Vascular-derived SPARC and SerpinE1 regulate interneuron tangential migration and accelerate functional maturation of human stem cell-derived interneurons
Figures
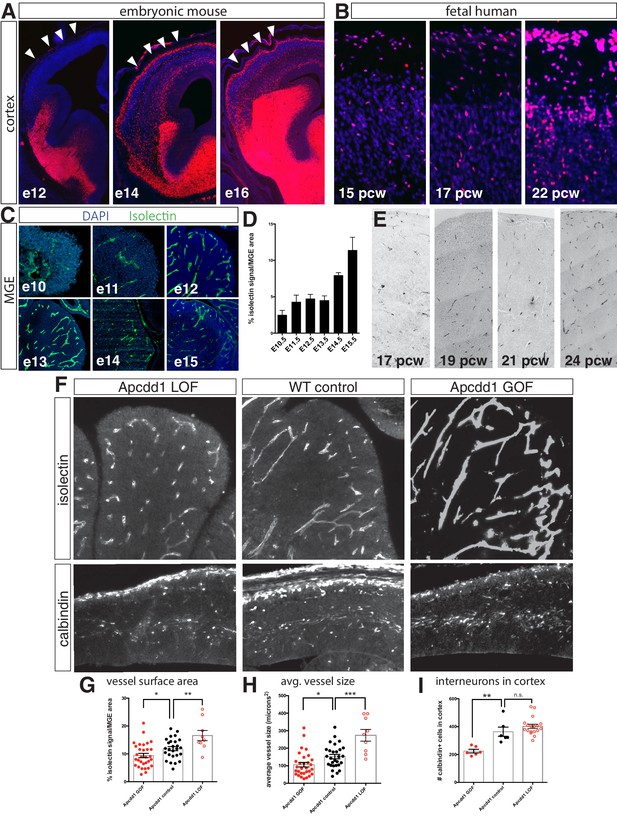
Interneuron migration progresses over development and is regulated by medial ganglionic eminence (MGE) vascularization.
(A) Coronal sections of Dlx6aCre; Ai9 embryonic mouse telencephalon over developmental time. tdTomato + interneurons progressively migrate into the cortex between e12 and e16. Cortex indicated by arrowheads. (B) Dlx2 immunohistochemistry (red) in human fetal cortex shows weeks-long progression of cortical interneurons migrating into cortex. (C) Representative images of isolectin labeling (green). (D) Quantification of isolectin + blood vessel staining as a percentage of MGE area for embryos ages e10.5–e15.5. (E) CD31 immunohistochemistry in human MGE from various fetal ages. (F) Top row, coronal sections of e14.5 MGE. Blood vessels labeled with isolectin in wildtype control, Apcdd1 loss-of-function and Apcdd1 gain-of-function mutants. Bottom row, coronal sections of e14.5 embryonic brain labeled with calbindin to show migratory interneurons in isolectin in wildtype control, Apcdd1 loss-of-function and Apcdd1 gain-of-function mutants. (G, H) Quantification of isolectin + MGE vascularization; (G) isolectin + vessel labeling as a percentage of MGE surface area; (H) average vessel size in square microns. (I) Quantification of total interneurons migrating into cortex/20 μm section. Paired t-test, *p<0.05; **p<0.01; ***p<0.001.
-
Figure 1—source data 1
source data for Figure 1.
- https://cdn.elifesciences.org/articles/56063/elife-56063-fig1-data1-v2.xlsx
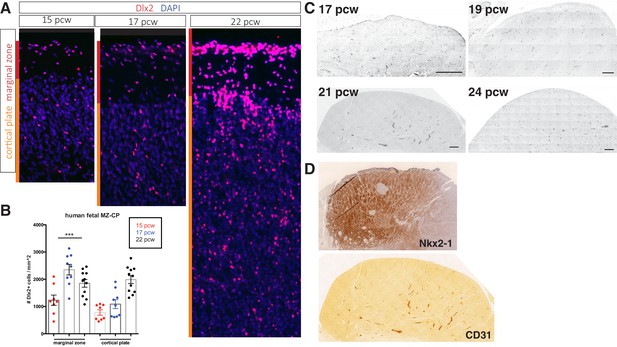
Cortical interneurons populate the human fetal cortex over a protracted period.
(A) Representative immunostaining for Dlx2 (red) in coronal sections of human fetal brain at 15, 17, and 22 post-conception weeks (pcw). Red bar denotes marginal zone (MZ), orange bar denotes cortical plate (CP). (B) Quantification of Dlx2 + interneuron cell density/mm2. (c) Dlx2 cell density (/mm2) present in MZ and CP. (C) Transverse sections of human fetal medial ganglionic eminence (MGE) at 17, 19, and 24 pcw immunolabeled for CD31. Scale bar denotes 200 μm. (D) Adjacent 21 pcw section confirming tissue is MGE by Nkx2-1 immunohistochemistry (top), CD31 IHC (bottom).
-
Figure 1—figure supplement 1—source data 1
source data for Figure 1—figure supplement 1.
- https://cdn.elifesciences.org/articles/56063/elife-56063-fig1-figsupp1-data1-v2.xlsx
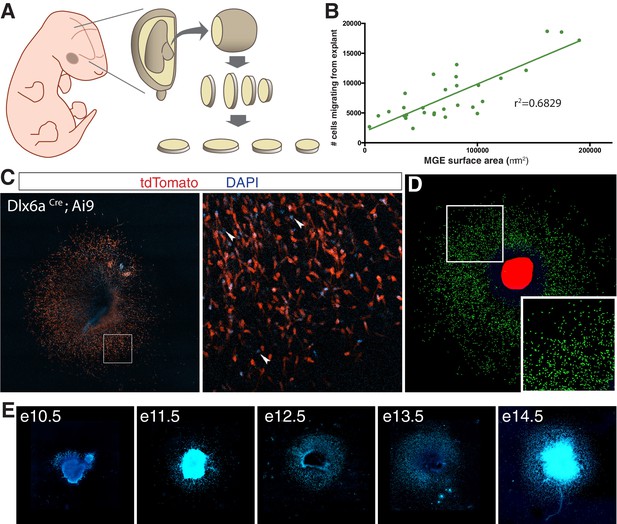
Medial ganglionic eminence (MGE) explants cultured from mouse embryos at various ages.
(A) Schematic representation of MGE explant preparation. MGE is dissected from embryo and sectioned at 250 μm. (B) Interneuron migration in e11.5 MGE explants linear correlation with surface area of MGE explant. (C) e14.5 MGE explant cultured from Dlx6aCre; Ai9 embryo. Right, higher magnification of boxed region on left. Arrowheads show DAPI + cells that are tdTomato-negative. (D) Computer segmented DAPI+ nuclei prior to quantification. (E) Representative DAPI-labeled MGE explants from embryos ages e10.5–14.5.
-
Figure 1—figure supplement 2—source data 1
source data for Figure 1—figure supplement 2.
- https://cdn.elifesciences.org/articles/56063/elife-56063-fig1-figsupp2-data1-v2.xlsx
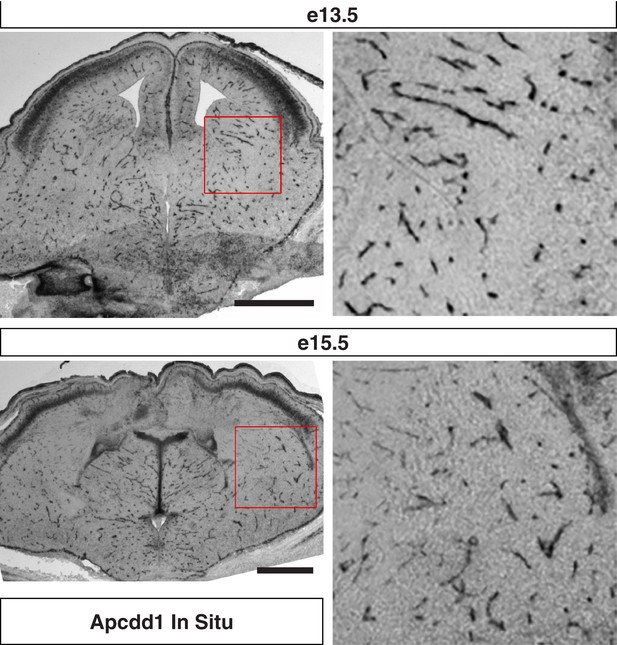
Apcdd1 in situ hybridization signal is confined to endothelial cells in the developing mouse telencephalon.
Coronal sections of (top) e13.5 and (bottom) e15.5 mouse telencephalon labeled with in situ hybridization antisense probe for Apcdd1. Higher magnifications of red boxed regions shows subpallium and is consistent with endothelial cell expression of Apcdd1. Scale bar denotes 100 μm.
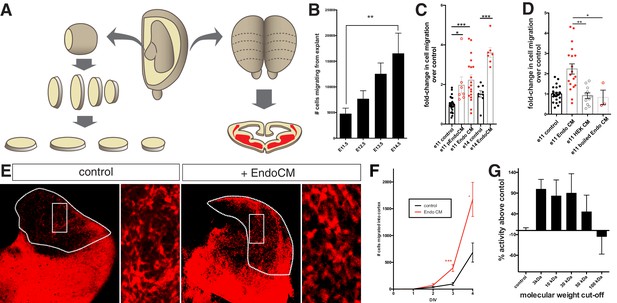
Endothelial cell conditioned medium increases interneuron migration in medial ganglionic eminence (MGE) explants and organotypic slice cultures.
(A) Schematic representation of MGE explant and organotypic slice preparation to assess interneuron migration in vitro. (B) Number of interneurons (normalized to MGE surface area) migrating from MGE explants from embryos ages e10.5–e15.5. (C) Number of interneurons (normalized to MGE surface area) migrating from e11.5 and e14.5 MGE explants with or without primary culture endothelial cell conditioned medium (p-EndoCM) or immortalized endothelial cell line conditioned medium (EndoCM). (D) Number of interneurons (normalized to MGE SA) migrating from e11.5 MGE explants treated with control, EndoCM, HEK 293 conditioned medium (HEK CM) or boiled EndoCM. (E) Representative images of DIV4 organotypic slice cultures without (control) or with EndoCM added. Right is higher magnification of boxed region on left. (F) Number of Dlx6aCre; Ai9 tdTomato + interneurons migrating into cortex over time in coronally section organotypic slice cultures (DIV 0–4) with or without EndoCM treatment. (G) Size fractionation of EndoCM assayed for normalized interneuron migration from e11.5 MGE explants. Paired t-test, *p<0.05; **p<0.01; ***p<0.001.
-
Figure 2—source data 1
source data for Figure 2.
- https://cdn.elifesciences.org/articles/56063/elife-56063-fig2-data1-v2.xlsx
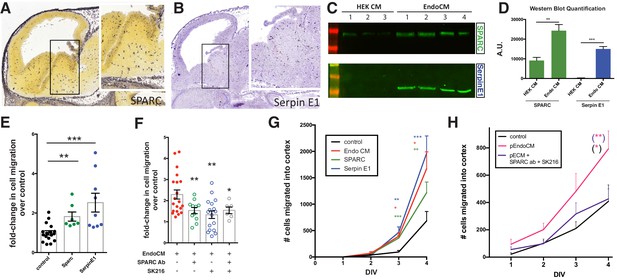
Endothelially derived factors, SPARC and SerpinE1, increase interneuron migration and account for most of the biological activity of endothelial cell line conditioned medium (EndoCM).
(A, B) Parasaggital sections of e13.5 embryonic brain showing in situ hybridization signal for (A) SPARC* and (B) SerpinE1#. Insets for (a) and (b) are higher magnification or boxed regions showing medial ganglionic eminence (MGE) expression. (C) Western blots of HEK 293 conditioned medium (HEK CM) (three replicates) and EndoCM (four replicates) for SPARC and SerpinE1. (D) Quantification of western band intensity for SPARC (green) and SerpinE1 (blue) in HEK CM and EndoCM. (E) Quantification of normalized interneuron migration from MGE explants treated with SPARC (filled green), SerpinE1 (filled blue) compared with control (filled black). (F) Quantification of normalized interneuron migration from MGE explants treated with EndoCM (filled red), EndoCM and SPARC function-blocking antibody (unfilled green), EndoCM and SerpinE1 small molecule inhibitor (unfilled blue), and EndoCM with combination of SPARC function-blocking antibody and SerpinE1 small molecule inhibitor (unfilled gray). (G) Number of Dlx6aCre; Ai9 tdTomato + interneurons migrating into cortex over time in coronally section organotypic slice cultures (DIV 0–4) with or without EndoCM, SPARC or SerpinE1 treatment. (H) Similar organotypic slice culture experiments as in (G) with or without primary EndoCM (p-EndoCM), or p-EndoCM depleted with SPARC function-blocking antibody and SerpinE1 small molecule inhibitor, SK216. Paired t-test, *p<0.05; **p<0.01; ***p<0.001. * from Allen Brain Atlas (http://developingmouse.brain-map.org/); # from GenePaint (http://gp3.mpg.de).
-
Figure 3—source data 1
source data for Figure 3.
- https://cdn.elifesciences.org/articles/56063/elife-56063-fig3-data1-v2.xlsx
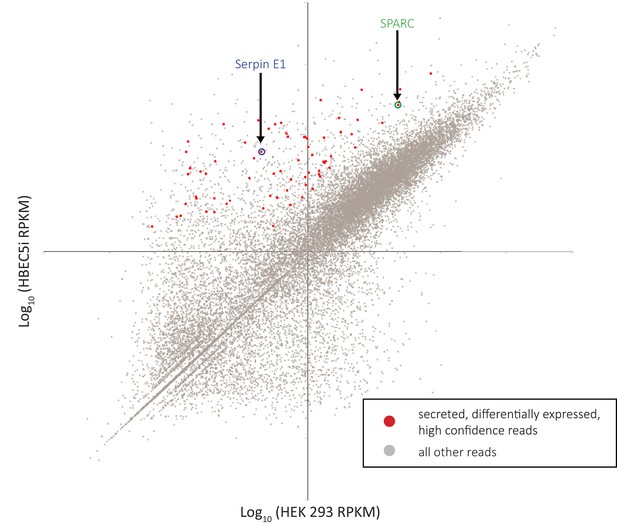
RNA-sequencing (RNA-seq) analysis identifies SPARC and SerpinE1 as highly enriched secreted factors in endothelial cell line conditioned medium (EndoCM) versus HEK 293 conditioned medium (HEK CM).
Scatterplot of RNA-seq reads plotting log10(HEK 293) sample versus log10(HBEC5i) sample. Red dots are HBEC5i-enriched secreted factor hits. All other reads are gray dots. SerpinE1 circled in blue. SPARC circled in green.
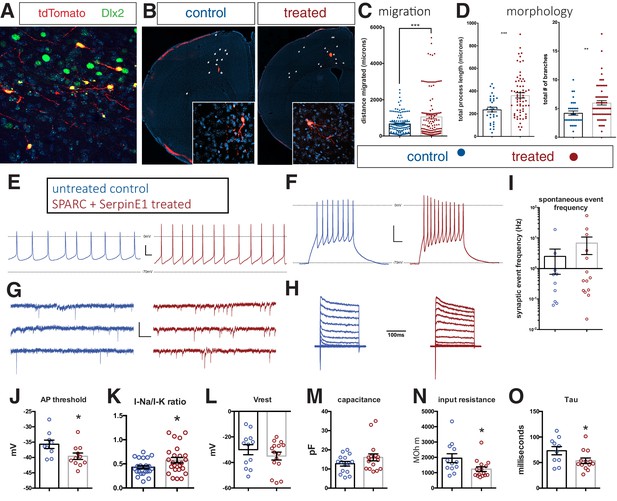
Human stem cell-derived interneurons (hSC-interneurons) xenografted into host mouse cortex are more migratory and more morphologically complex with SPARC and SerpinE1 pre-treatment.
(A) Immunohistochemistry for Dlx2 (green), Dapi (blue), and tdTomato + hSC interneurons (red) near injection site 1 month post-transplant. (B) Representative images of coronal sections of host mouse cortex 1 month post-transplant of control untreated and SPARC/SerpinE1 pre-treated hSC-interneurons (asterisk denotes transplant site). Arrowheads show tdTomato + hSC interneurons migrating away from transplant site. (C) Quantification of migratory distance of hSC-interneurons from site of injection 1 month post-transplantation. (D) Quantification of hSC-interneuron morphology by average process length (left) and total number of neurite branches (right). (E–O) Whole-cell recordings of hSC-interneurons 2 months post-transplant. Untreated control (blue), SPARC and SerpinE1 pre-treated (red). (E) Representative traces of spontaneous AP firing. (F) Evoked firing upon square pulse depolarization. (G) Representative traces of spontaneous miniature EPSCs. (H) Step recordings of sodium current. (I–O) Quantitative measurements of (I) spontaneous mEPSC frequency (Hz), (J) AP threshold (mV) (p=0.0166), (K) sodium-to-potassium current ratio (p=0.0338), (L) resting membrane potential (p=0.12), (M) capacitance (p=0.067), (N) input resistance (p=0.0155), (O) tau (p=0.0239). Paired t-test, *p<0.05; **p<0.01; ***p<0.001.
-
Figure 4—source data 1
source data for Figure 4.
- https://cdn.elifesciences.org/articles/56063/elife-56063-fig4-data1-v2.xlsx
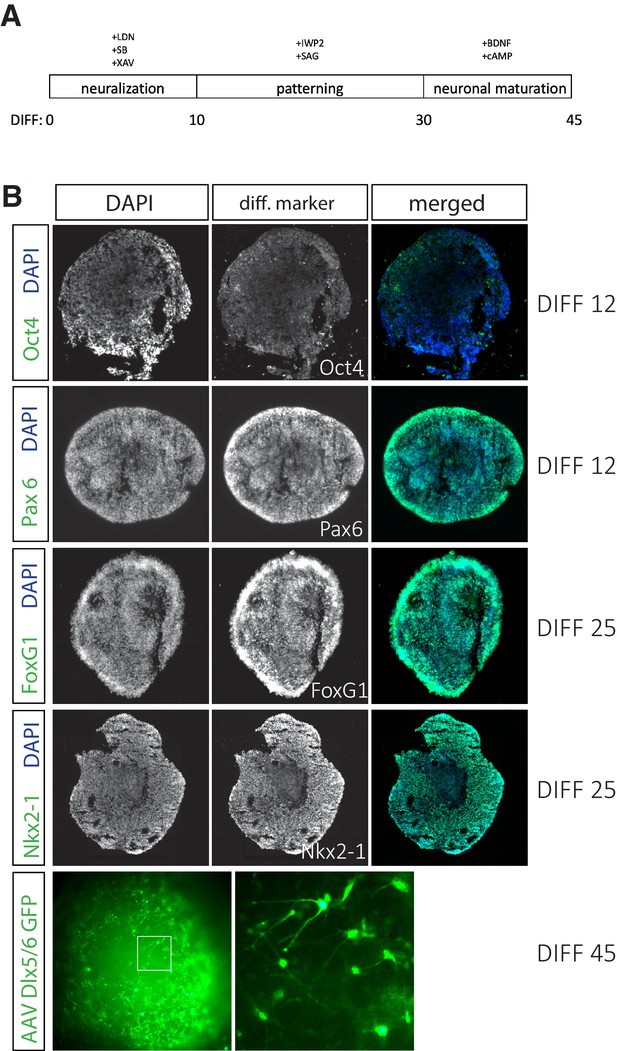
Human stem cells (hSCs) efficiently differentiate into interneurons.
(A) Time line for hSC differentiation to cortical interneuron fate. (B) Differentiation day (DIFF) 12 organoids sectioned at 16 μm are largely Oct4-negative and almost completely Pax6-positive, indicating successful neural differentiation. DIFF 25 organoids sectioned at 16 μm are FoxG1- and Nkx2-1-positive. DIFF 45 whole organoid labeled with AAV-Dlx5/6-eGFP shows efficient differentiation to interneuron cell fate.
-
Figure 4—figure supplement 1—source data 1
source data for Figure 4—figure supplement 1.
- https://cdn.elifesciences.org/articles/56063/elife-56063-fig4-figsupp1-data1-v2.xlsx
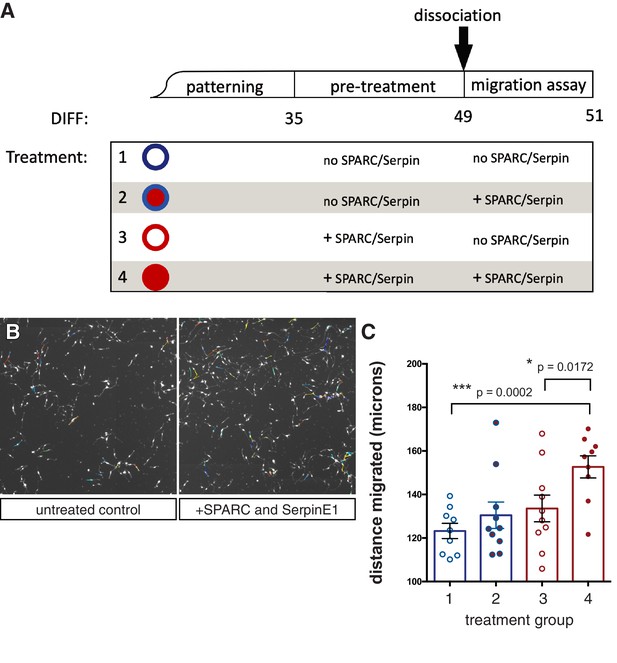
Human stem cell-derived interneurons (hSC-interneurons) migrate further in vitro with SPARC and SerpinE1 treatment.
(A) Schematic of in vitro treatment groups for hSC-interneurons with SPARC and SerpinE1. (B) Example image of live tracking of hSC-interneurons plated as monolayer. (C) Quantification of hSC-interneuron treatment groups for total migratory distance over 48 hr. Paired t-test, *p<0.05; **p<0.01; ***p<0.001.
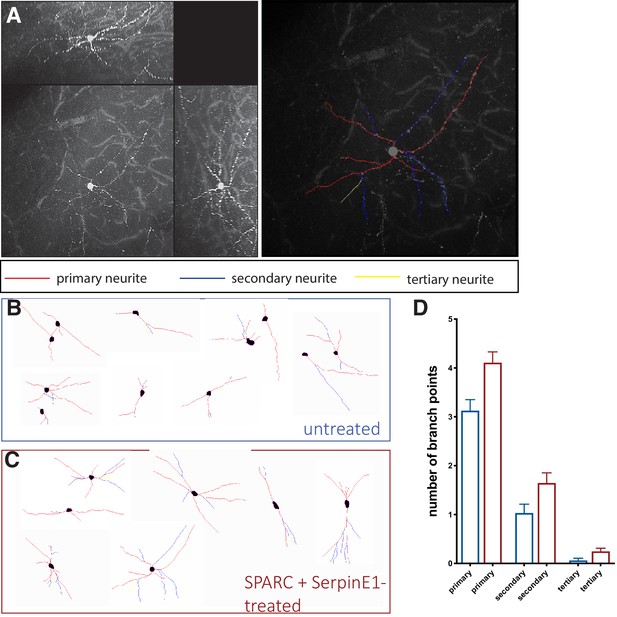
SPARC and SerpinE1-pre-treated human stem cell-derived interneurons (hSC-interneurons) exhibit more complex morphologies after xenotransplantation into host mouse cortex.
(A) Example of traced hSC-interneuron in cortex 2 months post-transplant. Primary neurite in red, secondary neurite in blue, tertiary neurite in yellow. (B, C) Representative traces of cell morphology for (B) untreated control and (C) SPARC/SerpinE1 pre-treated hSC-interneurons 2 months post-transplant. (D) Quantification of branch points in primary, secondary, and tertiary process for untreated control and SPARC/SerpinE1 pre-treated groups.
-
Figure 4—figure supplement 3—source data 1
source data for Figure 4—figure supplement 3.
- https://cdn.elifesciences.org/articles/56063/elife-56063-fig4-figsupp3-data1-v2.xlsx
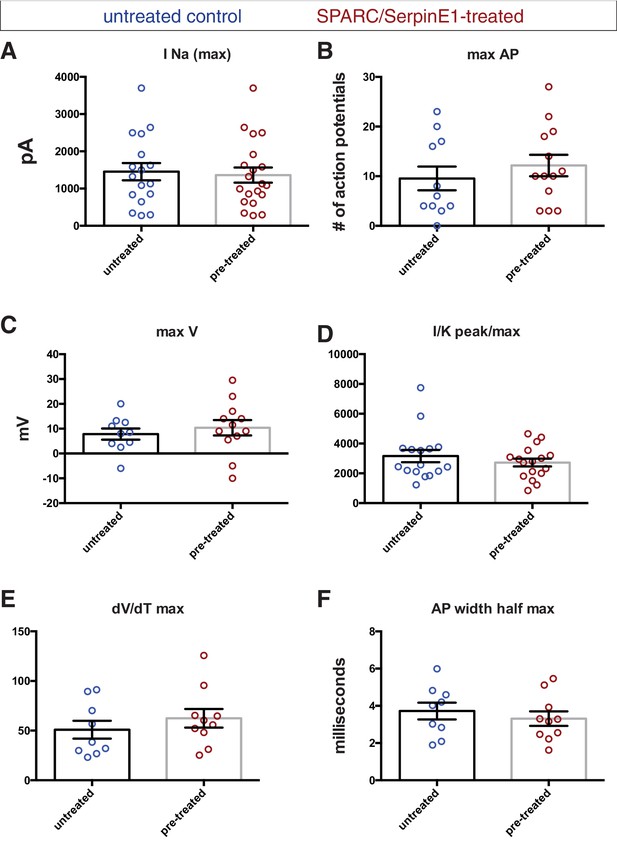
Electrophysiological recordings of control and pre-treated human stem cell-derived interneurons (hSC-interneurons) show pre-treated group trends toward greater maturity by most measures.
Untreated control (blue) and pre-treated (red) hSC-interneurons measured for (A) sodium current (pico-amps), (B) maximum number of action potentials (APs) elicited by square pulse depolarization, (C) maximum AP depolarization (milli-volts), (D) sodium-to-potassium peak current/max AP depolarization, (E) AP risetime, (F) AP width at half maximum amplitude (milliseconds).
-
Figure 4—figure supplement 4—source data 1
source data for Figure 4—figure supplement 4.
- https://cdn.elifesciences.org/articles/56063/elife-56063-fig4-figsupp4-data1-v2.xlsx
Additional files
-
Supplementary file 1
Top differentially expressed genes between HEK 293 and HBEC-5i.
HEK 293 and HBEC-5i cell lines were pre-treated in serum-free medium as if they were being used to collect conditioned medium. Cells were lysed and RNA was collected for bulk RNA-sequencing (RNA-seq) analysis. Top differentially expressed genes were screened by protein size and using the Gene Ontology (GO) term extracellular space in order to identify candidates listed in this table.
- https://cdn.elifesciences.org/articles/56063/elife-56063-supp1-v2.docx
-
Transparent reporting form
- https://cdn.elifesciences.org/articles/56063/elife-56063-transrepform-v2.docx