A two-lane mechanism for selective biological ammonium transport
Figures
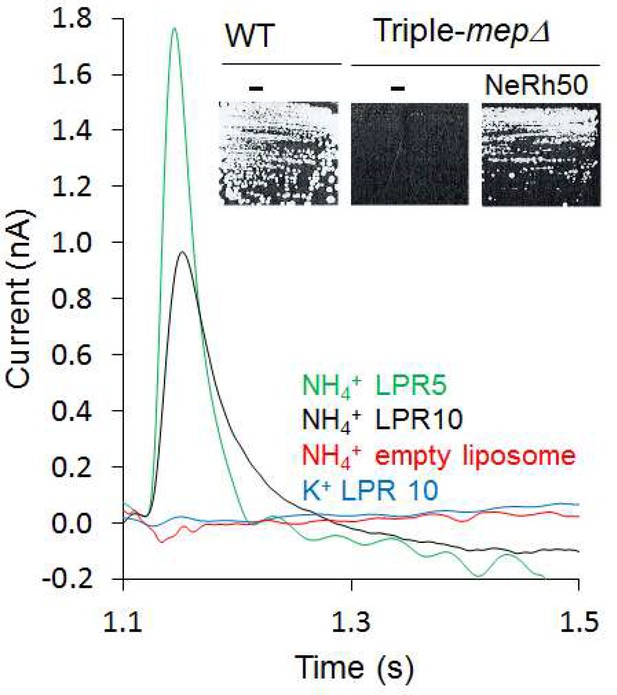
Characterization of the activity of NeRh50.
Transient current measured using SSME after a 200 mM pulse (ammonium or potassium). Insert: Yeast complementation by NeRh50 (strain 31019b, mep1Δ mep2Δ mep3Δ ura3) on minimal medium supplemented with 3 mM ammonium as sole nitrogen source.
-
Figure 1—source data 1
Characterization of the activity of NeRh50.
- https://cdn.elifesciences.org/articles/57183/elife-57183-fig1-data1-v3.xlsx
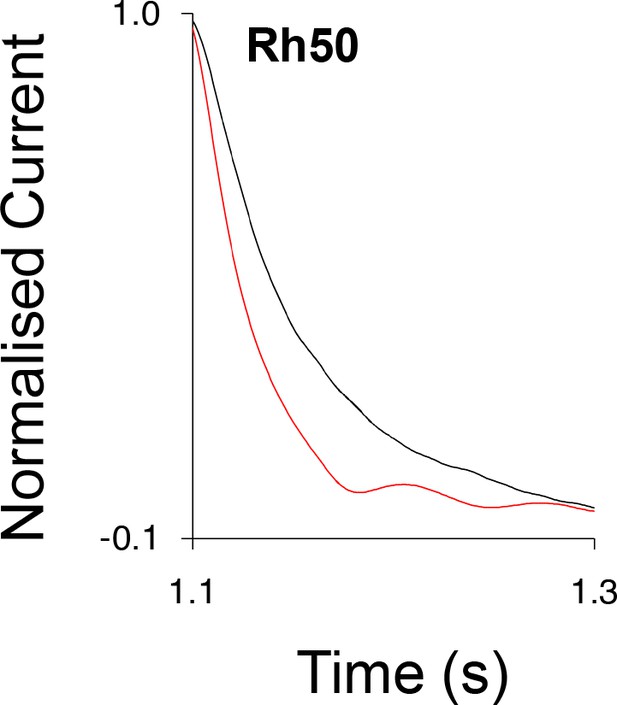
Characterization of the activity of NeRh50.
Normalized traces following a 200 mM ammonium pulse in proteoliposomes containing NeRh50 at LPR 10 (black) or LPR 5 (red). Traces have been normalized based on their own maximum amplitude to permit comparison. eight sensors from two independent protein purification batches were measured, with three measurements recorded for each sensor. The average value are given Table 2. Single representative trace were chosen to visualize the results.
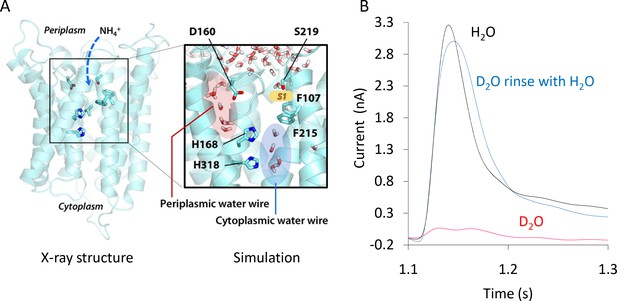
Formation and functionality of the periplasmic (PWW) and cytoplasmic (CWW) water wires in AmtB.
(A) Extended atomistic simulations show a hydration pattern across the protein, in which cytoplasmic and periplasmic water wires, connected via H168, form a continuous pathway for proton transfer from the S1 NH4+ sequestration region to the cytoplasm. (B) Transient currents measured following a 200 mM ammonium pulse on sensors prepared with solutions containing either H2O (black) or D2O (red). D2O sensors were rinsed with H2O solutions and subsequently exposed to another 200 mM ammonium pulse (blue).
-
Figure 2—source data 1
Functionality of the periplasmic (PWW) and cytoplasmic (CWW) water wires in AmtB.
- https://cdn.elifesciences.org/articles/57183/elife-57183-fig2-data1-v3.xlsx
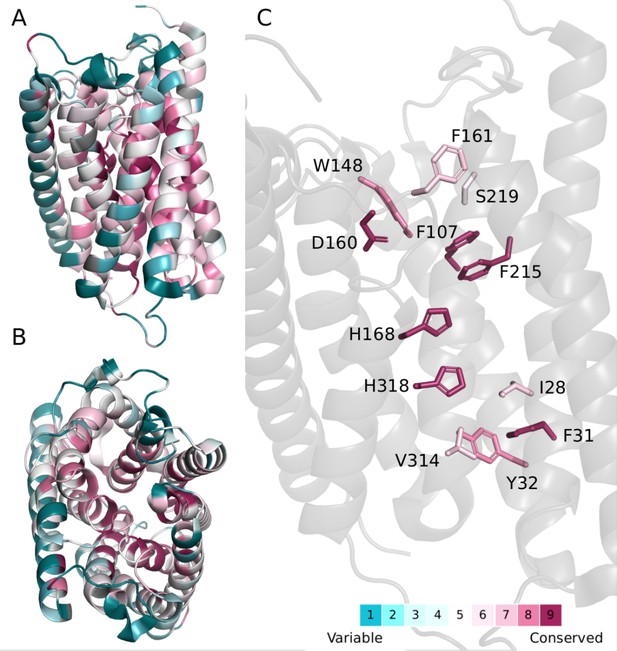
Evolutionary conservation of the proton and hydrophobic pathways for H+ and NH3 translocation in AmtB.
Evolutionary conservation scores indicate that the residues that line both the water wires and the internal hydrophobic pathway show a large degree of conservation among homologous proteins. Evolutionary conservation scores were estimated using the ConSurf server (Ashkenazy et al., 2016). In brief, a multiple sequence alignment (MSA) was built of homologues collected from the SWISS-PROT database. Homologues were identified by five iterations of HMMER, producing an MSA of 64 sequences. The evolutionary conservation scores are projected onto an AmtB monomer, (PDB code: 1U7G), with some helices removed for clarity. (A) Side view. (B) Top view from periplasmic side. (C) Close-up view of key residues involved in the two-lane mechanism.
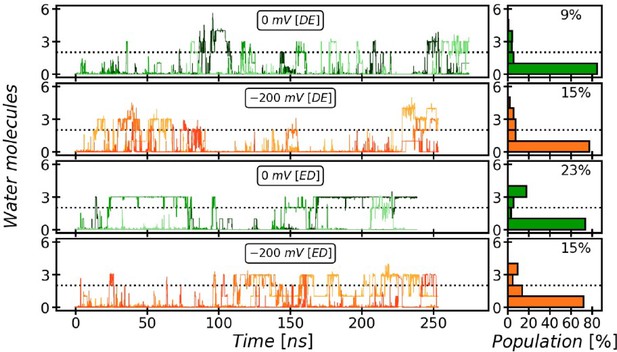
Evolution and occupancy of the Periplasmic Water Wire (PWW).
The periplasmic water wire evolves on a slower time scale than the CWW and shows greater fluctuations. It is stabilized by the ED twin-His tautomeric state, where 23% occupancy of the PWW with three water molecules or more is observed. Stably occupied states are seen after ~25 ns simulated time. Since earlier simulations studying the internal hydration of AmtB were shorter (Lamoureux et al., 2007), this may explain why the PWW has not been described previously. A significant influence of membrane voltage of a magnitude that is biologically relevant for the cytoplasmic membrane of E. coli is not seen.
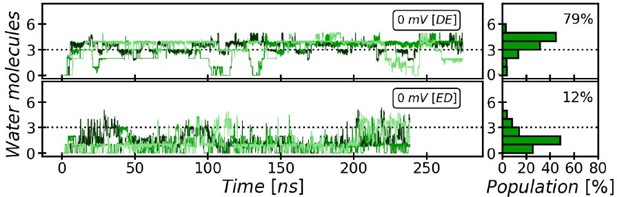
Evolution and occupancy of the Cytoplasmic Water Wire (CWW).
The cytoplasmic water wire develops in an early stage of the simulations, as has been previously observed (Lamoureux et al., 2007). The CWW is particularly stabilized in the DE twin-His tautomeric state, where the occupancy is 79% on average with at least three water molecules over the course of our simulations.
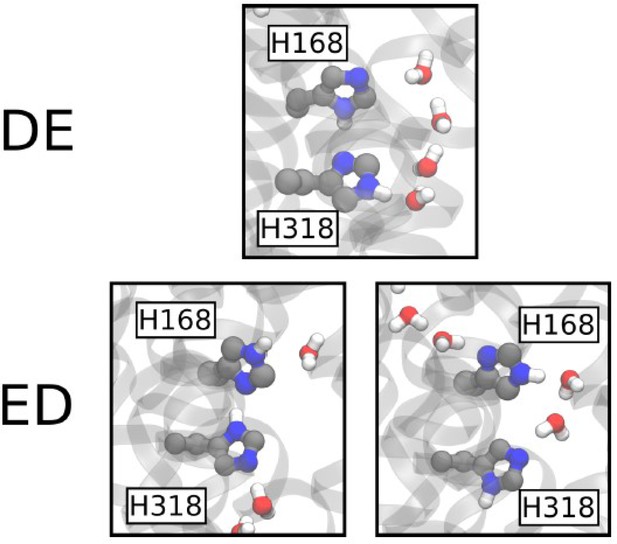
The DE and ED twin-His motif configurations.
Nomenclature as in Lamoureux et al., 2007. The top panel shows the configuration of H168 and H318 observed in the DE simulations, with the protonated Nδ atom of H168 pointing towards the Nδ atom of H318. The bottom panel shows the two distinct configurations observed in the ED simulations; on the left is the equivalent of the situation observed in DE, while on the right the H168 sidechain flips and its Nδ atom contacts the most proximal molecule of the PWW.
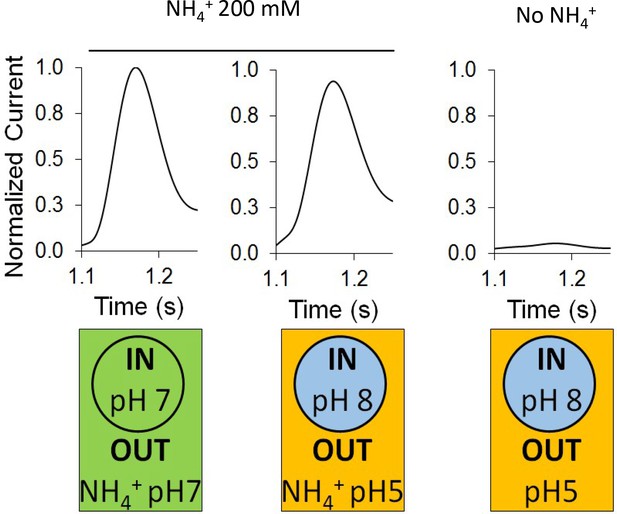
Effect of a proton gradient on AmtB activity.
The transient currents were measured using SSME following an ammonium pulse of 200 mM at pH 7 (left) or under an inwardly directed pH gradient in the presence (center) or absence (right) of ammonium. eight sensors from two independent protein purification batches were measured, with three measurements recorded for each sensor. Single representative traces were chosen to visualize the results. Each sensor was measured in the order pH (in/out) 7/7, 8/5, 8/5 (this time without NH4+), and finally 7/7 again to be sure that the signals do not significantly decrease with time. The data are normalized against the measurements done at pH7 in/out for each sensor.
-
Figure 3—source data 1
Effect of a proton gradient on AmtB activity.
- https://cdn.elifesciences.org/articles/57183/elife-57183-fig3-data1-v3.xlsx
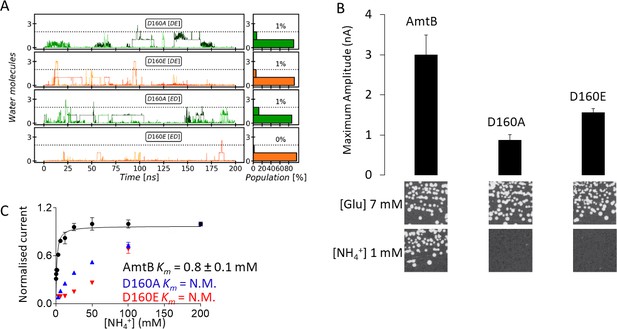
Effect of D160 substitutions.
(A) The Periplasmic Water Wire (PWW) in the D160A and D160E variants. We observe no significant occupancy of the PWW above the threshold of at least three water molecules in the D160A and D160E AmtB variants, irrespective of the tautomeric protonation states of H168 and H318 (DE or ED, see Materials and method section). (B) Upper panel: maximum amplitude of the transient current measured using SSME following a 200 mM ammonium pulse. Eight sensors from two independent protein purification batches were measured, with three measurements recorded for each sensor (means ± SD). Lower panel: yeast complementation test (strain 31019b, mep1Δ mep2Δ mep3Δ ura3) using 7 mM Glutamate (Glu) or 1 mM ammonium as a sole nitrogen source. The growth tests have been repeated twice. (C) Kinetics analysis of the transport of ammonium. The maximum amplitudes recorded after a 200 mM ammonium pulse have been normalized to 1.0 for comparison. N.M.: Non Measurable. eight sensors from two independent protein purification batches were measured, with three measurements recorded for each sensor (means ± SD).
-
Figure 4—source data 1
Effect of D160 substitutions on AmtB activity measured by SSME.
- https://cdn.elifesciences.org/articles/57183/elife-57183-fig4-data1-v3.xlsx
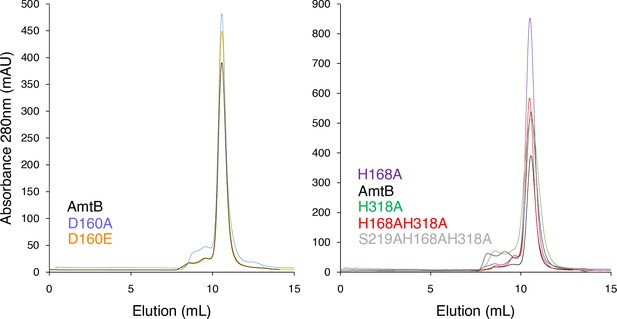
Size Exclusion Chromatography analysis of AmtB.
Gel filtration trace (Superdex 200 10/300 increase) of wild-type AmtB and variants after solubilization of the proteoliposome in 2% DDM. All of the AmtB variants elute at ~11.5 ml.
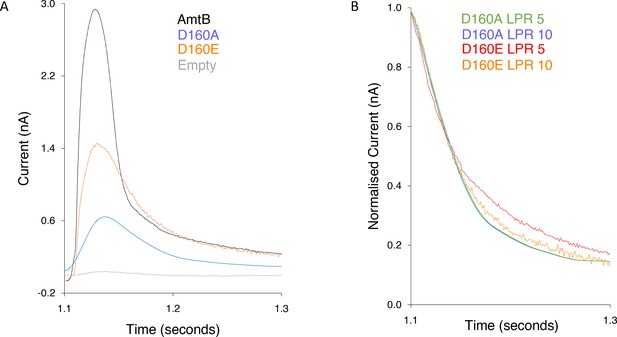
Characterization of the activity and specificity of AmtB variants.
(A) SSME analysis. Transient current measured after a 200 mM pulse of ammonium in proteoliposomes containing AmtB (black), AmtBD160A (blue), or AmtBD160E (orange) at LPR 10. (B) Normalized traces following a 200 mM ammonium pulse in proteoliposomes containing AmtBD160E at LPR 10 (orange), AmtBD160E at LPR 5 (red), AmtBD160A at LPR 10 (blue), and AmtBD160A at LPR 5 (green). Eight sensors from two independent protein purification batches were measured, with three measurements recorded for each sensor. Single representative traces were chosen to visualize the results.
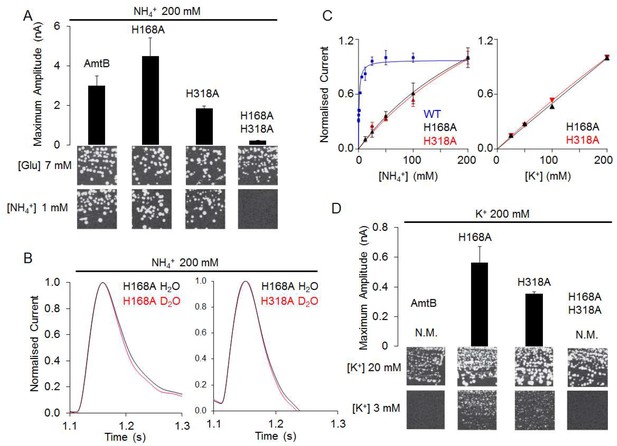
The AmtBH168A and AmtBH318A lose their specificity toward ammonium.
(A) Upper panels: maximum amplitude of the transient current measure using SSME after a 200 mM ammonium pulse. Eight sensors from two independent protein purification batches were measured, with three measurements recorded for each sensor (means ± SD). Lower panels: yeast complementation test (strain 31019b, mep1Δ mep2Δ mep3Δ ura3) using 7 mM Glutamate (Glu) or 1 mM ammonium as a sole nitrogen source. The growth tests have been repeated twice. (B) Transient currents measured using SSME following a 200 mM ammonium pulse on sensors prepared with solutions containing either H2O (black) or D2O (red). The maximum amplitudes recorded after a 200 mM ammonium pulse on sensor prepared in H2O have been normalized to 1.0 for comparison. eight sensors from two independent protein purification batches were measured, with three measurements recorded for each sensor (means ± SD). (C) Kinetics analysis of the transport of NH4+ (or K+ in AmtBH168A (black), AmtBH318A (red) and WT-AmtB (bleu, only for NH4+, as no signal was measurable with K+). The maximum amplitudes recorded after a 200 mM NH4+ or K+ pulse have been normalized to 1.0 for comparison. Eight sensors from two independent protein purification batches were measured, with three measurements recorded for each sensor (means ± SD). (D) Upper panels: maximum amplitude of the transient current measured using SSME after a 200 mM potassium pulse. N.M. Non Measurable. Eight sensors from two independent protein purification batches were measured, with three measurements recorded for each sensor (means ± SD). Lower panels: yeast complementation test (strain #228, mep1Δ mep2Δ mep3Δ trk1Δ trk2Δ leu2 ura3) using media supplemented with 20 mM or 3 mM KCl. The growth test has been repeated twice.
-
Figure 5—source data 1
Effect of H168 and/or H318 substitution on AmtB activity and selectivity measured by SSME.
- https://cdn.elifesciences.org/articles/57183/elife-57183-fig5-data1-v3.xlsx
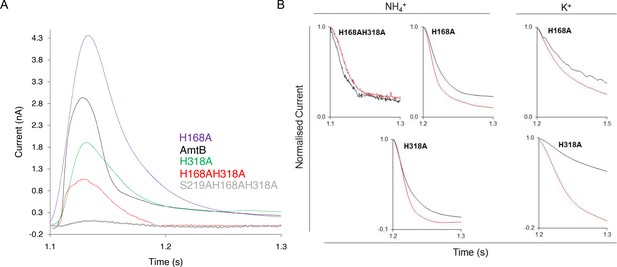
Characterization of the activity and specificity of AmtB variants.
(A) SSME analysis. Transient current measured after a 200 mM pulse of ammonium in proteoliposomes containing AmtB (black), AmtBH168A (purple), AmtBH318A (green), AmtBH168AH318A (red), AmtBS219AH168AH318A (grey) at LPR 10. (B) Normalized traces following a 200 mM ammonium or K+ pulse in proteoliposomes containing various AmtB variant at LPR 10 (black) or LPR 5 (red). Traces have been normalized based on their own maximum amplitude to permit comparison. eight sensors from two independent protein purification batches were measured, with three measurements recorded for each sensor. The average value are given Table 2. Single representative trace were chosen to visualize the results.
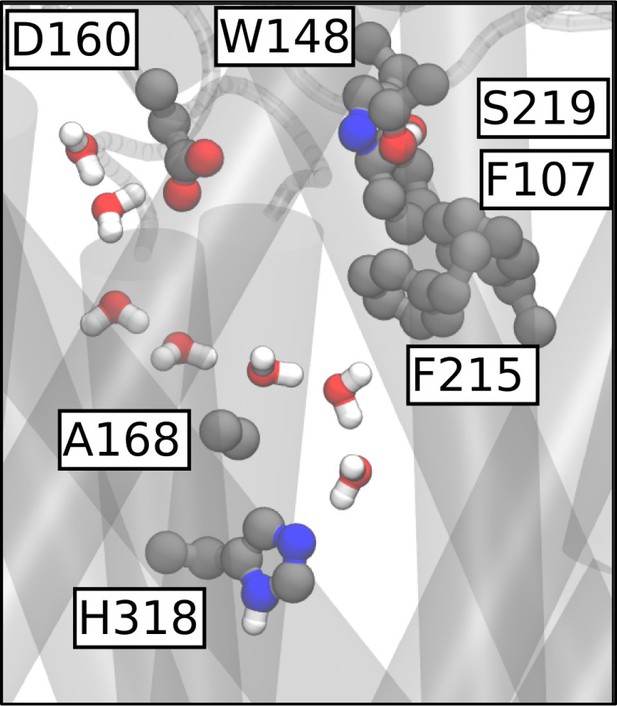
MD simulation of AmtBH168A showing formation of a continuous water wire traversing the central pore region.
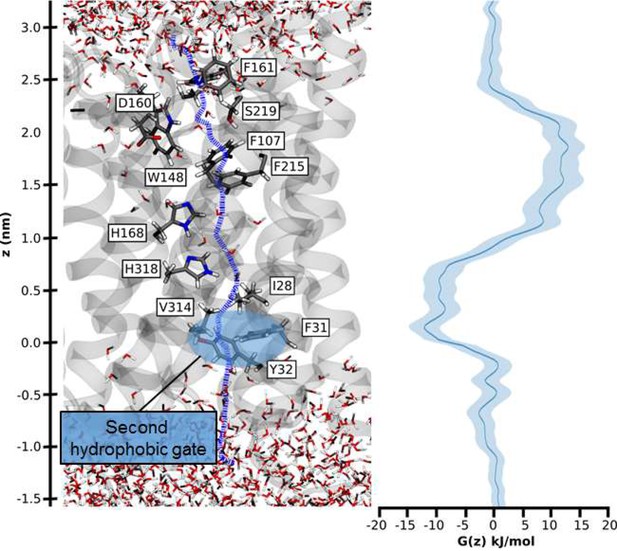
Hydrophobic pathway and energetics for NH3 translocation in AmtB.
We probed an optimal pathway for NH3 transfer during our PMF calculations (left, purple dash trajectory) in the presence of both the PWW and CWW. The software HOLE (49) was used to determine the most likely transfer route. The pathway from the periplasm to the cytoplasm traverses the hydrophobic gate region (F107 and F215), crosses the cavity next to the twin-His motif (H168 and H318) occupied by the CWW, and continues across a second hydrophobic region (I28, V314, F31, Y32) before entering the cytoplasm.
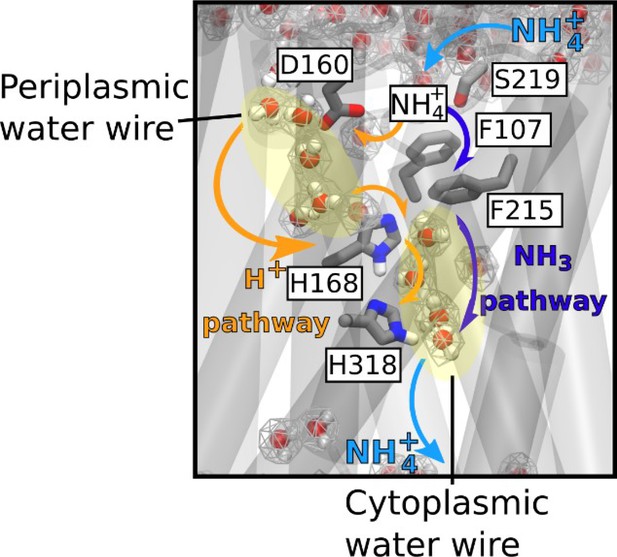
Mechanism of electrogenic NH4+ translocation in AmtB.
Following sequestration of NH4+ at the periplasmic face, NH4+ is deprotonated and H+ and NH3 follow two separated pathways to the cytoplasm (orange arrows depict the pathway for H+ transfer, dark blue arrows for NH3), facilitated by the presence of two internal water wires. NH3 reprotonation likely occurs near the cytoplasmic exit (Figure 6). The hydrated regions within the protein as observed in simulations are highlighted by wireframe representation, crucial residues involved in the transport mechanism are shown as sticks.
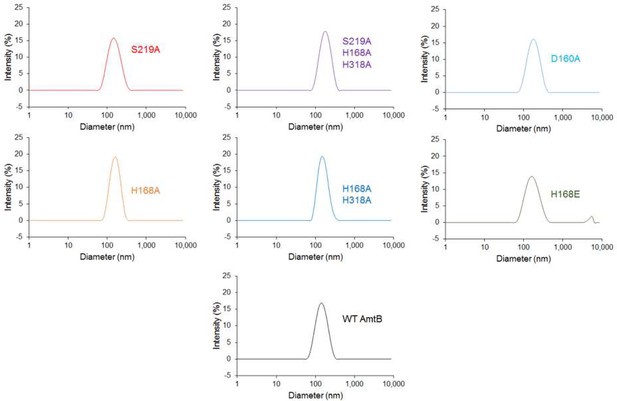
Size distribution of the proteoliposomes containing wild-type and variants of AmtB.
Dynamic light scattering was used to determine the number-weighted distribution of liposome sizes in the detection reagent. The distribution was monodisperse, with a mean diameter of 110 nm.
Tables
Decay time constants (s−1) of transient currents triggered after an ammonium or potassium pulse of 200 mM in proteoliposomes containing AmtB at various LPR*.
NH4+ | K+ | |||
---|---|---|---|---|
Variant | LPR 10 | LPR 5 | LPR 10 | LPR 5 |
AmtB-WT | 13.4 ± 1.5 | 18.7 ± 1.0 | NC | NC |
D160A | 21.6 ± 1.2 | 24.3 ± 1.5 | NC | NC |
D160E | 17.03 ± 2.84 | 19.53 ± 1.8 | NC | NC |
H168A H318A | 29.5 ± 2.1 | 29.8 ± 2.6 | NC | NC |
S219A H168A H318A | NC | NC | NC | NC |
H168A | 28.3 ± 1.5 | 38.0 ± 1.0 | 2.7 ± 0.5 | 5.2 ± 1.0 |
H318A | 22.56 ± 2.63 | 28.25 ± 3.1 | 10.07 ± 1.7 | 15.64 ± 2.1 |
NeRh50 | 24.0 ± 1.7 | 39.0 ± 3.6 | NC | NC |
-
*NC: No transient current recorded.
-
Table 1—source data 1
Decay time constants (s−1) of transient currents triggered after an ammonium or potassium pulse of 200 mM measured by SSME.
- https://cdn.elifesciences.org/articles/57183/elife-57183-table1-data1-v3.xlsx
Free energies for proton translocation through the cytoplasmic and periplasmic water wires and neighboring water molecules (bulk)*.
Z (Å) | Free energy (kJ/mol) | |||
---|---|---|---|---|
(bulk) | Peripl. water wire | wat1 | 14.7 | 0.0 |
wat2 | 12.7 | 8.7 | ||
wat3 | 10.7 | 15.0 | ||
wat4 | 8.3 | 14.4 | ||
wat5 | 6.1 | 7.5 | ||
D160 | wat6 | 5.4 | 11.0 | |
wat7 | 3.2 | 14.4 | ||
wat8 | 0.6 | 18.5 | ||
H168 | ||||
cytopl. water wire | wat9 | −0.4 | 17.3 | |
wat10 | −0.8 | 14.4 | ||
wat11 | −3.2 | 12.1 | ||
H318 | wat12 | −5.1 | 13.8 |
-
*The vertical coordinate z was calculated relative to the position of the sidechain of H168. Positions of the sidechains of D160, H168 and H318 with respect to the periplasmic and cytoplasmic water wires are indicated in the left column.
Reagent type (species) or resource | Designation | Source or reference | Identifiers | Additional information |
---|---|---|---|---|
Gene (Escherichia coli) | AmtB | Zheng et al., 2004 | Uniprot: C3TLL2 | |
Gene (Nitrosomonas europea) | Rh50 | Lupo et al., 2007 | Uniprot Q82 × 47 | |
Strain, strain background (Escherichia coli) | C43 (DE3) | Miroux and Walker, 1996 | Chemically competent cells | |
Strain, strain background (Escherichia coli) | GT1000 | Javelle et al., 2004 | Chemically competent cells | |
Recombinant DNA reagent | pET22b (+) | Novagen | Cat# - 69744 | |
Recombinant DNA reagent | pDR195 | Rentsch et al., 1995 | Addgene - 36028 | High copy yeast expression vector |
Recombinant DNA reagent | pAD7 | Cherif-Zahar et al., 2007 | pESV2-RH50(His)6 | |
Recombinant DNA reagent | p426MET25 | Mumberg et al., 1994 | ||
Recombinant DNA reagent | PZheng | Zheng et al., 2004 | pET22b-AmtB(His)6 | |
Recombinant DNA reagent | pGDM2 | This study | pET22b-AmtB(His)6H168AH318A | |
Recombinant DNA reagent | pGDM4 | This study | pET22b-AmtB(His)6D160A | |
Recombinant DNA reagent | pGDM5 | This study | pET22b-AmtB(His)6D160E | |
Recombinant DNA reagent | pGDM6 | This study | pET22b-AmtB(His)6S219AH168AH318A | |
Recombinant DNA reagent | pGW2 | This study | pET22b-AmtB(His)6H168A | |
Recombinant DNA reagent | pGDM9 | This study | pDR195-AmtB(His)6D160A | |
Recombinant DNA reagent | pGDM10 | This study | pDR195-AmtB(His)6D160E | |
Recombinant DNA reagent | pGDM12 | This study | pDR195-AmtB(His)6H168AH318A | |
Recombinant DNA reagent | pGDM13 | This study | pDR195-AmtB(His)6S219AH168AH318A | |
Recombinant DNA reagent | pGW7 | This study | pDR195-AmtB(His)6H168A | |
Sequence-based reagent | AmtBS219A F | IDT | PCR Primer (Mutagenesis) | GGTGGCACCGTGGTGGATATTAACGCCGCAATC |
Sequence-based reagent | AmtBD160A F | IDT | PCR Primer (Mutagenesis) | CTCACGGTGCGCTGGCCTTCGCGGGTGGCACC |
Sequence-based reagent | AmtBD160E F | IDT | PCR Primer (Mutagenesis) | CTCACGGTGCGCTGGAGTTCGCGGGTGGCACC |
Sequence-based reagent | AmtBH168A F | IDT | PCR Primer (Mutagenesis) | GGTGGCACCGTGGTGGCCATTAACGCCGCAATC |
Sequence-based reagent | AmtBH318A F | IDT | PCR Primer (Mutagenesis) | TGTCTTCGGTGTGGCCGGCGTTTGTGGCATT |
Sequence-based reagent | AmtB XhoI | IDT | PCR primer | AGTCCTCGAGATGAAGATAGCGACGATAAAA |
Sequence-based reagent | AmtB BamHI | IDT | PCR primer | AGTCGGATCCTCACGCGTTATAGGCATTCTC |
Sequence-based reagent | P5’NeRh | IDT | PCR primer | GCCACTAGTATGAGTAAACACCTATGTTTC |
Sequence-based reagent | P3’NeRh | IDT | PCR primer | GCCGAATTCCTATCCTTCTGACTTGGCAC |
Peptide, recombinant protein | AmtB(His)6 | This study | purified from E. coli C43 (DE3) cells | |
Peptide, recombinant protein | AmtB(His)6D160A | This study | purified from E. coli C43 (DE3) cells | |
Peptide, recombinant protein | AmtB(His)6 D160E | This study | purified from E. coli C43 (DE3) cells | |
Peptide, recombinant protein | AmtB(His)6 H168AH318A | This study | purified from E. coli C43 (DE3) cells | |
Peptide, recombinant protein | AmtB(His)6 S219AH168AH318A | This study | purified from E. coli C43 (DE3) cells | |
Peptide, recombinant protein | AmtB(His)6 H168A | This study | purified from E. coli C43 (DE3) cells | |
Peptide, recombinant protein | AmtB(His)6 H318A | This study | purified from E. coli C43 (DE3) cells | |
Peptide, recombinant protein | NeRh50(His)6 | This study | purified from E. coli C43 GT1000 cells | |
Peptide, recombinant protein | XhoI | Promega | Cat# - R6161 | |
Peptide, recombinant protein | BamHI | Promega | Cat# - R6021 | |
Commercial assay or kit | Quikchange XL site-directed mutagensis kit | Agilent Technologies | Cat# 200516 | |
Chemical compound, drug | n-dodecyl-β-D-maltopyranoside (DDM) | Avanti | Cat#- 850520 | |
Chemical compound, drug | lauryldecylamine oxide (LDAO) | Avanti | Cat#- 850545 | |
Chemical compound, drug | E. coli Polar Lipids | Avanti | Cat#−100600 | |
Chemical compound, drug | Phosphotidylcholine (POPC) | Avanti | Cat#−850457 | |
Software, algorithm | Graphpad Prism software | GraphPad Prism (https://www.graphpad.com) | Version 6.01 | |
Software, algorithm | Origin Pro Software | Origin Labs (https://www.originlab.com) | Origin 2017 Version 94E | |
Software, algorithm | SURFE2R Control Software | Nanion (https://www.nanion.de/en/) | V1.5.3.2 |
Additional files
-
Supplementary file 1
Supplementary Table 1.
- https://cdn.elifesciences.org/articles/57183/elife-57183-supp1-v3.docx
-
Transparent reporting form
- https://cdn.elifesciences.org/articles/57183/elife-57183-transrepform-v3.docx