcdhg+/+ (Pcdhg WT, black) and Nkx2.1Cre;Ai14;Pcdhgtako/tako(Pcdhga1, Pcdhga2, and Pcdhga3 mutant, blue bar) P30 mice (Mann-Whitney test, p=0.175, n = 4–5 mice of each genotype). Quantification of the density of MGE-derived cINs (bottom graph) in in primary visual cortex (V1) of Pcdhg WT (black bar) and Pcdhga1, Pcdhga2, and Pcdhga3 mutant (blue bar) P30 mice (Mann-Whitney test, p=0.9048, n = 4–5 mice of each genotype).
-
Figure 7—source data 1
Analysis of Nkx2.1-derived cINs in P30 control, Pcdha, Pcdhb and Pcdhg mutant mice.
- https://cdn.elifesciences.org/articles/55374/elife-55374-fig7-data1-v2.xlsx
Loss of Pcdhg does not affect cIN dispersion after transplantation but affects their survival
In order to compare the timing and extent of migration, survival, and maturation of cINs of different genotypes within the same environment, we co-transplanted into the cortex of host animals, MGE-derived cIN precursor cells expressing red and green fluorescent proteins. MGE cIN precursors were derived from E13.5 Gad1-GFP embryos (Pcdhg WT controls) or from Nkx2.1Cre;Ai14 embryos that were either Pcdhg WT or Pcdhg mutant (Figure 8A). We first confirmed that MGE cells WT for Pcdhg, but carrying the two different fluorescent reporters, displayed no differences in their survival. Equal proportions of Gad1-GFP cells (Pcdhg WT GFP+) and Nkx2.1Cre;Ai14 cells (Pcdhg WT tdTomato+) were co-transplanted into the neocortex of neonatal recipients. While equivalent numbers of red and green cells were mixed before being transplanted, the absolute number of cells transplanted varied from transplant to transplant. In order to compare the survival, we use the fraction of green or red cells, among all co-transplanted cells (red + green). The fraction of surviving GFP+ and tdTomato+ cells at 3, 6, 13, and 21 days after transplantation (DAT) was measured (Figure 8A and B, top graph). The contribution of each cell population to the overall pool of surviving cells was found to be ~50% at 3 DAT, and remained constant at 6, 13, and 21 DAT (Figure 8B, top graph). This experiment indicates that the fluorescent reporters (GFP or tdTomato) or breeding background does not affect the survival of MGE cINs in this assay. Next, we co-transplanted equal numbers of Gad1-GFP cells (Pcdhg WT) and Nkx2.1Cre;Ai14;Pcdhgfcon3/fcon3 cells (Pcdhg mutant) into the cortex of WT neonatal recipients. As above, we measured the proportion of surviving GFP+ and tdTomato+ cells at 3, 6, 13, and 21 DAT (Figure 8A). Similar numbers of GFP+ and tdTomato+ cells were observed at 3 and 6 DAT. However, the fraction of Pcdhg mutant cINs (tdTomato+) surviving was dramatically lower when the transplanted cells reached a cellular age equivalent to that of endogenous cINs after the normal wave of programmed cell death (6DAT is roughly equivalent to P0; 21DAT is roughly equivalent to P15) (Southwell et al., 2012). Note that in this experiment the proportion of WT cells increases during this same period. This change in proportion is not a reflection of increased survival, as these cells also undergo elimination by programmed cell death (see below) (Figure 8—figure supplement 1), but that, with the increased loss of mutant cells, the WT cells account for a larger fraction of the total.
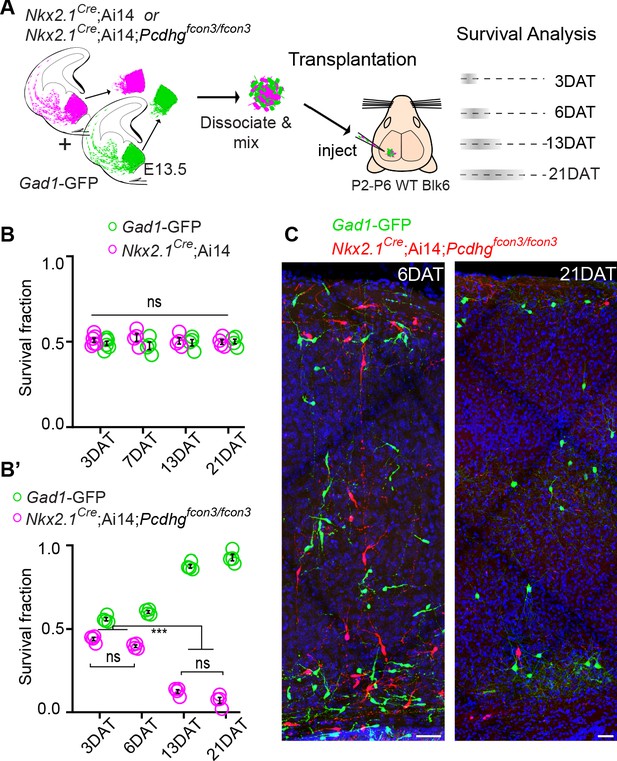
Pcdhg are required for cIN survival after transplantation.
(A) Schematic of co-transplantation of MGE-derived cIN precursors. MGE cells were derived from Nkx2.1Cre;Ai14;Pcdhg+/+ (Pcdhg WT) or Nkx2.1Cre;Ai14;Pcdhgfcon3/fcon3 (Pcdhg mutant) embryos. These cells were mixed in equal proportions with MGE cells from Gad1-GFP embryos (Pcdhg WT, green) and transplanted into WT black (Blk) six host recipient mice. Cell survival was analyzed before (3 DAT) and throughout the period of cell death (6–21 DAT). (B,B’) Survival fraction of co-transplanted MGE-derived cIN precursors. (B) MGE cells were derived from Gad1-GFP (green) and Nkx2.1Cre;Ai14; (magenta) embryos; both GFP+ and tdTomato+ cells carry WT Pcdhg. In this control experiment the survival fraction was similar for both genotypes carrying the different fluorescent reporters (2-way ANOVA, Fgenotype = 2.54, P value > 0.999; n = 4–6 mice per time point from two transplant cohorts). (B’) MGE cells were derived from Gad1-GFP WT (green) and Nkx2.1Cre;Ai14;Pcdhg mutant (magenta) embryos. GFP+ and tdTomato+ cells showed dramatic differences in their survival; the majority of cells carrying the Pcdhg mutant allele (magenta) were eliminated between 6 and 21 DAT (2-way ANOVA, Fgenotype = 2738.02, P value < 0.0001; adjusted p values ***p<0.0001; n = 4–5 mice per time point from two transplant cohorts. Quantifications in (B and B’) were done at 3, 6, 13 and 21 DAT and are represented as fractions of GFP+ or tdTomato+ cells from total cells (GFP + tdTomato+) per brain section. The increase in the proportion of WT cells during this period is not a reflection of increased cell numbers (WT cIN also undergo elimination by programmed cell death (See Figure 8—figure supplement 1), but rather that WT cells account for a larger fraction of all transplant-derived cells (WT + Pcdhg mutant). (C) Representative photographs of cortical sections from transplanted host mice at 6 (left) and 21 (right) DAT. Transplanted MGE cells were derived from Gad1-GFP (Pcdhg WT, green) and Nkx2.1Cre;Ai14;Pcdhgfcon3/fcon3 (Pcdhg mutant, red) embryos. Scale bars, 50 μm.
-
Figure 8—source data 1
Survival of transplanted MGE-derived cIN precursor cells carrying WT or mutant Pcdhg.
- https://cdn.elifesciences.org/articles/55374/elife-55374-fig8-data1-v2.xlsx
We next determined whether the survival of Pcdhg WT (GFP+) or Pcdhg mutant (tdTomato+) cINs was affected by their density (Figure 9). At 6 DAT, WT and Pcdhg mutant MGE-derived cells had migrated away from the injection site establishing a bell-shaped distribution of density as a function of tangential distance from the injection site (Figure 9B and B’). The dispersion of developing cINs lacking Pcdhg was indistinguishable from that of control WT cells at this time (Figure B’, top graph), consistent with our observation that Pcdhg expression is not required for the migration of MGE-derived cINs. Strikingly, the survival fraction at 6 DAT of control Pcdhg WT (GFP+) and Pcdhg mutant (Nkx2.1Cre;Ai14 ;Pcdhgfcon3/fcon3) cINs at the injection site or at multiple locations anterior or posterior to the site of injection were also similar (Figure 9B’, bottom graph). By 21 DAT the survival of Pcdhg mutant (Nkx2.1Cre;Ai14 ;Pcdhgfcon3/fcon3) cells was dramatically reduced, and to a similar extent at all distances from the injection site (Figure 9B and B’). Since the density of cIN varies fivefold over regions measured, we conclude that the survival of control Pcdhg WT and Pcdhg mutant cIN does not depend on their density over this range.
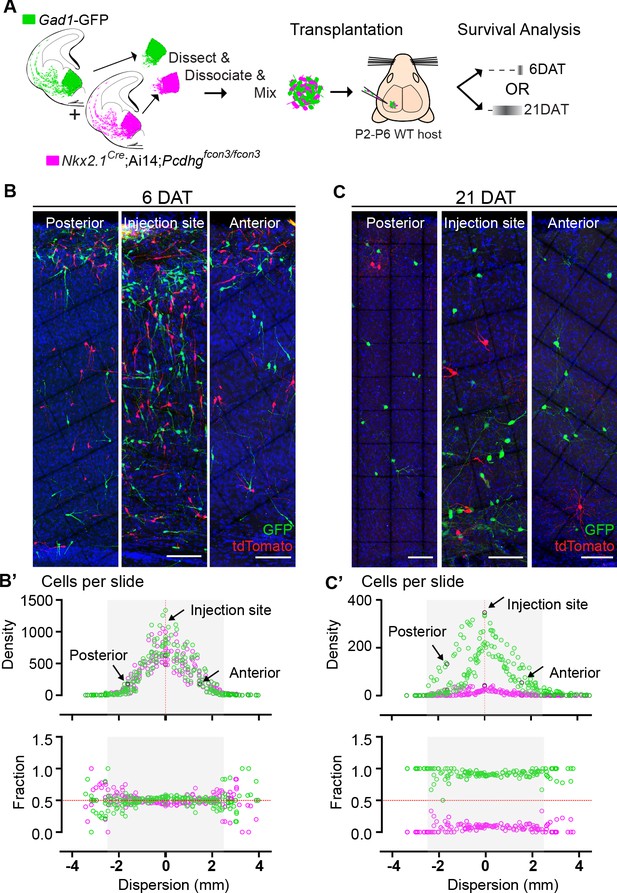
Survival of cIN, WT or mutant for Pcdhg, was not affected by cell density.
(A) MGE cells derived from Nkx2.1Cre;Ai14;Pcdhgfcon3/fcon3 embryos (Pcdhg mutant, magenta) and from Gad1-GFP embryos (Pcdhg WT, green) were mixed in equal numbers and transplanted into WT hosts. The survival of tdTomato and GFP- labeled cINs was analyzed in every other section throughout the brain region of the transplant dispersal. (B) Photographs of representative coronal sections at the injection site, or anterior and posterior to it, from host mice at 6DAT. Similar numbers of tdTomato and GFP-labeled cINs were observed at each location. Scale bar 100 μm. (B’) Dispersion analysis at 6 DAT of the Pcdhg WT (green) or Pcdhg mutant (magenta) cells, represented as density (top) or survival fraction (bottom) as a function of distance from the site of injection in the host recipients. Note that the density of cells decreases as one moves anteriorly or posteriorly with respect to the injection site. At 6 DAT, the dispersal and survival was similar for both WT and Pcdhg mutant cells. (C) Photographs of representative coronal sections at the injection site, or anterior and posterior to it, from host mice at 21DAT. Note the dramatic reduction in the number of Pcdhg mutant cells (magenta) compared to the Pcdhg WT cells (green). Scale bar 100 μm. (C’) Dispersion analysis at 21DAT of the Pcdhg WT (green) or Pcdhg mutant (magenta) cells, represented as density (top) or survival fraction (bottom) as a function of distance from the site of injection in the host recipients. At 21 DAT, the survival fraction for the Pcdhg mutant cells (magenta) was dramatically reduced and similarly affected at different locations with respect to the injection site.
-
Figure 9—source data 1
Dispersal and survival analysis of transplanted MGE-derived cIN precursor cells carrying WT or mutant Pcdhg.
- https://cdn.elifesciences.org/articles/55374/elife-55374-fig9-data1-v2.xlsx
In order to determine the absolute number of cINs eliminated in our co-transplantation experiments, we co-transplanted 50 K cells of each genotype (Pcdhg WT and Pcdhg mutant) into WT host mice (Figure 10A). Our baseline for survival was established at 6 DAT, before the period of cIN programmed cell death. In control experiments where cIN precursors WT for the Pcdhg allele, derived from Nkx2.1Cre;Ai14 or Gad1-GFP embryos, were transplanted, 39% of the transplanted cIN population was eliminated between 6 and 21 DAT (Figure 10A–C and Figure 10—figure supplement 1). Therefore, transplanted MGE cINs not only undergo programmed cell death during a period defined by their intrinsic cellular age but are also eliminated in a proportion that is strikingly similar to that observed during normal development (Wong et al., 2018; Southwell et al., 2012). Given these observations, we next asked how the presence of Pcdhg mutant cIN affected the survival of WT cIN in the transplantation setting. We co-transplanted 50K Gad1-GFP Pcdhg WT (GFP+) with 50K Nkx2.1Cre;Ai14 Pcdhg mutant (tdTomato+) MGE cIN precursors and compared the survival of each population at 6 and 21 DAT. At 6 DAT the total number of tdTomato+ cells in the cortex of recipient mice was similar to that of GFP+ cells (Figure 10A,D and E). However, between 6 and 21DAT, the total number of GFP+ cells had decreased by an average of ~63% (Figure 10E, compared to Figure 10C). Compared to the ~40% of endogenous or transplanted WT cINs that are normally eliminated (present study and previous work Southwell et al., 2012; Wong et al., 2018), this experiment suggests that WT cells die at a higher rate (63%) when co-transplanted with Pcdhg mutant MGE cells. However, this observation would require additional animals for statistical confirmation. Regardless, the number of Pcdhg mutant (tdTomato+ cells) cINs decreased dramatically, by ~96% (Figure 10E). This experiment confirms that MGE cells lacking Pcdhg function are eliminated in far greater numbers than control MGE cells and show that the presence of Pcdhg WT cINs within a mixed population also affects the survival of mutant cINs (compare Figure 8 and Figure 10). These observations are consistent with the hypothesis that cINs interact with others of the same age discussed below.
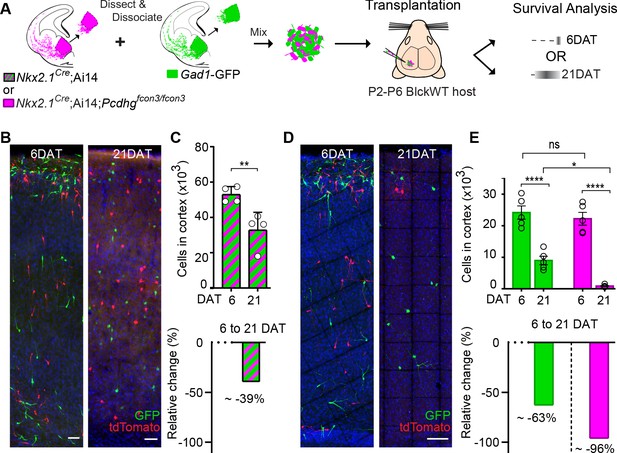
MGE cell transplantation reveals a non-cell autonomous effect of Pcdhg on cIN survival.
(A) Schematic of co-transplantation experiment for quantification of absolute number of transplanted MGE cells derived from (1) Nkx2.1Cre;Ai14 and Gad1-GFP embryos WT for Pcdhg or (2) Nkx2.1Cre;Ai14;Pcdhgfcon3/fcon3 (Pcdhg mutant, magenta) and Gad1-GFP (Pcdhg WT, green) embryos. The total numbers of transplant-derived cINs were counted at 6 and 21 DAT throughout the volume of cortex were transplanted cells dispersed. (B) Photographs of representative coronal sections of co-transplanted tdTomato and GFP-labeled cells, both Pcdhg WT, at 6 and 21 DAT. Scale bar 100 um. (C) Absolute number of surviving tdTomato and GFP-labeled Pcdhg WT cIN at 6 and 21 DAT (top graph) (Mann-Whitney test, **p=0.0286, n = 4 mice per time point from one transplant cohort). The drop in number of transplant derived cells was similar for WT-GFP+ and WT-tdTomato+ (Figure 10—figure supplement 1). A 39% drop in cIN number was observed between 6 and 21 DAT (bottom graph). (D) Photographs of representative coronal sections of transplanted tdTomato-labeled Pcdhg mutant (magenta) and GFP-labeled Pcdhg WT (green) cells at 6 and 21 DAT. Survival of the cINs drops for both genotypes, but the tdTomato-labeled cells were nearly eliminated by 21 DAT. Scale bar 100 μm. (E) Absolute number of surviving cINs at 6 and 21 DAT (top graph)(2-way ANOVA; Fage = 128.65, P value < 0.0001, adjusted p value ****p<0.0001); Fgenotype = 9.74 (p value=0.0066, adjusted p value *p=0.0126); n = 5 mice per time point from one transplant cohort). Comparing 6 and 21 DAT a drop of ~63% and of 96.0% was observed, respectively, for cells WT and mutant for Pcdhg (bottom graph).
-
Figure 10—source data 1
Survival analysis and absolute quantification of transplanted MGE-derived cIN precursor cells carrying WT or mutant Pcdhg.
- https://cdn.elifesciences.org/articles/55374/elife-55374-fig10-data1-v2.xlsx
Loss of Pcdhg isoforms Pcdhgc3, Pcdhgc4, and Pcdhgc5 is sufficient to increase cell death
The results above indicate that the loss of function of all 22 Pcdh isoforms encoded from the Pcdhg gene cluster significantly increased cell death among cINs. Whether all 22 Pcdhg are equally involved in the regulation of cIN survival remains unclear. Our qPCR expression analysis suggests that the expression of Pcdhga1, Pcdha2, Pcdhgc4 and Pcdhgc5 in cINs increases during, or soon after, the period of cell death (Figure 1C). To test if Pcdhga1, Pcdhga2, and Pcdhga3 were required for the normal survival of MGE-derived cINs, we crossed the Pcdhgtako/tako mouse line (Pcdhga1, Pcdhga2, and Pcdhga3 isoform KO) to Nkx2.1Cre; Ai14 mice (Figure 7A). At P30, the density of cINs in visual cortex of Nkx2.1Cre;Ai14;Pcdhgtako/tako (Pcdhga1, Pcdhga2, and Pcdhga3 mutant) mice was not significantly different from that of control Nkx2.1Cre;Ai14 mice that are WT for Pcdhg (Figure 7D). Consistent with this finding, co-transplanted E13.5 Nkx2.1Cre; Ai14;Pcdhgtako/tako MGE cells and Pcdhg WT (GFP+) displayed surviving fractions of similar sizes (Figure 11A,B & B’). Therefore, the removal of the first three isoforms (Pcdga1, Pcdhga2 and Pcdhga3) of the Pcdhg cluster does not significantly affect cIN survival.
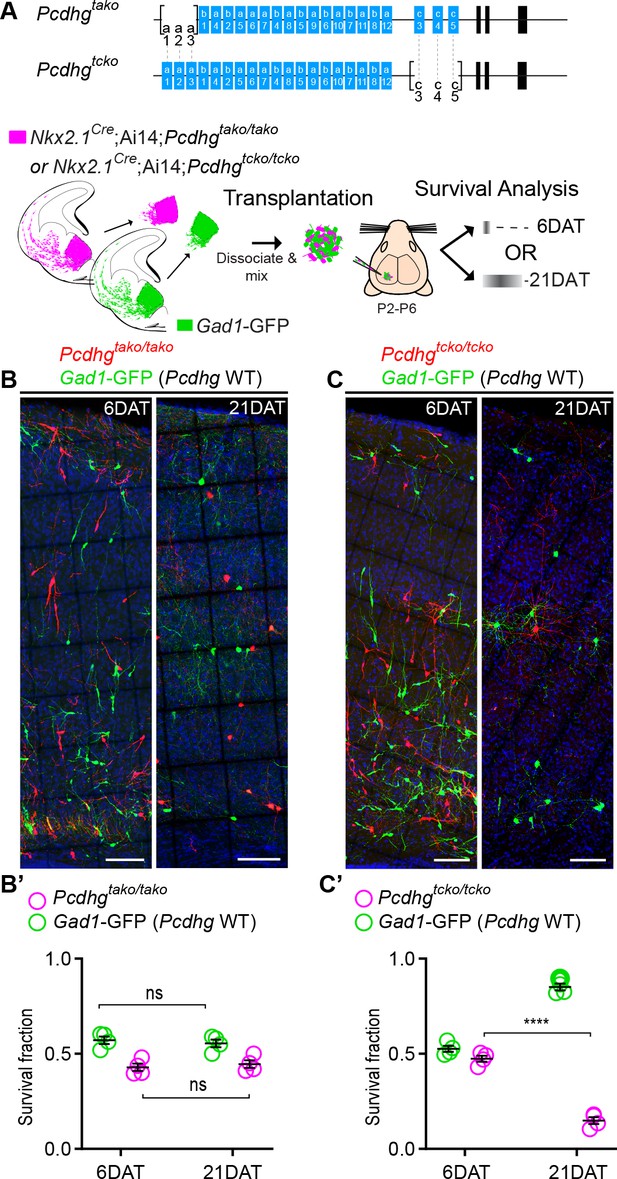
Loss of Pcdhgc3, Pcdhgc4, and Pcdhgc5 is sufficient to increase cIN cell death.
(A) Diagram of the mutant alleles Pcdhgtako (Pcdhga1, Pcdhga2, and Pcdhga3 KO) and Pcdhgtcko (Pcdhgc3, Pcdhgc4, and Pcdhgc5 KO). Below - schematic of transplantation of MGE cIN precursors from Nkx2.1Cre;Ai14;Pcdhgtako/tako (Pcdhga1, Pcdhga2, and Pcdhga3 deleted) and Nkx2.1Cre;Ai14;Pcdhgtcko/tcko (Pcdhgc3, Pcdhgc4, and Pcdhgc5 deleted) embryos. These cells were mixed in equal proportions with MGE cells from Gad1-GFP embryos (Pcdhg WT, green) and transplanted into WT Blk6 host recipients. Survival of the GFP and tdTomato-labeled cells was analyzed at 6 and 21 DAT. (B, B’) Representative photographs of cortical sections from transplanted host animals at 6 (left) and 21 (right) DAT. Note the similar proportions of Pcdhg WT (GFP+) and Pcdhga1, Pcdhga2, and Pcdhga3 deleted cells (tdTomato+) at 6 and 21DAT. Scale bars, 100 μm. (B’) Quantifications of the survival fraction of the GFP (green) and tdTomato (magenta)-labeled MGE-derived cells at 6 and 21 DAT. Note, survival fraction remains similar and constant for both genotypes (Pcdhg WT and Pcdhga1, Pcdhga2, and Pcdhga3 deleted cells) between 6 and 21 DAT (Mann-Whitney test, p=6571, n = 4 mice per time point from one transplant cohort). (C, C’) Representative photographs from coronal brain sections of transplanted host animals at 6 (left) and 21 (right) DAT. Scale bars, 100 μm. Survival of MGE-derived cINs from Pcdhgc3, Pcdhgc4, and Pcdhgc5 deleted embryos (tdTomato+) is markedly different from MGE-derived cINs from Pcdhg WT embryos (GFP+). (C’) Survival fraction at 6 and 21 DAT of the Pcdhg WT (green) and Pcdhgc3, Pcdhgc4, and Pcdhgc5 deleted cells (magenta) (Mann-Whitney test, ****p=0.0286, n = 4 mice per time point from one transplant cohort).
-
Figure 11—source data 1
Survival analysis of transplanted MGE-derived cIN precursor cells deficient in Pcdha1, Pcdha2 and Pcdha3 or deficient in Pcdhc3, Pcdhc4 and Pcdhc5.
- https://cdn.elifesciences.org/articles/55374/elife-55374-fig11-data1-v2.xlsx
We next tested if removal of the last three isoforms of the Pcdhg cluster (Pcdhgc3, Pcdhgc4, and Pcdhgc5) affected cIN survival. Pcdhgtcko/+ mice, heterozygous for excision of Pcdhgc3, Pcdhgc4, and Pcdhgc5 were crossed to the Nkx2.1Cre;Ai14 mouse line to label MGE/POA-derived cINs (Figure 11A). Even though homozygous Nkx2.1Cre;Ai14 ;Pcdhgtcko/tcko mice develop normally (normal weight and no evidence of brain abnormalities) and are born in normal Mendelian ratios, these mice die shortly after birth (Chen et al., 2012). To bypass neonatal lethality, and study the role of Pcdhgc3, Pcdhgc4, and Pcdhgc5 isoforms during the normal period of cIN programmed cell death, we co-transplanted Pcdhgtcko/tcko (tdTomato+, mutant) and Pcdhg WT (GFP+) E13.5 MGE cells into the cortex of WT neonatal recipients (Figure 11A). At 6 DAT, the dispersion and density of tdTomato+ and GFP+ cells were indistinguishable. However, the number of tdTomato+ MGE-derived Pcdhgtcko/tcko mutant cINs dropped dramatically between 6 and 21 DAT, compared to the Pcdhg WT (GFP+) population (Figure 11C). The survival of Pcdhgtcko/tcko mutant cIN at 21 DAT was strikingly similar to that observed after transplantation of MGE cells lacking the entire Pcdhg cluster (Nkx2.1Cre;Ai14;Pcdhgfcon3/fcon3); compare Figure 8 and Figure 11. These results indicate that unlike Pcdga1, Pcdhga2 and Pcdhga3 isoforms, Pcdhgc3, Pcdhgc4, and Pcdhgc5 are essential for cIN survival.
Morphological and physiological maturation of cINs lacking Pcdhg
The above results indicate that cINs lacking Pcdhg genes have increased cell death, specifically when the transplanted cells reach an age equivalent to that of endogenous cINs undergoing their normal period of programmed cell death. We therefore asked whether the loss of Pcdhg in cINs affected their morphological maturation during this period. We first determined the survival fraction for co-transplanted control Gad1-GFP (Pcdhg WT) and Nkx2.1Cre;Ai14;Pcdhgfcon3/fcon3 (Pcdhg mutant) MGE-derived cIN precursors at two-day intervals during the intrinsic period of cIN cell death in the transplanted population (6, 8, 10 and 12 DAT). When equal proportions of Pcdhg WT and Pcdhg mutant cells were co-transplanted, their survival fraction remained similar up to 6 DAT, but the proportion of the Pcdhg mutant cells dropped steadily throughout the period of cell death (Figure 12B). Morphological reconstructions of the transplanted cells during this period of cIN programmed cell death (Figure 12A) revealed no obvious differences between the Pcdhg mutant and control Pcdhg WT cells in neuritic complexity, including neurite length (Figure 12C), the number of neurites (Figure 12D), number of nodes (Figure 12E) and number of neurite ends (Figure 12F). These results suggest that Pcdhg genes do not play a major role in the morphological maturation of cINs during the period of cIN death.
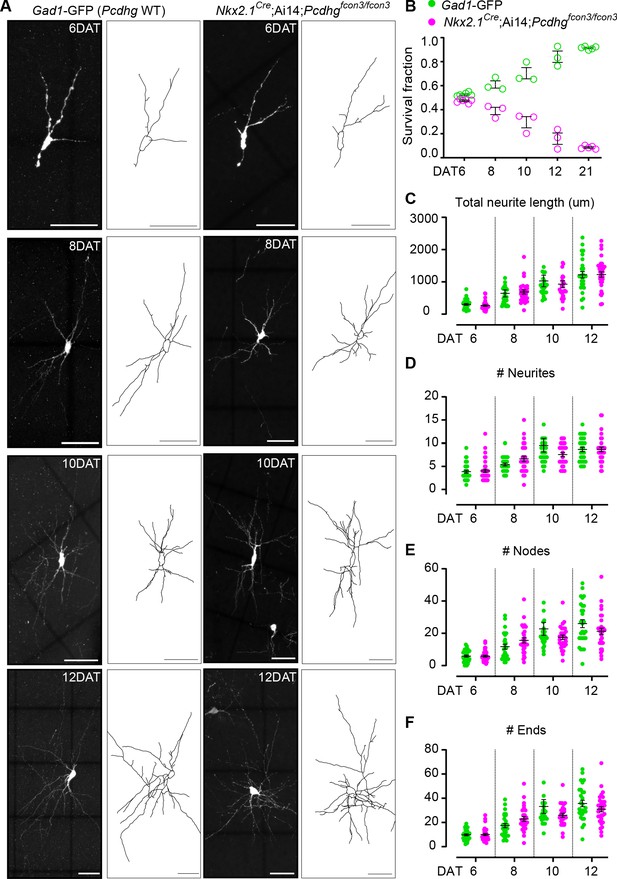
Loss of Pcdhg does not affect the morphological maturation of cIN during the period of programmed cell death.
(A) Photographs of representative images and morphological reconstructions of co-transplanted Gad1-GFP cells (Pcdhg WT, left columns) with Nkx2.1Cre;Ai14;Pcdhgfcon3/fcon3 cells (Pcdhg mutant, right columns) at 6, 8, 10 and 12DAT. Scale bars, 50 μm. (B) Quantifications of Pcdhg WT(green) and Pcdhg mutant cells (magenta) from co-transplanted animals, represented as survival fraction from total number of cells per section at 6, 8 10 12 and 21 DAT. Pcdhg mutant cells begin to increase their elimination between 6 and 8 DAT and this increased death occurs through 21 DAT. (C–F) Measurements of neurite complexity during the period of programmed cell death, including neurite length (C), neurite number (D), node number (E) and neurite ends (F) in Pcdhg WT(green) and Pcdhg mutant (magenta) neurons at 6, 8, 10 and 12 DAT. Two-tailed unpaired Student’s t-test, n = 32 (WT), n = 35 (Pcdhg mutant) cells at 6 DAT, n = 27 (WT and Pcdhg mutant) cells at 8 DAT, n = 26 (WT), n = 27 (Pcdhg mutant) cells at 10 DAT, and n = 27 (WT), n = 31 (Pcdhg mutant) cells at 12 DAT; cells analyzed from two transplant cohorts. All statistical comparisons were not significant following Benjamini-Hochberg multiple comparisons correction at alpha of 0.05.
-
Figure 12—source data 1
Morphological reconstruction of transplanted MGE-derived cIN precursor cells carrying WT or mutant Pcdhg.
- https://cdn.elifesciences.org/articles/55374/elife-55374-fig12-data1-v2.xlsx
Next, we utilized co-transplantation of cINs that were either Pcdhg-deficient (Nkx2.1Cre;Ai14;Pcdhgfcon3/fcon3) or WT (Gad1-GFP) to investigate whether the loss of Pcdhg affected the integration or intrinsic physiological properties of these cells at time points around the peak of Pcdhg-mediated cell death. To test how integration was affected, we made acute cortical slices of mouse visual cortex at 8, 9, 10, 11, and 12 DAT and measured the frequency of spontaneous excitatory (glutamatergic) and inhibitory (GABAergic) synaptic events, comparing mutant and WT cINs within the same slice. There was no effect of Pcdhg loss of function on the frequency of spontaneous excitatory (glutamatergic) synaptic events or on the frequency of spontaneous inhibitory (GABAergic) synaptic events (Figure 13B and Tables 1 and 2). We next investigated whether the loss of Pcdhg function altered intrinsic physiological properties in co-transplanted cINs. There was no effect of the loss of Pcdhg on the maximum firing rate (Figure 13C and Tables 1 and 2), membrane time constant (Tau) (Figure 13D and Table 1), or input resistance (Figure 13F and Table 1). A difference in capacitance was observed between WT and Nkx2.1Cre;Ai14 ;Pcdhgfcon3/fcon3 cINs at 8DAT, but this difference was not statistically significant following multiple comparisons correction and was not seen at later time points (Figure 13E and Table 1). We conclude that the synaptic integration and morphological - functional maturation of cINs lacking Pcdhg is similar to that of WT controls.
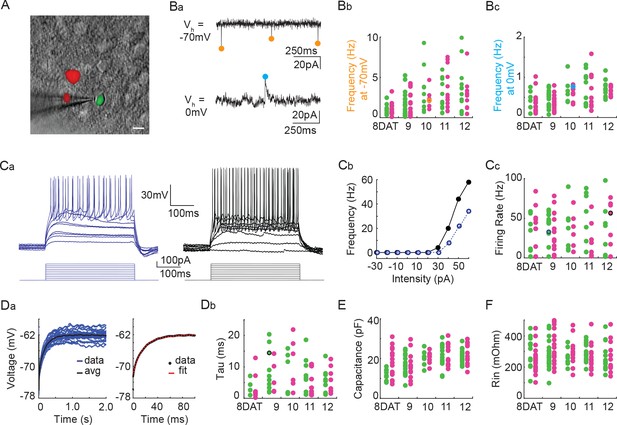
Pcdhg deletion does not affect the physiological properties of cINs during the period of programmed cell death.
(A) DIC image with fluorescence image overlaid showing co-transplanted cINs from the MGE of Gad1-GFP (Pcdhg WT, green) or Nkx2.1Cre;Ai14;Pcdhgfcon3/fcon3 (Pcdhg mutant, red) embryos, recorded in an acute brain slice taken from visual cortex (scale bar 10 µm). (Ba) Representative voltage clamp recordings (1 s) from a Nkx2.1Cre;Ai14;Pcdhgfcon3/fcon3 (Pcdhg mutant) cINs held at −70 mV (top) to record glutamatergic events (orange circles) and 0 mV (bottom) to record GABAergic events (cyan circles). Bb, Bc. Group data from cINs recorded at 8, 9, 10, 11, and 12 DAT showing that co-transplanted Gad1-GFP cINs (WT, green circles) and Nkx2.1Cre;Ai14;Pcdhgfcon3/fcon3 cINs (Pcdhg mutant, magenta circles) have similar rates of glutamatergic events (measured at −70 mV, Bb) and similar rates of GABAergic events (measured at 0 mV, Bc). The voltage clamp recordings from (Ba) are represented within the group data by the orange (−70 mV) and cyan (0 mV) circles in Bb and Bc) respectively. Ca, Cb. Representative current clamp traces showing a range of firing rates from a Gad1-GFP cIN (WT, blue trace) and a Nkx2.1Cre;Ai14;Pcdhgfcon3/fcon3 (Pcdhg mutant, black trace) cIN responding to intracellular current injections (Ca), and the corresponding FI curves (Cb). (Cc) Group data from cINs recorded at 8, 9, 10, 11, and 12 DAT showing that co-transplanted Gad1-GFP cINs (WT, green circles) and Nkx2.1Cre;Ai14;Pcdhgfcon3/fcon3 cINs (Pcdhg mutant, pink circles) have similar maximum spike rates. The current clamp traces from (Ca and Cb) are represented within the group data by the blue and black circles. (Da) Left: Gad1-GFP (WT) cIN voltage responses to repeated current injections (blue traces). Right: The membrane time constant (Tau) is calculated by fitting an exponential to the average voltage trace (black line). (Db) Group data from current clamp recordings of co-transplanted Gad1-GFP cINs (WT, green circles) and Nkx2.1Cre;Ai14;Pcdhgfcon3/fcon3 cINs (Pcdhg mutant, magenta circles) at 8, 9, 10, 11, and 12 DAT shows that Pcdhg deletion does not affect membrane time constant. The current clamp recording from (Da) is represented within the group data by a black circle. (E and F) Group data from current clamp recordings of co-transplanted Gad1-GFP cINs (WT, green circles) and Nkx2.1Cre;Ai14;Pcdhgfcon3/fcon3 cINs (Pcdhg mutant, magenta circles) at 8, 9, 10, 11, and 12 DAT shows that Pcdhg deletion does not affect either capacitance (E) or input resistance (F). Cells analyzed in A–F were taken from at least three transplant cohorts.
-
Figure 13—source data 1
Analysis of the intrinsic electrophysiological properties of transplanted MGE-derived cIN precursor cells carrying WT or mutant Pcdhg.
- https://cdn.elifesciences.org/articles/55374/elife-55374-fig13-data1-v2.xlsx
Group data for the frequency of spontaneous excitatory and inhibitory synaptic currents (Hz), max firing rate (Hz), tau (ms), capacitance (pF), and input resistance (mOhm) from Figure 13.
The mean, standard deviation, sample size, and statistical tests are reported. Comparisons were not statistically significant following Benjamini-Hochberg multiple comparisons correction at an alpha of 0.05.
Frequency of spontaneous excitatory synaptic currents (Hz) | Frequency of spontaneous inhibitory synaptic currents (Hz) | |||||
---|---|---|---|---|---|---|
Pcdhgfcon3/fcon3 | WT | Mann-Whitney | Pcdhgfcon3/fcon3 | WT | Mann-Whitney | |
Mean ± SD | Mean ± SD | p value | Mean ± SD | Mean ± SD | p value | |
8DAT | 1.0 ± 0.7 (n = 18) | 1.0 ± 0.7 (n = 9) | 0.890 | 0.4 ± 0.2 (n = 18) | 0.4 ± 0.2 (n = 6) | 0.463 |
9DAT | 1.4 ± 1.4 (n = 19) | 1.6 ± 1.4 (n = 21) | 0.432 | 0.4 ± 0.2 (n = 15) | 0.4 ± 0.2 (n = 18) | 0.347 |
10DAT | 2.2 ± 1.0 (n = 9) | 3.4 ± 2.8 (n = 9) | 0.652 | 0.6 ± 0.2 (n = 9) | 0.6 ± 0.3 (n = 7) | 0.859 |
11DAT | 3.2 ± 2.3 (n = 14) | 2.5 ± 1.8 (n = 13) | 0.298 | 0.6 ± 0.5(n = 11) | 0.7 ± 0.4 (n = 11) | 0.711 |
12DAT | 2.6 ± 2.1 (n = 11) | 3.3 ± 2.8 (n = 14) | 0.597 | 0.7 ± 0.1 (n = 10) | 0.7 ± 0.2 (n = 13) | 0.436 |
Max Firing Rate (Hz) | Tau (ms) | |||||
Pcdhgfcon3/fcon3 | WT | Mann-Whitney | Pcdhgfcon3/fcon3 | WT | Mann-Whitney | |
Mean ± SD | Mean ± SD | p value | Mean ± SD | Mean ± SD | p value | |
8DAT | 21.8 ± 23 (n = 16) | 21.5 ± 22.9 (n = 12) | 0.789 | 3.6 ± 4.3 (n = 8) | 3.8 ± 2.6 (n = 4) | 0.683 |
9DAT | 29.1 ± 25.1 (n = 17) | 35.0 ± 22.3 (n = 19) | 0.416 | 6.8 ± 5.3 (n = 5) | 8.7 ± 6.7 (n = 10) | 0.717 |
10DAT | 35.7 ± 22.8 (n = 6) | 37.7 ± 28.6 (n = 7) | 1.000 | 11.0 ± 8 (n = 6) | 7.8 ± 6.7 (n = 6) | 0.387 |
11DAT | 24.5 ± 24.4 (n = 11) | 36.3 ± 34.9 (n = 12) | 0.534 | 5.7 ± 4.3 (n = 10) | 7.3 ± 5.7 (n = 9) | 0.707 |
12DAT | 35.0 ± 32.3 (n = 10) | 29.8 ± 29.3 (n = 12) | 0.757 | 5.8 ± 4.1 (n = 8) | 5.5 ± 3.9 (n = 9) | 0.880 |
Capacitance (pF) | Input Resistance (mOhm) | |||||
Pcdhgfcon3/fcon3 | WT | Mann-Whitney | Pcdhgfcon3/fcon3 | WT | Mann-Whitney | |
Mean ± SD | Mean ± SD | p value | Mean ± SD | Mean ± SD | p value | |
8DAT | 18.3 ± 7.3 (n = 16) | 11.6 ± 2.7 (n = 10) | 0.019 | 257.3 ± 87.1 (n = 23) | 313.6 ± 86.5 (n = 16) | 0.027 |
9DAT | 16.4 ± 6.0 (n = 15) | 17.2 ± 5.1 (n = 19) | 0.465 | 327 ± 106.5 (n = 23) | 278.9 ± 83.4 (n = 23) | 0.095 |
10DAT | 20.5 ± 2.8 (n = 9) | 20.2 ± 3.9 (n = 7) | 0.837 | 297.5 ± 94.3 (n = 11) | 264.7 ± 52.9 (n = 9) | 0.304 |
11DAT | 22.4 ± 5.1 (n = 15) | 20.4 ± 6.5 (n = 13) | 0.503 | 269.1 ± 79.6 (n = 15) | 294.2 ± 59.9 (n = 14) | 0.185 |
12DAT | 23.2 ± 5.8 (n = 13) | 21.5 ± 3.4 (n = 15) | 0.338 | 266.5 ± 96.4 (n = 13) | 262.3 ± 83.6 (n = 15) | 0.882 |
Ratios are reported for the frequency of spontaneous excitatory and inhibitory synaptic currents where wild type and Pcdhgfcon3/fcon3 co-transplants are compared within the same slice.
The natural log of each ratio was averaged for 8, 9, 10, 11, and 12 DAT and show that slice variation was not significant.
Frequency of spontaneous excitatory synaptic currents | Frequency of spontaneous inhibitory synaptic currents | ||
---|---|---|---|
LN(Pcdhg fcon3/fcon3/WT) | LN(Pcdhg fcon3/fcon3/WT) | ||
Mean ± SD | Mean ± SD | ||
8DAT | −0.305 ± 0.425 | 0.162 ± 0.793 | |
9DAT | 0.177 ± 0.468 | 0.018 ± 0.550 | |
10DAT | 0.321 ± 0.706 | 0.301 ± 0.436 | |
11DAT | 0.120 ± 0.927 | −0.075 ± 0.262 | |
12DAT | −0.523 ± 0.943 | −0.072 ± 0.128 |
Discussion
The findings above indicate that Pcdhg genes play a critical role in regulating cIN survival during the endogenous period of cIN programmed cell death. Specifically, Pcdhgc3, Pcdhgc4, and Pcdhgc5 isoforms within the Pcdhg cluster are essential for the selection of those cINs that survive past the period of programmed cell death and become part of the adult cortical circuit. Pcdhg genes do not affect the production or migration of cINs and appear to be dispensable for the survival of cINs after the period of cell death. Together with previous work in the spinal cord and retina, these results suggest that Pcdhgc3, Pcdhgc4, and Pcdhgc5 isoforms are key to the regulation of programmed cell death in the CNS. In contrast, deletions of the alpha and beta Pcdh gene clusters did not alter cell death during this period.
Our initial approach involved the removal of Pcdhg function from all GAD2 expressing cells using the Gad2Cre;Ai14;Pcdhgfcon3/fcon3 mice. These mice displayed a dramatic reduction of cortical interneurons of all subtypes, including a significant decrease in the number of VIP+ cells, which are derived from the CGE. A similar observation has been recently reported by Carriere et al. (2020). In these mice, Pcdhg function was also removed from most other GABAergic neurons throughout the nervous system, as well as from a small fraction of astrocytes (Taniguchi et al., 2011). Since the removal of Pcdhg function in all GAD2-CRE expressing cells could affect the survival of cINs indirectly, we used Nkx2.1Cre;Ai14 ; mice to more specifically remove Pcdhg function from MGE derived cINs. As in the Gad2Cre;Ai14;Pcdhgfcon3/fcon3 mice, a sharp decrease in cINs was observed, but now only MGE-derived PV, SST and a subpopulation of RLN cIN were affected. The number of VIP cells was not affected in these mice, suggesting that the reduction in the number of MGE-derived cINs does not affect the survival of those derived from the CGE. Consistent with recent observations (Carriere et al., 2020), the number of un-recombined PV and SST (PV+/tdTomato- and SST+/tdTomato-) cINs in Nkx2.1Cre;Ai14;Pcdhgfcon3/fcon3 mice, increased compared to WT mice (Figure 3—figure supplement 4). These cells, which are likely derived from the dorsal NKX6.2+/NKX2.1- MGE domain (Hu et al., 2017a; Hu et al., 2017b; Fogarty et al., 2007; Sousa et al., 2009) may increase their survival in compensation for the loss of Nkx2.1-derived cINs lacking Pcdhg function. However, we cannot exclude that the increased number of un-recombined PV and SST cells in Nkx2.1Cre;Ai14;Pcdhgfcon3/fcon3 mice resulted from increased production or migration of cINs derived from regions of low, or no, expression of Nkx2.1. Further experiments will be required to understand the origin of these un-recombined PV+/Ai14- and SST+/Ai14- cINs and whether the observed increase in their numbers is due to compensatory survival mechanisms.
Pcdhg genes are not required for the normal production of young Nkx2.1-derived cINs in the MGE, or for their migration into the mouse cerebral cortex. The extent of proliferation in the MGE was essentially the same in Pcdhg WT and mutant mice, and the number of migrating MGE-derived cINs into the cortex was also indistinguishable between control and mutant mice. We did not directly address whether interference with the Pcdha and Pcdhb gene clusters affected the birth and migration of cINs, but we infer these two clusters also have no, or minimal effects on cIN production and migration because the final numbers of MGE-derived cINs were not significantly affected after the loss of either Pcdha or Pcdhb. However, the aggregate loss of Pcdh genes in multiple clusters might in principle be required for phenotypes to be manifested (Ing-Esteves et al., 2018). Our findings, therefore, cannot exclude the possibility that simultaneous elimination of the Pcdha and Pcdhb isoforms might have an effect on cIN production, migration, or apoptosis. However, the elimination of Pcdhg alone, and specifically of the Pcdhgc3, Pcdhgc4, and Pcdhgc5 isoforms increases cell death among cINs, establishing that removal of a limited number of isoforms in the Pcdhg cluster is sufficient to reveal the cell-death phenotype.
Importantly, the increase in programmed cell death observed following the loss of Pcdhg function was fully rescued when the pre-apoptotic gene Bax was also eliminated. Not only was the increased Pcdhg-dependent cell death eliminated in Bax mutant animals, but these animals had ~40% increase in the numbers of surviving cINs compared to WT controls, identical to the effect of the Bax mutation in wild type animals. This observation is consistent with previous observation showing that ~ 40% of cINs are eliminated during the period of programmed cell death (Southwell et al., 2012; Wong et al., 2018). Moreover, the increased death of cINs after removal of Pcdhg function occurs precisely during the normal period of programmed cell death. These observations indicate that Pcdhg isoforms are required specifically to regulate cIN numbers during the critical window of programmed cell death. This is consistent with previous studies in the retina and spinal cord that have pointed to Pcdhg, and specifically the C-isoforms (Pcdhgc3, Pcdhgc4 and Pcdhgc5), as key mediators of programmed cell death (Lefebvre et al., 2008; Chen et al., 2012). Interestingly, in all three neural structures, the cortex, the spinal cord, and the retina, Pcdhg C-isoforms appear to be the key regulators of survival of local circuit interneurons.
A recent study suggests that the Pcdhgc4 isoform is the key mediator in the regulation of neuronal cell death in the spinal cord (Garrett et al., 2019). How the specific Pcdhgc4 isoform in the Pcdhg gene cluster mediates cell death remains a fundamental question for future research. Interestingly, the Pcdhgc4 isoform appears to be unique in that it is the only Pcdh isoform that does not bind in a homophilic manner (Garrett et al., 2019) and it is not translocated to the membrane unless it is associated with other Pcdha or Pcdhg (Aye et al., 2014). The mechanism by which the Pcdhgc4 isoform regulates cell-cell interactions among young cINs, leading to the adjustment of local circuit neuron numbers, remains unclear.
Heterochronic transplantation of cIN from the MGE into the cortex of WT mice allowed us to test for the survival of Pcdhg WT and Pcdhg mutant cIN simultaneously and in the same environment. As previously reported (Southwell et al., 2012), cINs die following their own time-course of maturation. Consistent with this, the transplanted cells (extracted from the MGE at E13.5) died with a delay of 6–12 days compared to the endogenous host cINs when transplanted into P0-P6 WT mice. This is consistent with the notion that the cellular age of cIN determines the timing of programmed cell death (Southwell et al., 2012). Interestingly, the role of Pcdhg function was clearly evident in these co-transplants in that the survival of the mutant cells was extremely low compared to that of WT cells. The dramatic decrease in the survival of Pcdhg mutant cINs occurs precisely during the period of programmed cell death for the transplanted population. The survival of transplanted WT cINs as well as that of Pcdhg mutant cINs was constant over a wide range of densities, as evidenced by the fact that while the density of transplanted cINs decreases as a function of the distance from the transplantation site, the proportion of dying cells of both phenotypes was similar at different distances from the site of transplantation.
Interestingly, the survival of WT cIN may also be reduced when co-transplanted with Pcdhg deficient MGE-cells although this difference did not reach statistical significance with the numbers of cases studied. If true, these findings would be consistent with the notion that cell-cell interactions among young cIN after their migration is an essential step in determining their final numbers. However, we cannot exclude that the increase in the elimination of WT cells may result from a non-specific (e.g., toxic) effect of the increased cell death among Pcdhg mutant cells. If the latter occurs, the process is specific to the population of Nkx2.1+ MGE-derived cINs because there was no effect on cell death of WT CGE-derived VIP cINs (Figure 3E, Figure 3—figure supplement 1) or on non-Nkx2.1-derived SST or PV cells (Figure 3—figure supplement 4). Interactions mediated by Pcdhg, and specifically among the C-isoforms, may directly or indirectly regulate survival of cINs of the same age and origin.
Unlike the spinal cord where cell death takes place prenatally (Wang et al., 2002b; Prasad et al., 2008), cIN programmed cell death occurs mostly postnatally. Since mice lacking Pcdhgc3, Pcdhgc4, and Pcdhgc5 isoforms die soon after birth, we could not study normal cIN cell death directly in these mutant animals. We, therefore, took advantage of transplantation and co-transplantation to compare the survival of cells lacking these three isoforms. The loss of cINs lacking Pcdhgc3, Pcdhgc4, and Pcdhgc5 isoforms was identical to that when the function of the entire Pcdhg cluster is lost. This further suggests that these Pcdhg C-isoforms (Pcdhgc3, Pcdhgc4, and Pcdhgc5) are the key to the regulation of cIN death. The co-transplantation assay, implemented in the present study, provides strong evidence that Pcdhg in cINs are key to their selection by programmed cell death. Pcdhg could be mediating initial cell-cell interactions that are important for the survival of cINs. Two non-exclusive possibilities exist: (1) Pcdhg mediate cell-cell interaction among young cINs to adjust their population size, and levels of inhibition, according to the numbers that reached the cortex; (2) Pcdhg mediate interactions with locally produced excitatory pyramidal neurons to adjust final numbers according to local levels of excitation. For the latter, MGE-derived cIN could interact with pyramidal neurons via Pcdhg C-isoforms. However, alternative # 2 is unlikely to explain how Pcdhg adjust cIN numbers since using conditional removal of Pcdhg in pyramidal cells shows no effect on the survival of cINs (Carriere et al., 2020). However, we cannot exclude that initial connectivity with excitatory pyramidal neurons may indeed require the proper expression of Pcdhg among cINs through non-homophilic interactions.
A recent study has shown that coordinated activity of synaptically connected assemblies of cINs is essential for their survival (Duan et al., 2020). Pyramidal cells receive information from these assemblies via GABAAγ2-signaling and through the de-synchronization of their activity regulate cIN programmed cell death. Pcdhg could be important in bringing together cINs of a common origin and at similar stages of maturation for the formation of initial cIN functional assemblies. The formation of these assemblies of synchronously firing cINs and the subsequent selection by pyramidal driven de-synchronization could explain both cell/population autonomous (Southwell et al., 2012), and non-cell autonomous (Wong et al., 2018) mechanisms of cIN programmed cell death. Interestingly, PCDHGC5 binds to the GABAAγ subunit of the GABA receptor (Li et al., 2012), but the role of PCDHGC5-GABAAγ interaction on neuronal survival remains unknown. The transplantation assay provides a powerful tool to further study how Pcdhg, cell-cell interactions, and cellular age contribute to cIN selection. It will be interesting, for example, to determine if heterochronically transplanted cINs form functional assemblies and whether these assemblies are affected by the removal of different Pcdhg isoforms.
During the evolution of multiple mammalian species including that of humans, the cerebral cortex has greatly expanded in size and in the number of excitatory and inhibitory neurons it contains. Interestingly, the proportion of cINs to excitatory pyramidal neurons has remained relatively constant. Appropriate numbers of inhibitory cINs are considered essential in the modulation of cortical function. The embryonic origin of cINs, far from the cerebral cortex, raises basic questions about how their numbers are ultimately controlled in development and during evolution. Coordinated increased production of inhibitory interneurons in the MGE and CGE is an essential step to satisfy the demand of an expanded cortex (Hansen et al., 2013). In addition, MGE and CGE derived interneurons in larger brains require longer and more protracted migratory periods (Paredes et al., 2016). Interneurons arrive in excess of their final number. This is ultimately adjusted by a period of programmed cell death once the young cINs have arrived in the cortex. Here we have identified the C-isoforms (Pcdhgc3, Pcdhgc4 and Pcdhgc5) in the Pcdhg cluster as an essential molecular component that regulates programmed cell death among cINs. The fact that a cell surface adhesion protein plays a key role in this regulation suggests that interactions with other cells, possibly other cINs of the same age (Southwell et al., 2012), or possibly excitatory pyramidal cells (Wong et al., 2018), is part of the logic to adjust the final number of these essential GABAergic cells for proper brain function. An understanding of the cell-cell interactions that use Pcdhg C-isoform to regulate cIN cell death should give fundamental insights into how the cerebral cortex forms and evolves.
Materials and methods
Reagent type (species) or resource | Designation | Source or reference | Identifiers | Additional information |
---|---|---|---|---|
Genetic reagent (mouse) | Gad2Cre | PMID:21943598 | Also referred to as Gad2-IRES-Cre knock-in | |
Genetic reagent (mouse) | Nkx2.1Cre | PMID:17990269 | Also referred to as C57BL/6J-Tg(Nkx2-1-cre)2Sand/J | |
Genetic reagent (mouse) | PVCre | PMID:15836427 | Also referred to as B6;129P2-Pvalbtm1(cre)Arbr/J | |
Genetic reagent (mouse) | SstCre | PMID:21943598 | Also referred to as Ssttm2.1(cre)Zjh/J | |
Genetic reagent (mouse) | Ai14 | The Jackson Laboratory | Also referred to as Ai14 , Ai14 D or Ai14 (RCL-tdT)-D | |
Genetic reagent (mouse) | Gad1-GFP | The Jackson Laboratory | Also referred to as G42 line. | |
Genetic reagent (mouse) | Bax-/- | The Jackson Laboratory | Also referred to as B6;129-Baxtm2Sjk Bak1tm1Thsn/J | |
Genetic reagent (mouse) | Pcdhaacon/acon | PMID:28450636 | Referred to as Pcdhαf/f and Pcdhα-/- in original publication. | |
Genetic reagent (mouse) | Pcdhbdel/del | PMID:28450637 | Referred to as Pcdhß-/- in original publication. | |
Genetic reagent (mouse) | Pcdhgtako/tako; Pcdhga1, Pcdhga2, and Pcdhga3 mutant; Pcdhga1, Pcdhga2, and Pcdhga3 KO | PMID:22884324 | Referred to as Pcdhgtako/tako in original publication. | |
Genetic reagent (mouse) | Pcdhgtcko/tcko; Pcdhgc3, Pcdhgc4, and Pcdhgc5 mutant; Pcdhgc3, Pcdhgc4, and Pcdhgc5 KO | PMID:22884324 | Referred to as Pcdhgtcko/tcko in original publication. | |
Genetic reagent (mouse) | Pcdhgfcon3/fcon3 | PMID:19029044 | Referred to as Pcdh-γfcon3 in original publication. | |
Antibody | anti-GFP (chicken polyclonal) | Aves Lab | Cat# GFP-1020, RRID:AB_10000240 | IF(1:2500) |
Antibody | Anti-Reelin (mouse monoclonal) | MBL International | Cat#: MBL, D223–3, RRID:AB_843523 | IF(1:500) |
Antibody | Anti-PV (rabbit antiserum) | Swant | Cat#: PV27 , RRID:AB_2631173 | IF(1:1000) |
Antibody | Anti-PV (mouse monoclonal) | Sigma-Aldrich | Cat#: P3088, RRID:AB_477329 | IF(1:500) |
Antibody | Anti-SST (rat, polyclonal | Santa Cruz Biotechnology | Cat#:sc-7819, RRID:AB_2302603 | IF(1:500) |
Antibody | Anti-cleaved caspase 3 (rabbit polyclonal) | Cell Signaling Technology | Cat#: 9661L, RRID:AB_2341188 | IF(1:400) |
Antibody | Anti-phosphohiston-H3 (rabbit polyclonal) | EDM Millipore | Cat#: 06–570, RRID:AB_310177 | IF(1:500) |
Antibody | Anti-NKX2-1 (rabbit polyclonal) | Life Technologies | Cat#: sc-13040, ARRID:AB_793532 | IF(1:250) |
Chemical compound, drug | DNAse I | Sigma Millipore | Cat#: 260913-10MU | 180 ug/mL |
Commercial assay or kit | QuantiTect Rev. Transcription Kit | Qiagen | Cat#: 205311 | |
Software, algorithm | Stereo Investigator | MBF bioscience | ||
Software, algorithm | Neurolucida | MBF bioscience | ||
Software, algorithm | custom software written in MATLAB | ‘other’ | Larimer, 2020. mPhys. MATLAB Central File Exchange. (https://www.mathworks.com/matlabcentral/fileexchange/21903-mphys). 1.2.0.0. |
Animals
R26-Ai14 , Gad1-GFP, Gad2-ires-Cre (Gad2Cre), BAC-Nkx2.1-Cre (Nkx2.1Cre), Sst-ires-Cre (SstCre), PV-IRES-Cre-pA (PVCre), Bax-/-, Baxfl/fl and WT C57BL/6J breeders were purchased from the Jackson Laboratory. Whenever possible, the number of males and females was matched for each experimental condition. All protocols and procedures followed the University of California, San Francisco (UCSF) guidelines and were approved by the UCSF Institutional Animal Care Committee.
Pcdhg loss of function mice were obtained by crossing Pcdhgfcon3/fcon3 mice with Gad2Cre;Ai14;Pcdhgfcon3/+ mice, Nkx2.1Cre;Ai14;Pcdhgfcon3/+, PVCre;Ai14;Pcdhgfcon3/+ or SstCre;Ai14;Pcdhgfcon3/+. Pcdhga1, Pcdhga2 and Pcdhga3 isoform knockout mice were obtained from crosses of Pcdhgtako/tako mice to Nkx2.1Cre;Ai14;Pcdhgtako/+ mice.
Pcdha loss function mice were obtained by crossing Pcdhaacon/acon mice with Nkx2.1Cre;Ai14; Pcdhaacon/+ mice. Pcdhb loss function mice were obtained by crossing Pcdhbdel/del mice with Nkx2.1Cre;Ai14;Pcdhbdel/+ mice. Embryonic donor tissue was produced by crossing WT C57BL/6J to heterozygous mice expressing green fluorescent protein-expressing (GFP) driven by Gad1 promoter. Pcdhgfcon3/fcon3 (tdTomato-expressing) tissue was obtained from embryos produced by crossing Nkx2.1Cre;Ai14;Pcdhgfcon3/+ mice with Pcdhgfcon3/fcon3 mice. Pcdhgtako/tako (tdTomato-expressing) tissue was obtained from embryos produced by crossing Nkx2.1Cre;Ai14;Pcdhgtako/+ mice to homozygote Pcdhgtako/tako mice. Pcdhgtcko/tcko (tdTomato-expressing) tissue was obtained from embryos produced by crossing Nkx2.1Cre;Ai14;Pcdhgtcko/+ mice to heterozygote Pcdhgtcko/+ mice. Homozygous Pcdhgtcko/tcko mice die around birth. GAD1-GFP and Nkx2.1Cre; Ai14 offspring were genotyped under an epifluorescence microscope (Leica), and PCR genotyping was used to screen for Pcdhgfcon3/fcon3, Pcdhgtako/tako and Pcdhgtcko/tcko embryos in GFP or Ai14 positive animals. All cell transplantation experiments were performed using wild type C57Bl/6 recipient mice. All mice were housed under identical conditions.
Immunostaining
Request a detailed protocolMice were fixed by transcardiac perfusion with 10 mL of ice-cold PBS followed by 10 mL of 4% formaldehyde/PBS solution. Brains were incubated overnight (12–24 hr) for postfixation at 4°C, then rinsed with PBS and cryoprotected in 30% sucrose/PBS solution for 48 hr at 4°C. Unless otherwise stated, immunohistochemistry was performed on 50 μm floating sections in Tris Buffered Saline (TBS) solution containing 10% normal donkey serum, 0.5% Triton X-100 for all procedures on postnatal mice. Immunohistochemistry from embryonic tissue was performed on 20 μm cryostat sections. All washing steps were done in 0.1% Triton X-100 TBS for all procedures. Sections were incubated overnight at 4°C with selected antibodies, followed by incubation at 4°C overnight in donkey secondary antibodies (Jackson ImmunoResearch Laboratories). For cell counting and post hoc examination of marker expression, sections were stained using chicken anti-GFP (1:2500, Aves Labs, GFP-1020, RRID:AB_10000240), mouse anti-Reelin (1:500 MBL, D223–3, RRID:AB_843523), rabbit anti-PV (1:1000, Swant PV27 , RRID:AB_2631173), mouse anti-parvalbumin (anti-PV, 1:500, Sigma-Aldrich, P3088, RRID:AB_477329), rat anti-somatostatin (SST, 1:500, Santa Cruz Biotechnology, sc-7819, RRID:AB_2302603), anti-cleaved caspase 3 (1:400, Cell Signaling Technology, 9661L, RRID:AB_2341188), rabbit anti-phosphohistone-H3 (1:500; EDM Millipore, RRID:AB_310177), rabbit anti-NKX2-1 (1:250, Life Technologies, RRID:AB_793532).
Cell counting
Request a detailed protocolFor cell density counts in the visual and barrel cortex (Figure 2C & C’, Figure 3C & C’, Figure 5C, Figure 6 and Figure 7), cells were directly counted using a Zeiss Axiover-200 inverted microscope (Zeiss) and an AxioCam MRm camera (Zeiss), using Stereo Investigator (MBF). tdTomato+ cells were counted in every six sections (i.e., 300 µm apart) along the rostral-caudal axis of visual and barrel cortex. Total cell counts were extrapolated by Stereo Investigator. Cell densities were determined by dividing the total number of tdTomato+ cells by the volume of the region of interest in the visual or barrel cortex, identified by landmarks, for each animal. To measure PV, SST, RLN and VIP-positive cell densities in the visual cortex (Figure 2D and Figure 3E), cells were counted from confocal-acquired images. Cleaved caspase-3-positive (CC3+) cells were counted from images acquired on a Zeiss Axiover-200 inverted microscope (Zeiss) and an AxioCam MRm camera (Zeiss) using Neurolucida (MBF). Cleaved caspase-3-positive cells were counted in the cortex of every six sections along the rostral-caudal axis for each animal (Figure A and B). Cells in the olfactory bulb, hippocampus, and piriform cortex were not counted. For layer distribution analysis of tdTomato+ cells and for analysis of tdTomato negative (non-recombined) PV and SST positive cells (Figure 2—figure supplement 2, Figure 3—figure supplements 3 and 4, cells were counted from confocal-acquired images and using Fiji software cell counter function.
Cell dissection and transplantation
Request a detailed protocolUnless otherwise mentioned, MGEs were dissected from E13.5 embryos as previously described (Southwell et al., 2012). The day when the sperm plug was observed was considered E0.5. Dissections were performed in ice-cold Leibovitz L-15 medium. MGEs were kept in L-15 medium at 4°C. MGEs were mechanically dissociated into a single cell suspension by repeated pipetting in L-15 medium containing DNAse I (180 ug/ml). The dissociated cells were then concentrated by centrifugation (4 min, 800xg). For all co-transplantations, the number of cells in each suspension (GFP+ or tdTomato+) was determined using a hemocytometer. Concentrated cell suspensions were loaded into beveled glass micropipettes (≈70–90 μm diameter, Wiretrol 5 μl, Drummond Scientific Company) prefilled with mineral oil and mounted on a microinjector. Recipient mice (C57Bl/6) were anesthetized by hypothermia (~4 min) and positioned in a clay head mold that stabilizes the skull (Merkle et al., 2007). Micropipettes were positioned at an angle of 0 degrees from vertical in a stereotactic injection apparatus. Unless otherwise stated, injections were performed in the left hemisphere 1 mm lateral and 1.5 mm anterior from Lambda, and at a depth of 0.8 mm from the surface of the skin. After the injections were completed, transplant recipients were placed on a warm surface to recover from hypothermia. The mice were then returned to their mothers until they were perfused or weaned (P21). Transplantation of Nkx2.1Cre;Ai14;Pcdhgtako/tako was performed using frozen cells (Figure 11B). For these experiments, dissected MGEs from each embryo were collected in 500 uL L15 and kept on ice until cryopreserved. MGEs were resuspended in 10% DMSO in L15 and cryopreserved as previously described (Rodríguez-Martínez et al., 2017). Vials were cooled to −80°C at a rate of −1 °C/minute in a Nalgene Mr. Frosty Freezing Container and then transferred to liquid nitrogen for long term storage. Prior to transplantation, vials were removed from −80°C and thawed at 37°C for 5 min, after which the freezing medium was removed from the vial and replaced with 37 °C L-15. Dissociation was performed as above.
For cell counts from transplanted animals, Gad1-GFP positive cells and tdTomato-positive cells were counted in all layers of the neocortex. Cells that did not display neuronal morphology were excluded from all quantifications. The vast majority of cells transplanted from the E13.5 MGE exhibited neuronal morphologies in the recipient brain. GFP and tdTomato-positive cells were counted from tiles acquired on a Zeiss Axiover-200 inverted microscope (Zeiss) with an AxioCam MRm camera (Zeiss); using Neurolucida (MBF). For quantification of the absolute numbers of transplanted cells in the neocortex of host recipients, cells from every second coronal section were counted (Figure 9). The raw cell counts were then multiplied by the inverse of the section sampling frequency (2) to obtain an estimate of total cell number (Figure 10). In some experiments the absolute number of grafted cells varies between transplants; hence we report findings as the fraction of cells (GFP or tdTomato) that survive from the total transplant-derived cell number in that animal (GFP + tdTomato-positive) (Figure 8). We determined for these experiments the number of transplant-derived cells of the different genotypes before and, in different animals, after the period of cell death. For some experiments, we also quantified the number of transplant-derived cells during the period of cell death (Figure 12B). In data presented as the fraction of transplant-derived cells (Figure 8 and Figure 11), GFP positive and tdTomato-positive cells were counted from coronal sections along the rostral-caudal axis in at least 10 sections per animal. The number of GFP or tdTomato-positive cells was divided by the total cell number (GFP + tdTomato) in that section. This fraction does not reflect the absolute number of cells, but their relative contribution to the overall population of transplant-derived cells at different DAT. For one experiment (Figure 10), we calculated the absolute number of transplant-derived WT (GFP) and Pcdhgfcon3/fcon3 (tdTomato) cells. For this experiment, 50 K cells of each genotype were counted before transplantation.
Neuron Morphology analysis
Request a detailed protocolRecipient brains were co-transplanted with Gad1-GFP (WT Pcdhg) and Nkx2.1Cre;Ai14;Pcdhgfcon3/fcon3 (mutant Pcdhg) MGE cIN precursors. Transplanted cells were identified in sections (50 µm) stained for GFP and tdTomato and analyzed at 6, 8, 10 and 12 days after transplantation (DAT). Neuron morphology was reconstructed from confocal image (20X objective-4X zoom) stacks taken at 1 μm intervals, using Neurolucida software (MBF). Sections with a relatively low density of GFP+ (WT) and TdTomato+ (Mutant) transplant-derived neurons (in order to clearly image individual cells) were selected and (145.31 um2) fields tiled to cover all the visible processes. All GFP+ (WT) and TdTomato+ (Mutant) positive neurons in these tiles were reconstructed. Neuron morphometric analysis was done using Neurolucida Explorer.
RT-PCR
Request a detailed protocolTotal RNA was prepared from dissected cortex of using Trizol (Invitrogen) and reverse-transcribed by Quantiscript Reverse Transcriptase (Qiagen), using a mix of oligo-dT and random primers, according to the manufacturer's protocol. Primer sequences for gene expression analysis in Figure 1 are provided in Appendix 1—table 1.
Slice electrophysiology
Request a detailed protocolAs in Larimer et al., 2016; Larimer et al., 2017; Priya et al., 2019, host animals were anesthetized and decapitated at 8, 9, 10, 11, or 12 days after transplant. The brain was removed into ice-cold dissection buffer containing (in mM): 234 sucrose, 2.5 KCl, 10 MgSO4, 1.25 NaH2PO4, 24 NaHCO3, 11 dextrose, 0.5 CaCl2, bubbled with 95% O2/5% CO2 to a pH of 7.4. Coronal slices of visual cortex (200 µm thick) were cut via vibratome (Precisionary Instruments) and transferred to artificial cerebrospinal fluid (ACSF) containing (in mM): 124 NaCl, 3 KCl, 2 MgSO4, 1.23 NaH2PO4, 26 NaHCO3, 10 dextrose, 2 CaCl2 (bubbled with 95% O2/5% CO2), incubated at 33°C for 30 min, then stored at room temperature. Fluorescently identified transplant-derived MGE-lineage interneurons (tdTomato+; Pcdhgfcon3/fcon3 or GFP; WT) were viewed using IR-DIC video microscopy. Whole-cell current-clamp recordings were made with a Multiclamp 700B (Molecular Devices) using an internal solution that contained (in mM): 140 K-gluconate, 2 MgCl2, 10 HEPES, 0.2 EGTA, 4 MgATP, 0.3 NaGTP, 10 phosphocreatine (pH 7.3, 290 mosm). Data were low-pass filtered at 2.6 kHz and digitized at 10 kHz by a 16 bit analog-to-digital converter (National Instruments). Data acquisition and analysis were done with custom software written in Matlab (https://www.mathworks.com/matlabcentral/fileexchange/21903-mphys). A Mann-Whitney (nonparametric) test, followed by multiple comparisons correction using the Benjamini-Hochberg stepdown method for control of false discovery rate (0.05 familywise) was used for the determination of statistical significance for all comparisons of physiological properties.
Statistical Analysis
Request a detailed protocolThe person carrying quantifications was blinded to the genotype, except for data shown in Figure 2—figure supplement 2, and Figure 3—figure supplements 3 and 4. With the exception of slice electrophysiology data, all results were plotted and tested for statistical significance using Prism 8. All samples were tested for normality using the Shapiro-Wilk normality test. Unpaired comparisons were analyzed using the two-tailed unpaired Student’s t test for normally distributed, and Mann-Whitney test for not normally distributed samples. For multiple comparisons analysis of one variable, either a one-way ANOVA with post hoc Tukey’s test was used to compare the mean of each column with the mean of every other column, or a Dunnett test was used to compare the mean of each column to the mean of the control group for normally distributed samples. For samples with non-Gaussian distributions, a nonparametric Kruskal-Wallis test was performed followed by a post-hoc Dunn’s test. Two-way ANOVA with post hoc Sidak’s test was used for multiple comparisons with more than one variable. Outliers were identified using ROUT method with alpha set to 0.05.
Appendix
Clustered protocadherin primer sequences for RT-PCR gene expression analysis in Figure 1.
Gene name | Oligo name | Sequence | forward/reverse |
---|---|---|---|
Pcdhga1 | Pcdhga1 | CACGAGAGCTGTGAGAAACAGG | F |
Pcdhga2 | Pcdhga2 | CTGATTTCCTCTCAGCACCTCAG | F |
Pcdhga3 | Pcdhga3 | GAAACGAAAGAAGACCCCACGC | F |
Pcdhga4 | Pcdhga4 | CTCCTGGTATCTCAAGACTTGC | F |
Pcdhga5 | Pcdhga5 | CACACAAAGAAGAGCCCGGAGA | F |
Pcdhga6 | Pcdhga6 | GCAAAGAGGAAGACTCTCTTGA | F |
Pcdhga7 | Pcdhga7 | TCAAGAATGTAAGGGTGAAGCC | F |
Pcdhga8 | Pcdhga8 | CATCCATAGATTTCCATGAGAATAA | F |
Pcdhga9 | Pcdhga9 | TCAGTTGAGCCCAAGTTTCCT | F |
Pcdhga10 | Pcdhga10 | CCAAGTGTCCTGTAGAAGACGC | F |
Pcdhga11 | Pcdhga11 | GCGAGCCTCTCCTGATAACTG | F |
Pcdhga12 | Pcdhga12 | CTTTTACCATCGGGTGATTCGG | F |
Pcdhgb1 | Pcdhgb1 | CAGGATCTCCTGTGCGATGATC | F |
Pcdhgb2 | Pcdhgb2 | GACTCTTGGGTACCAGGTACTC | F |
Pcdhgb4 | Pcdhgb4 | TGATCAGTTGAAATCAGGACAAGA | F |
Pcdhgb5 | Pcdhgb5 | CCTTCTTTGCCCTGAGTCATC | F |
Pcdhgb6 | Pcdhgb6 | CTTAATTCCGCTTCACCTTGG | F |
Pcdhgb7 | Pcdhgb7 | AAAGATAGCTCCTCGGCACTG | F |
Pcdhgb8 | Pcdhgb8 | CGAGACCTTTGTACGGAAGC | F |
Pcdhgc3 | Pcdhgc3 | GCTGCGAAGTTGTGATCCTGTG | F |
Pcdhgc4 | Pcdhgc4 | CAAGCTGTCCACCCTCTGATCTT | F |
Pcdhgc5 | Pcdhgc5 | GCCTTGCGTTCCCGCTCTAGTA | F |
PcdhgCon | PcdhgCon | GTAAACTGGGGTCCGTATCGAG | R |
Pcdhga1 | PcdhgA_1 | TTTTGTCAGCACCCCAGTC | F |
Pcdhga2 | PcdhgA_2 | TTTCCTCTCAGCACCTCAGTC | F |
Pcdhga3 | PcdhgA_3 | GTGGGAAAAGCGAGCCTCTTA | F |
Pcdhga4 | PcdhgA_4 | AGCTGTGGGAAGAGTGATCC | F |
Pcdhga5 | PcdhgA_5 | AGAGCTGTGAGAAGAGTGAGC | F |
Pcdhga6 | Pcdh_γA6 | CATCAGTCAGGAGGGCTGTG | F |
Pcdhga7 | PcdhgA_7 | ATCAGCCAAGATAGCTGTGAG | F |
Pcdhga8 | 2 PcdhgA_8 | CATCCATAGATTTCCATGAG | F |
Pcdhga9 | 2Pcdh_γA9 | TGTGGGAAGAGTGAACCTCTG | F |
Pcdhga11 | 3Pcdh_γA11 | GAAAAGCGAGCCTCTCCTG | F |
Pcdhgb1 | PcdhgB_1 | TGTGCGATGATCCTTCTGTG | F |
Pcdhgb2 | 3Pcdh_γB2 | GACTCCGGAAGTTGCTCCTC | F |
Pcdhgb4 | 2Pcdh_γB4 | CAGGACAAGATCTACAATTTGC | F |
Pcdhgb5 | 4- PcdhgB5 | TCTGGACAAGGCCTTCTTTG | F |
Pcdhgb6 | Pcdh_γB6 | GATCGTTTCCGGTAGTTCTCC | F |
Pcdhgb7 | 2- PcdhgB7 | TCCAGCCGCACAAGATATTC | F |
Pcdhgb8 | 2- PcdhgB8 | CGAGACCTTTGTACGGAAGC | F |
Pcdhgc3 | PcdhgC_3 | TGCGAAGTTGTGATCCTGTG | F |
Pcdhgc4 | PcdhgC_4 | CAAGCTGTCCACCCTCTGATC | F |
PcdhgCon | Pcdhg_COM-R | GAGAGAAACGCCAGTCAGTG | R |
Pcdha1 | 2-PcdhAlpha_1 | AAAGAAGTGACCACGCAGAAG | F |
Pcdha2 | PcdhA2-F | GGAATCAGCAGAAGAGAGACAA | F |
Pcdha3 | PcdhAlpha_3 | ACACCATGCCCAGTTAATCAAG | F |
Pcdha4 | PcdhAlpha_4 | TCTGATTCAAGGGACAGAGAGG | F |
Pcdha5 | Pcdh_α5 | TTCAGTCCCAGCCTACCTCA | F |
Pcdha6 | Pcdh_α6 | TGAGCATCAGGATTTGAACG | F |
Pcdha7 | Pcdh_α7 | GGGTCCCAGCTCTACAGATAAC | F |
Pcdha8 | Pcdh_α8 | TTCTTTGGACTCCTCCGAGA | F |
Pcdha9 | PcdhAlpha_9 | CCGAAGTGGGAATGGAAAGT | F |
Pcdha10 | PcdhAlpha_10 | CAGTGTTCCTCCTGGTTTGG | F |
Pcdha11 | Pcdh_α11 | TCCCAACCTGGGTAGAGATG | F |
Pcdha12 | PcdhAlpha_12 | TGCAGAGGACACATGTCAGAG | F |
Pcdhac1 | PcdhAlpha_C1 | TGCCAGTATCCTGTGTTCAGA | F |
Pcdhac2 | Pcdh_αc2 | AACTCACCGGCCAAAGTAGG | F |
PcdhaCon | Pcdh_α-CR | TGCTCTTAGCGAGGCAGAGTAG | R |
Pcdhb1 | pcdhb1_F | TAGTTGCCGAGGGTAACAGG | F |
Pcdhb1 | Pcdh_ß1_R | GGGTCAGATTTGCCACAAAG | R |
Pcdhb2 | 2Pcdh_ß2_F | AATGGTTTGCTCCATCCAAG | F |
Pcdhb2 | 2Pcdh_ß2_R | GATCCAGGGCTGTGTTTGTC | R |
Pcdhb3 | PcdhB3-F | TCAGGGAAATGCACAGTCATAG | F |
Pcdhb3 | PcdhB3-R | CACAGTTTCTGCTGAATTCTCG | R |
Pcdhb4 | pcdhb4_F | TCTGGGATGACCACAGTTCA | F |
Pcdhb4 | pcdhb4_R | TGCACCTCATAGAGCGATTG | R |
Pcdhb5 | 3Pcdh_ß5_F | TGTGCTCACGCTCTACCTTG | F |
Pcdhb5 | 3Pcdh_ß5_R | CTCACTCCCACGAACATCAG | R |
Pcdhb6 | PcdhB6-F | GTCAAGGACAATGGAGAACCTC | F |
Pcdhb6 | PcdhB6-R | CTTCATCCTGTGAAGAGTCGTG | R |
Pcdhb7 | pcdhb7_F | GACCTCATGGAGAAGCTGGA | F |
Pcdhb7 | 2-pcdhb7_R | GAGTTGTTGGCTCACTGCAA | R |
Pcdhb8 | 2-pcdhb8_F | ATTCCATGCCAGAAGAAACG | F |
Pcdhb8 | 2-pcdhb8_R | TAGTGGTCAGTTCCCCAACC | R |
Pcdhb9 | 2-pcdhb9_F | AACAAAGGAAGCAGACAAGAGC | F |
Pcdhb9 | 2-pcdhb9_R | TCACCGTGTTGCTCATAATCTC | R |
Pcdhb10 | 2PcdhB10-F | GGTATTTGAGCGTGATCTAGGG | F |
Pcdhb10 | 2PcdhB10-R | AGAGGGCGCTTCTTCTTCTAGT | R |
Pcdhb11 | 3Pcdh_beta11_F | CGACCACTCTCCAGAGTTCC | F |
Pcdhb11 | 3Pcdh_beta11_R | GCTGCCTTCAGAGGAAACAC | R |
Pcdhb12 | pcdhb12_F | CTGGGATATATGGCAATGTCG | F |
Pcdhb12 | pcdhb12_R | GTCAGACGGATTTCTCCTGTG | R |
Pcdhb13 | 2PcdhB13-F | GATAACGCTCCAGAAGTGATCC | F |
Pcdhb13 | 2PcdhB13-R | CTGCTGGTTATTTCCAGAGTCC | R |
Pcdhb14 | 2-pcdhb14_F | CCCAGCACACCATAACAGTG | F |
Pcdhb14 | 2-pcdhb14_R | GATGGTGCCTATGTGCAATG | R |
Pcdhb15 | PcdhB15-F | CTCAGTCCGCTTACTGGAGAAT | F |
Pcdhb15 | PcdhB15-R | AATGGTCTTTCCAACAGCAACT | R |
Pcdhb16 | pcdhb16-F | TCATCCGTGAGAACAACAGC | F |
Pcdhb16 | pcdhb16-R | GCAGCAGCGAGTAAGTGATG | R |
Pcdhb17 | PcdhB17-F | AAGAGAGCACTTGACAGGGAAG | F |
Pcdhb17 | PcdhB17-R | AGACCTGCACTGTTATGGTGTG | R |
Pcdhb18 | PcdhB18-F | TGCATGGAGTCATACTTTGGAC | F |
Pcdhb18 | pcdhb18-R | TAGCCATGTTTAGAAAGCAGCA | R |
Pcdhb19 | 3Pcdh_beta19_F | TTCGCTCTTCCTCCTCTCTG | F |
Pcdhb19 | 3Pcdh_beta19_R | AGTTCCCGCACTGTTAATGC | R |
Pcdhb20 | PcdhB20-F | GAAGTGATCATGTCGTCGGTTA | F |
Pcdhb20 | PcdhB20-R | CTTCCGTTGTCTCCAGAGTCTT | R |
Pcdhb21 | 2Pcdh_beta21_F | ACAGCACTCGGGCTTTCTAC | F |
Pcdhb21 | 2Pcdh_beta21_R | GGCAGCTCAGAGAGTGGTTC | R |
Pcdhb22 | 2Pcdh_beta22_F | GCTCTGCTAGCGTCACACTG | F |
Pcdhb22 | 2Pcdh_beta22_R | ATCACCTTCCTGGTGACTGG | R |
Data availability
Data generated for this study are included in the manuscript and source data files have been provided for Figures 1 to 13.
References
-
GABAergic signaling as therapeutic target for autism spectrum disordersFrontiers in Pediatrics 2:70.https://doi.org/10.3389/fped.2014.00070
-
Homeostatic regulation of interneuron apoptosis during cortical developmentJournal of Experimental Neuroscience 12:117906951878427.https://doi.org/10.1177/1179069518784277
-
GABA(A) receptor downregulation in brains of subjects with autismJournal of Autism and Developmental Disorders 39:223–230.https://doi.org/10.1007/s10803-008-0646-7
-
Differential expression of individual gamma-protocadherins during mouse brain developmentMolecular and Cellular Neuroscience 29:603–616.https://doi.org/10.1016/j.mcn.2005.05.001
-
Non-epithelial stem cells and cortical interneuron production in the human ganglionic eminencesNature Neuroscience 16:1576–1587.https://doi.org/10.1038/nn.3541
-
Distinct and cooperative functions for the Protocadherin-α, -β and -γ clusters in neuronal survival and axon targetingFrontiers in Molecular Neuroscience 9:00155.https://doi.org/10.3389/fnmol.2016.00155
-
Gene expression deficits in a subclass of GABA neurons in the prefrontal cortex of subjects with schizophreniaThe Journal of Neuroscience 23:6315–6326.https://doi.org/10.1523/JNEUROSCI.23-15-06315.2003
-
Functions and dysfunctions of neocortical inhibitory neuron subtypesNature Neuroscience 20:1199–1208.https://doi.org/10.1038/nn.4619
-
Single-neuron diversity generated by Protocadherin-β cluster in mouse central and peripheral nervous systemsFrontiers in Molecular Neuroscience 5:90.https://doi.org/10.3389/fnmol.2012.00090
-
Cortical interneuron development: a tale of time and spaceDevelopment 144:3867–3878.https://doi.org/10.1242/dev.132852
-
Development of GABA innervation in the cerebral and cerebellar corticesNature Reviews Neuroscience 8:673–686.https://doi.org/10.1038/nrn2188
-
Neurotrophins: roles in neuronal development and functionAnnual Review of Neuroscience 24:677–736.https://doi.org/10.1146/annurev.neuro.24.1.677
-
Combinatorial effects of alpha- and Gamma-Protocadherins on neuronal survival and dendritic Self-AvoidanceThe Journal of Neuroscience 38:2713–2729.https://doi.org/10.1523/JNEUROSCI.3035-17.2018
-
Allelic gene regulation of Pcdh-alpha and Pcdh-gamma clusters involving both monoallelic and biallelic expression in single purkinje cellsThe Journal of Biological Chemistry 281:30551–30560.https://doi.org/10.1074/jbc.M605677200
-
Domain-restricted expression of two glutamic acid decarboxylase genes in midgestation mouse embryosThe Journal of Comparative Neurology 424:607–627.https://doi.org/10.1002/1096-9861(20000904)424:4<607::AID-CNE4>3.0.CO;2-C
-
Development and long-term integration of MGE-lineage cortical interneurons in the heterochronic environmentJournal of Neurophysiology 118:131–139.https://doi.org/10.1152/jn.00096.2017
-
Molecular and functional interaction between protocadherin-γC5 and GABAA receptorsJournal of Neuroscience 32:11780–11797.https://doi.org/10.1523/JNEUROSCI.0969-12.2012
-
Subcortical origins of human and monkey neocortical interneuronsNature Neuroscience 16:1588–1597.https://doi.org/10.1038/nn.3536
-
Interneuron dysfunction in psychiatric disordersNature Reviews Neuroscience 13:107–120.https://doi.org/10.1038/nrn3155
-
Programmed cell death and neurotrophic factorsFundamental Neuroscience 32:478–490.https://doi.org/10.1016/j.devcel.2015.01.019
-
Vesicular GABA transporter is necessary for Transplant-Induced critical period plasticity in mouse visual cortexThe Journal of Neuroscience 39:2635–2648.https://doi.org/10.1523/JNEUROSCI.1253-18.2019
-
Model of autism: increased ratio of excitation/inhibition in key neural systemsGenes, Brain and Behavior 2:255–267.https://doi.org/10.1034/j.1601-183X.2003.00037.x
-
Longitudinal organization of the anterior neural plate and neural tubeDevelopment 121:3923–3933.
-
Green fluorescent protein expression and colocalization with calretinin, Parvalbumin, and somatostatin in the GAD67-GFP knock-in mouseThe Journal of Comparative Neurology 467:60–79.https://doi.org/10.1002/cne.10905
-
In utero fate mapping reveals distinct migratory pathways and fates of neurons born in the mammalian basal forebrainDevelopment 128:3759–3771.
-
Fate mapping Nkx2.1-lineage cells in the mouse telencephalonThe Journal of Comparative Neurology 506:16–29.https://doi.org/10.1002/cne.21529
Article and author information
Author details
Funding
National Institutes of Health (R01NS028478)
- Arturo Alvarez-Buylla
National Institutes of Health (EY02517)
- Arturo Alvarez-Buylla
National Institutes of Health (R01DC014101)
- Andrea R Hasenstaub
National Institutes of Health (R01EY025174)
- Michael P Stryker
National Institutes of Health (5F32EY029935)
- Benjamin Rakela
- Michael P Stryker
John G. Bowes Research Fund
- Arturo Alvarez-Buylla
The Klingenstein Foundation
- Andrea R Hasenstaub
Coleman Memorial Fund
- Andrea R Hasenstaub
Hearing Research institute
- Andrea R Hasenstaub
The funders had no role in study design, data collection and interpretation, or the decision to submit the work for publication.
Acknowledgements
We thank Joshua Sanes for kindly proving the Pcdhgfcon3 mice. We also thank Ricardo Romero, Jose Rodrigues, and Cristina Guinto for technical help and John Rubenstein, Arnold Kriegstein, and Duan Xin for the insightful discussion of experimental data. This work was supported by NIH Grants (R01 NS028478, R01 EY02517) and a generous gift from the John G Bowes Research Fund to AAB; NIH Grant R01DC014101 to ARH, the Klingenstein Foundation to ARH, Hearing Research Inc to ARH, and the Coleman Memorial Fund to ARH; NIH Grants R01EY025174 and 5F32EY029935 to MPS and 5F32EY029935 to BR. AAB is the Heather and Melanie Muss Endowed Chair and Professor of Neurological Surgery at UCSF. MPS is a recipient of the Research to Prevent Blindness Disney Award for Amblyopia Research.
Ethics
Animal experimentation: Data presented in this study were acquired following the University of California, San Francisco (UCSF) Institutional Animal Care Committee guidelines under the following protocols: AN178775-02C, AN180588, AN175872.
Copyright
© 2020, Mancia Leon et al.
This article is distributed under the terms of the Creative Commons Attribution License, which permits unrestricted use and redistribution provided that the original author and source are credited.
Metrics
-
- 3,133
- views
-
- 413
- downloads
-
- 40
- citations
Views, downloads and citations are aggregated across all versions of this paper published by eLife.
Download links
Downloads (link to download the article as PDF)
Open citations (links to open the citations from this article in various online reference manager services)
Cite this article (links to download the citations from this article in formats compatible with various reference manager tools)
Further reading
-
- Developmental Biology
Congenital malformations can originate from numerous genetic or non-genetic factors but in most cases the causes are unknown. Genetic disruption of nicotinamide adenine dinucleotide (NAD) de novo synthesis causes multiple malformations, collectively termed Congenital NAD Deficiency Disorder (CNDD), highlighting the necessity of this pathway during embryogenesis. Previous work in mice shows that NAD deficiency perturbs embryonic development specifically when organs are forming. While the pathway is predominantly active in the liver postnatally, the site of activity prior to and during organogenesis is unknown. Here, we used a mouse model of human CNDD and assessed pathway functionality in embryonic livers and extraembryonic tissues via gene expression, enzyme activity and metabolic analyses. We found that the extra-embryonic visceral yolk sac endoderm exclusively synthesises NAD de novo during early organogenesis before the embryonic liver takes over this function. Under CNDD-inducing conditions, visceral yolk sacs had reduced NAD levels and altered NAD-related metabolic profiles, affecting embryo metabolism. Expression of requisite pathway genes is conserved in the equivalent yolk sac cell type in humans. Our findings show that visceral yolk sac-mediated NAD de novo synthesis activity is essential for mouse embryogenesis and its perturbation causes CNDD. As mouse and human yolk sacs are functionally homologous, our data improve the understanding of human congenital malformation causation.
-
- Developmental Biology
- Stem Cells and Regenerative Medicine
The purpose of these studies is to investigate how Sphingosine-1-phosphate (S1P) signaling regulates glial phenotype, dedifferentiation of Müller glia (MG), reprogramming into proliferating MG-derived progenitor cells (MGPCs), and neuronal differentiation of the progeny of MGPCs in the chick retina. We found that S1P-related genes are highly expressed by retinal neurons and glia, and levels of expression were dynamically regulated following retinal damage. Drug treatments that activate S1P receptor 1 (S1PR1) or increase levels of S1P suppressed the formation of MGPCs. Conversely, treatments that inhibit S1PR1 or decrease levels of S1P stimulated the formation of MGPCs. Inhibition of S1P receptors or S1P synthesis significantly enhanced the neuronal differentiation of the progeny of MGPCs. We report that S1P-related gene expression in MG is modulated by microglia and inhibition of S1P receptors or S1P synthesis partially rescues the loss of MGPC formation in damaged retinas missing microglia. Finally, we show that TGFβ/Smad3 signaling in the resting retina maintains S1PR1 expression in MG. We conclude that the S1P signaling is dynamically regulated in MG and MGPCs in the chick retina, and activation of S1P signaling depends, in part, on signals produced by reactive microglia.