Genomic innovation of ATD alleviates mistranslation associated with multicellularity in Animalia
Figures
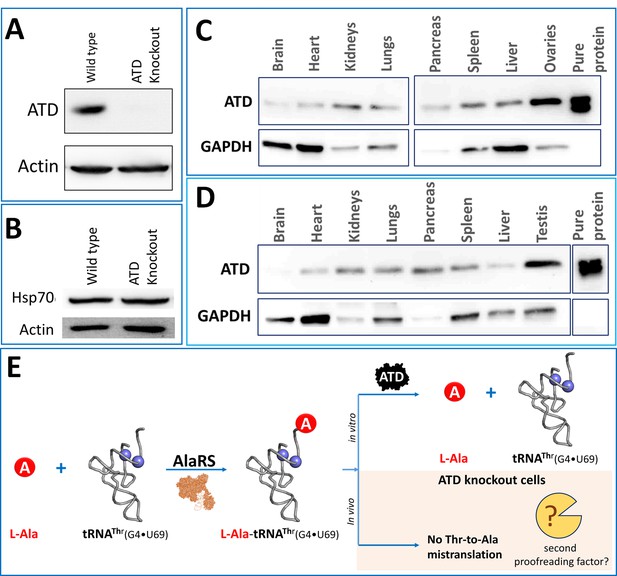
Unperturbed proteome homeostasis in Atd gene knockout cells and ubiquitous expression of ATD.
(A) Western blot showing expression of ATD in wild type and ATD knockout cells of HEK293T. (B) Western blot of Hsp70 for checking protein misfolding in ATD knockout and wild type of HEK293T cells. No significant change was observed in Hsp70 levels of knockout and wild type. Expression of ATD in different tissues of mice from both (C) female and (D) male, using GAPDH as a loading control. The tissue samples of mice were lysed in RIPA buffer and quantified using Bradford colorimetric assay and an equal amount of protein was loaded (15 µg per well). (E) Schematic representing the tRNA misselection by AlaRS but no mistranslation in the ATD knockout cells indicating the presence of an unknown second proofreading factor for correcting L-Ala-tRNAThr error.
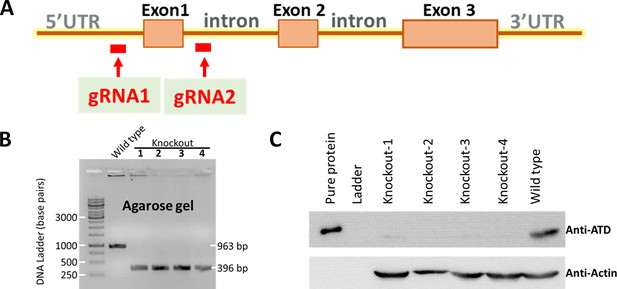
Generation of ATD knockout.
(A) Schematic showing the Atd gene with the target sites for gRNAs. Confirming the generation of ATD knockout using (B) PCR based amplification and (C) also probing of ATD using western blots.
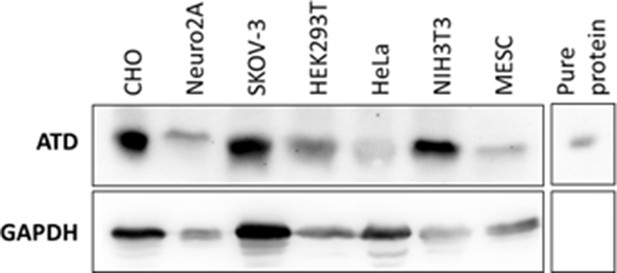
Expression of ATD in different cell lines.
All other cell lines were grown normally and the cell lysates were used for immunoblotting. CHO stands for Chinese hamster ovary cells, Neuro2A are neuroblastoma cells derived from mouse, SKOV3 is a human ovarian cancer cell line, HEK293T is a Human embryonic kidney cell line, HeLa is a cervical cancer cell line, NIH/3T3 cell lines are fibroblast cells and MESC stands for mouse embryonic stem cells (Tg2a). Purified mouse ATD protein was used as a positive control and GAPDH as loading control.
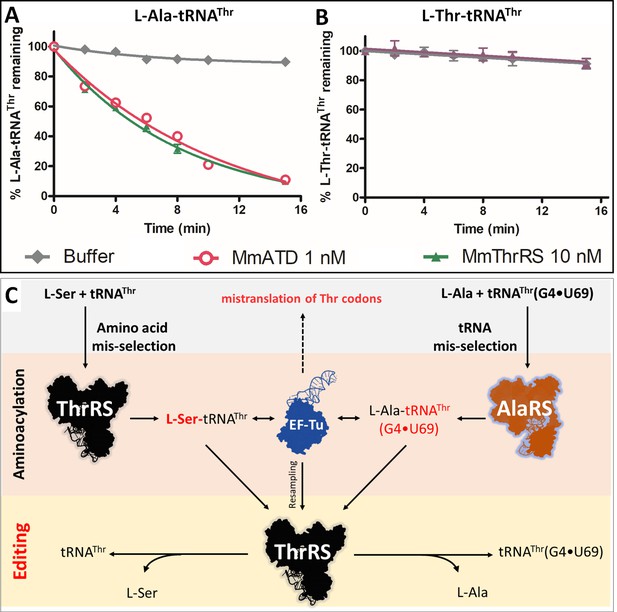
Cross-synthetase error correction by ThrRS.
(A) Deacylation of L-Ala-tRNAThr(G4•U69) by MmThrRS and MmATD, the graph clearly shows that both the enzymes are active on the substrate. (B) Deacylation of L-Thr-tRNAThr by MmThrRS, and as expected MmThrRS has no activity on the cognate substrate. Data are represented as mean ± SD of at least three independent experiments. (C) Schematic showing the cross-synthetase error correction by ThrRS. ThrRS mischarges L-Ser on tRNAThr which is proofread by the editing domain of ThrRS. The tRNA induced misselection by AlaRS generates L-Ala-tRNAThr(G4•U69), which is also proofread by ThrRS editing domain either from the free aminoacyl-tRNA pool or by resampling from EF-Tu.
-
Figure 2—source data 1
Deacylation of L-Ala/Thr-tRNAThr by MmATD and MmThrRS.
- https://cdn.elifesciences.org/articles/58118/elife-58118-fig2-data1-v2.xlsx
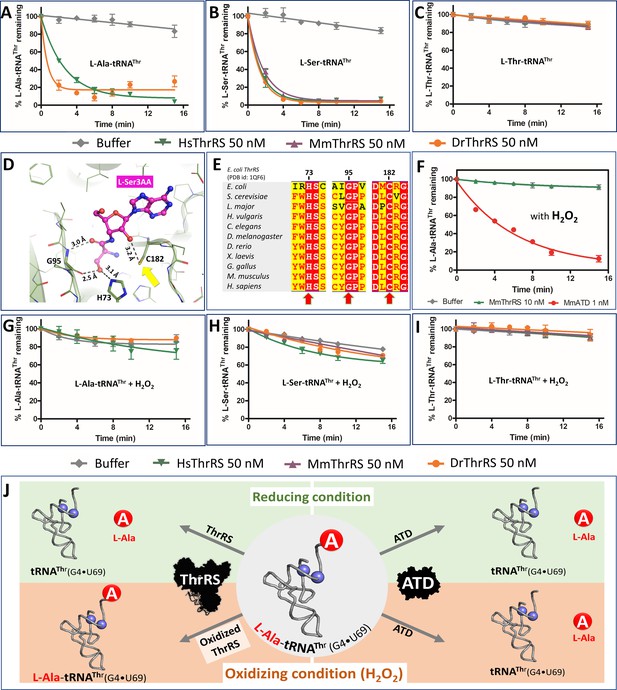
ThrRS is sensitive to oxidative stress.
(A–C) Deacylation of L-Ala/Ser/Thr-tRNAThr by ThrRS from different organisms in the absence of H2O2. (D) The active site of ThrRS editing domain in complex with L-Ser3AA (magenta sticks) showing the important interaction of the ligand with protein residues (PDB id: 1TKY). The amino acid (carbonyl oxygen and side chain hydroxyl group of L-Ser) of the incoming substrate is captured by an invariant Gly95 and His73. The ROS sensitive Cys182 (indicated using a yellow arrow) interacts with 2’OH of the terminal adenosine A76 of the aa-tRNA. (E) Structure-based sequence alignment of the ThrRS editing domain from different organisms, highlighting the invariance of residues His73, Gly95 and Cys182 throughout evolution from bacteria to humans, which are essential for the interaction with the substrate and catalysis. (F) Deacylation assay of L-Ala-tRNAThr(G4•U69) by MmThrRS and MmATD in presence of 5 mM H2O2 in the reaction mixture. The graph clearly indicates that MmThrRS is inactive in the presence of H2O2 while ATDs activity is unaffected. (G–I) Deacylation of L-Ala/Ser/Thr-tRNAThr by ThrRS from different organisms in the presence of 5 mM H2O2. The biochemical data are represented by the mean ± SD of at least three independent experiments. (J) Schematic showing the functional redundancy of ThrRS and ATD in proofreading L-Ala-tRNAThr(G4•U69) in reducing conditions, and inactivation of ThrRS during oxidative stress.
-
Figure 3—source data 1
Deacylation of L-Ala/Thr/Ser-tRNAThr by ATD and ThrRS in presence and absence of oxidative stress.
- https://cdn.elifesciences.org/articles/58118/elife-58118-fig3-data1-v2.xlsx
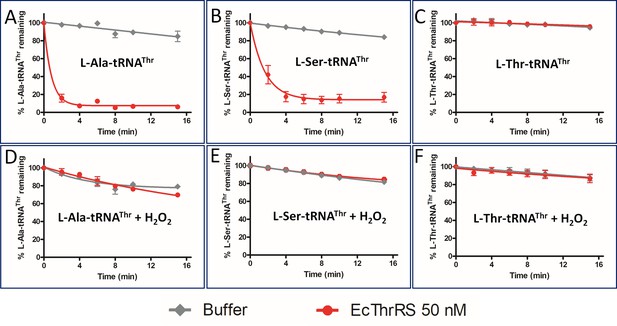
E. coli ThrRS proofreads L-Ala-tRNAThr and is sensitive to oxidative stress.
Deacylation of various substrates (L-Ala/L-Ser/L-Thr-tRNAThr) by EcThrRS in presence and absence of 5 mM H2O2.
-
Figure 3—figure supplement 1—source data 1
ThrRS editing is sensitive to oxidative stress but not ATD.
- https://cdn.elifesciences.org/articles/58118/elife-58118-fig3-figsupp1-data1-v2.xlsx
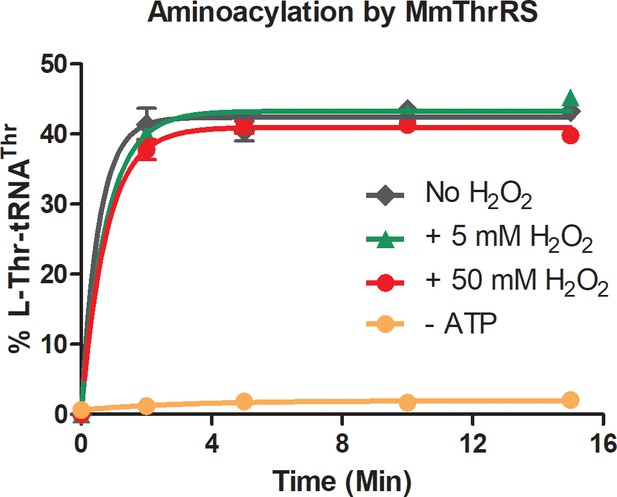
H2O2 does not affect the synthetase activity of ThrRS.
Threonylation of 2 µM tRNAThr by 400 nM of M. musculus ThrRS in presence and absence of H2O2. Reaction without ATP acts as a negative control.
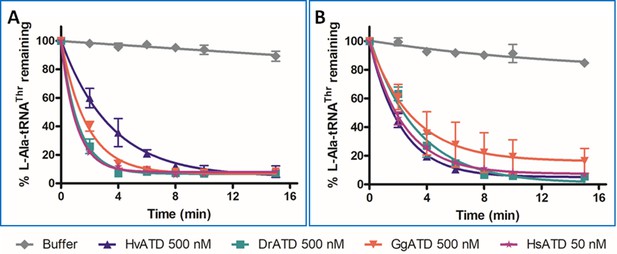
ATD is inert to oxidative stress.
Deacylation of L-Ala-tRNAThr by H. vulgaris, D. rerio, G. gallus and H. sapiens ATD A) without H2O2 and B) in presence of 5 mM H2O2. Each data point represents the mean of three independent experiments, error bars represent standard deviation.
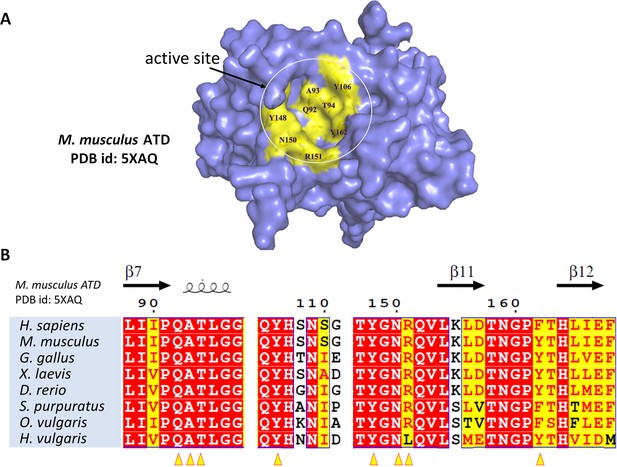
ATD does not contain any cysteine in the active site.
(A) Surface representation of MmATD with active site residues marked in yellow. (B) Structure-based sequence alignment of ATD’s across organisms with active site residues marked (with yellow arrowheads).
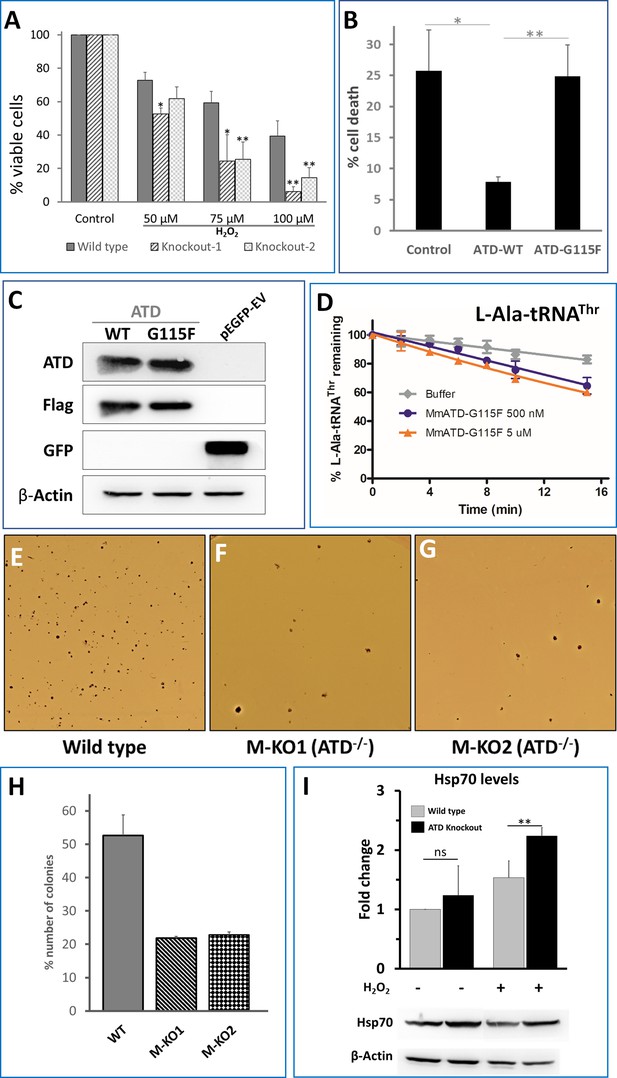
ATD is essential during oxidative stress.
(A) Cell viability assay using MTT assay. Both wild type and knockout cells (knockout 1 and knockout 2 are two lines created using CRISPR-Cas9) are treated with varying concentrations of H2O2 followed by 24 hr of incubation and assessed for viability. The p-value is a comparison between the wild type and knockout at the same concentration of H2O2. (B) Overexpression of ATD rescues H2O2 induced cell toxicity: ATD knockout cells of HEK293T were transfected with a vector containing no insert, wild type ATD and inactive mutant of ATD, and treated with 75 µM H2O2 followed by MTT assay, all the values are normalized using H2O2-untreated cells as control. * indicates p-value<0.1 and ** indicates p-value<0.01 obtained by doing student’s t-test. (C) Immunoblotting of ATD knockout cells expressing FLAG-tagged ATD using Anti-ATD and Anti-FLAG antibody. (D) Deacylation of L-Ala-tRNAThr by G115F mutant of MmATD. Data points correspond to the mean of three independent readings and error bars represent one standard deviation. (E–G) Leishman-stained colonies of mouse ES cells after growing the wild type and ATD knockout cells in presence of 0.1X βME. (H) Graph representing the number of colonies formed by wild type E14Tg2a (mouse embryonic stem cells) and ATD knockout E14Tg2a cells (M-KO represents ATD knockout of MES cells, 1 and 2 refer to the clone number) formed when grown in media containing 0.1X βME compared to that of 1X βME (100 µM). (I) Probing for the markers of protein mistranslation, Hsp70, after exposing the wild type and ATD knockout cells to oxidative stress (75 µM of H2O2) for 6 hr. (p-value for untreated wild type and knockout is insignificant (ns) and the p-value between H2O2-treated wild type and control is 0.023, denoted by ** obtained by doing student’s t-test).
-
Figure 4—source data 1
Deacylation of L-Ala-tRNAThr by G115F mutant of ATD.
- https://cdn.elifesciences.org/articles/58118/elife-58118-fig4-data1-v2.xlsx
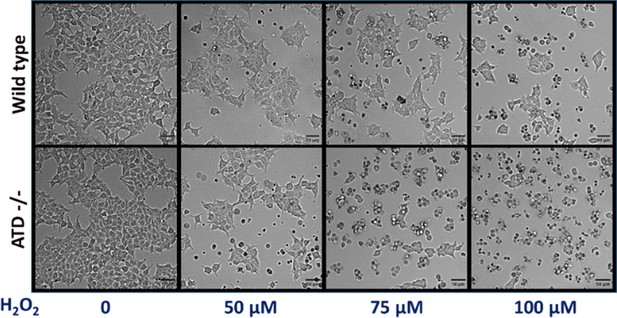
ATD knockout cells are sensitive to oxidative stress.
Microscopic images showing the higher cell death in ATD knockout cells compared to wild type cells when treated with different concentrations of H2O2.
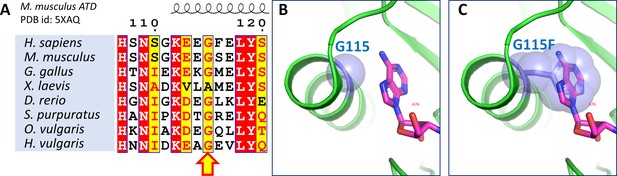
G115F mutant of ATD is inactive.
(A) Structure-based sequence alignment of ATDs from different organisms showing the conserved Gly115 in the adenine binding pocket of ATD. Adenine binding pocket of ATD (adenine modeled by overlapping MmATD (PDB id: 5XAQ) and ligand bound PfDTD (PDB id: 4NBI)) showing (B) G115 in and (C) G115F in spheres, which clearly depicts the steric exclusion of A76 from the ATDs active site.
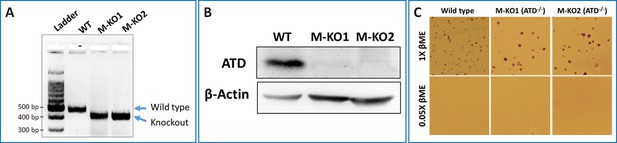
ATD knockout of mouse embryonic stem cells.
(A) Agarose gel image showing the deletion of 58 base pairs (wild type amplicon size is 473 bp and knockout amplicon size is 415 bp) in the genomic copy of ATD gene in mouse embryonic stem cell knockout (M-KO, 1 and 2 represent two independent cell lines) compared to the wild type Tg2a cells. (B) Western blot analysis of ATD expression in knockout and wild type Tg2A cells. (C) Leishman-stained colonies of MES cells wild type and ATD knockout in presence of 1X (100 µM) and 0.05X βME (5 µM) in the growth medium.
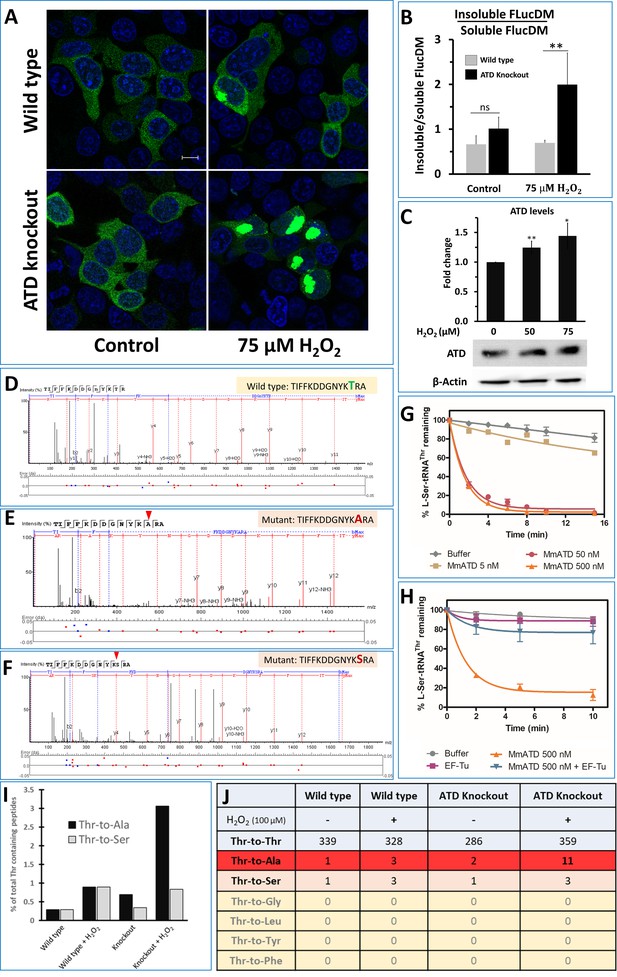
ATD avoids ROS-induced mistranslation.
(A) Microscopic images showing the aggregation of FlucDM-EGFP in the presence and absence of oxidative stress, in wild type and ATD knockout cells. The blue color corresponds to the DAPI staining of the nucleus while green corresponds to the GFP speckles formed due to the aggregation of FlucDM in response to the proteome stress. The scale corresponds to 10 µm. (B) Western blot-based quantification of soluble and insoluble (aggregates) fraction of FlucDM-EGFP using anti-GFP antibody. There was no significant difference between untreated wild type and knockout cells, while the treated cells had a significant difference and has a p-value of 0.032 obtained by doing student’s t-test indicated by **. (C) Overexpression of ATD in response to oxidative stress. Western blot of wild type HEK293T cells lysates after treating the cells with H2O2 for 2 hr and probed for expression of ATD using specific antibody. There was significant difference in ATD levels between treated and untreated cells, the * indicates a p-value of 0.023 and ** indicated p-value of 0.016 obtained by doing student’s t-test. (D–F) Tandem mass spectrometry-based analysis of the reporter protein (overexpressed GFP) isolated from H2O2-treated ATD knockout cells showing the wild type and mutant (Thr-to-Ser/Ala) spectra of the mistranslated peptides of the reporter protein. (G) Deacylation of L-Ser-tRNAThr by various concentrations of MmATD. (H) Deacylation of L-Ser-tRNAThr by MmATD in the presence and absence of elongation factor Tu. Even 500 nM of MmATD does not deacylate L-Ser-tRNAThr in presence of elongation factor. This signifies that even though ATD clears L-Ser-tRNAThr in vitro conditions, in vivo ATD does not deacylate L-Ser-tRNAThr. Biochemical data is mean of at least three independent reading and error bars represent one standard deviation. (I) Percentage of peptides with Thr-to-Ala/Ser peptides found compared to that of the wild type peptides. (J) Table listing the number of wild type and mutant peptides found in the mass spectrometry experiments and the row highlighted in red corresponds to Thr-to-Ala mutation which is highest in the H2O2-treated ATD knockout cells.
-
Figure 5—source data 1
In vivo ATD edits L-Ala-tRNAThr but not L-Ser-tRNAThr.
- https://cdn.elifesciences.org/articles/58118/elife-58118-fig5-data1-v2.xlsx
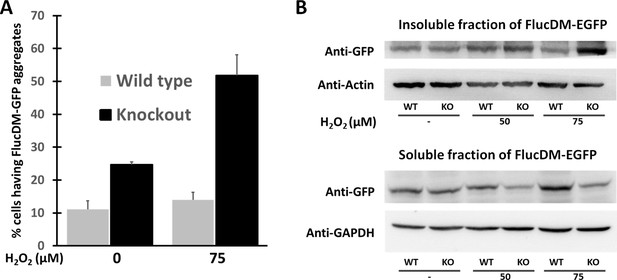
Oxidative stress induces proteome stress in ATD knockouts.
(A) The bar graph plotted represents the percentage of cells with GFP speckles formed due to aggregation of FlucDM-EGP in presence and absence of H2O2 (n = 150 cells). The number of aggregates formed increases with oxidative stress which is also seen in the form of (B) increased insoluble fraction of FlucDM-EGFP protein in the western blot.
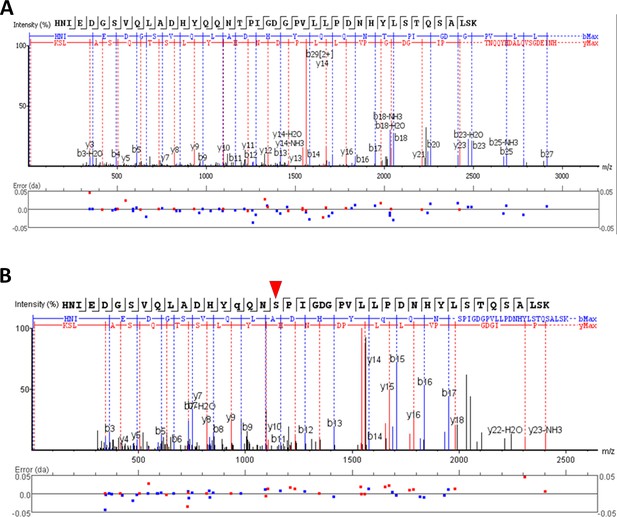
Oxidative stress leads to Thr-to-Ser mistranslation in wild type cells.
Mass spectrometry analysis of the reporter protein (GFP) isolated from H2O2-treated wild type HEK293T cells (A) wild type peptide and (B) mutant peptide showing Thr-to-Ser mistranslation.
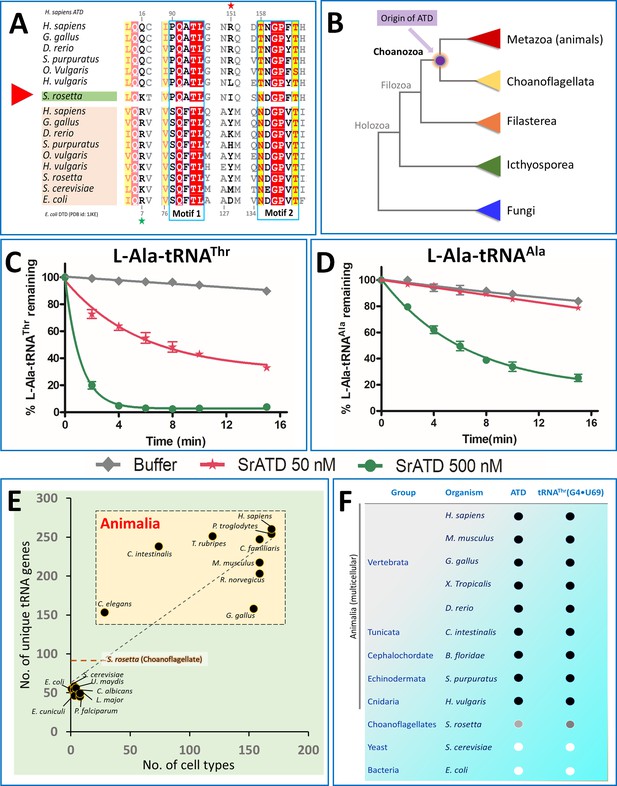
Choanoflagellates mark the origin of ATD and G4•U69 containing tRNA isodecoders.
(A) Structure-based sequence alignment of ATD and DTD sequences across organisms with Choanoflagellate ATD marked in green, which has characteristic motifs from both ATD and DTD. The sequences clearly show the mixed features of Choanoflagellate ATD in having both NXGPXT (DTD-specific) and PQATL (ATD-specific) motif along with a DTD-specific Arg/Lys (Arg7 in E. coli DTD, marked with a green star). While lacking the ATD specific Arg151 (marked with a red star). (B) The evolutionary tree showing the ATD emergence of ATD in the common ancestors of Choanoflagellates and Animals while absent in other branches of life (except few pathogens such as Leishmania, Trypanosomes, Acanthamoeba, which might have acquired from the host genome). Deacylation of (C) L-Ala-tRNAThr and (D) L-Ala-tRNAAla by various concentrations of S. rosetta ATD. Biochemical graphs are plotted using mean values and error bars represent the standard deviation. (E) Correlation between cell types and number of tRNA genes: Dot plot showing the number of unique tRNA genes and cell types in organisms (taken from Tan et al., 2009) across different life forms. The dotted orange line indicates the number of tRNAs in S. rosetta obtained using tRNA-Scan (Chan and Lowe, 2019) of the genome (Fairclough et al., 2013). (F) Co-evolution of tRNAThr(G4•U69) and ATD: The emergence of ATD is highly linked with the appearance of tRNA isodecoders containing G4•U69. ● indicates the presence of the gene, while ○ indicates absence. Choanoflagellates mark the beginning of both ATD and tRNA isodecoders with G4•U69. Intriguingly, the Choanoflagellate ATD is in a transition state and G4•U69 is present in tRNAGln, and not in tRNAThr, and hence indicated with grey circles.
-
Figure 6—source data 1
Choanoflagellate ATD edits L-Ala-tRNAThr.
- https://cdn.elifesciences.org/articles/58118/elife-58118-fig6-data1-v2.xlsx
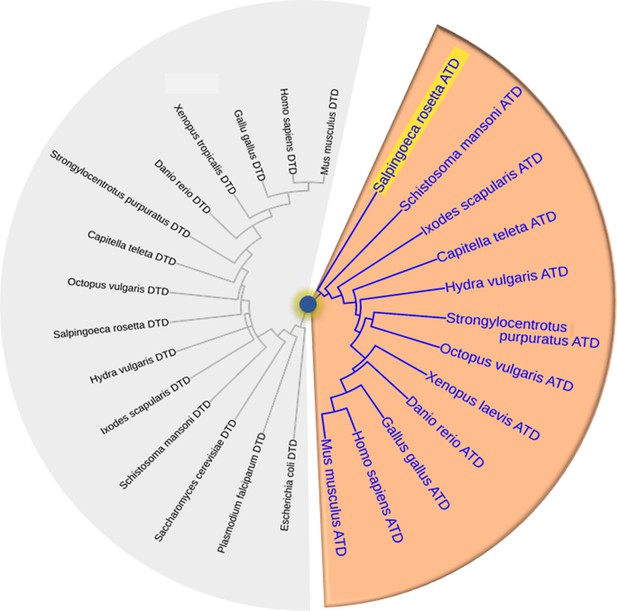
Choanoflagellates are the root of ATD origin.
Phylogeny based on sequences of DTD (with grey background) and ATD (with orange background) taken from different organisms depicting ATD is first rooted in Choanoflagellate S. rosetta which is highlighted in yellow.
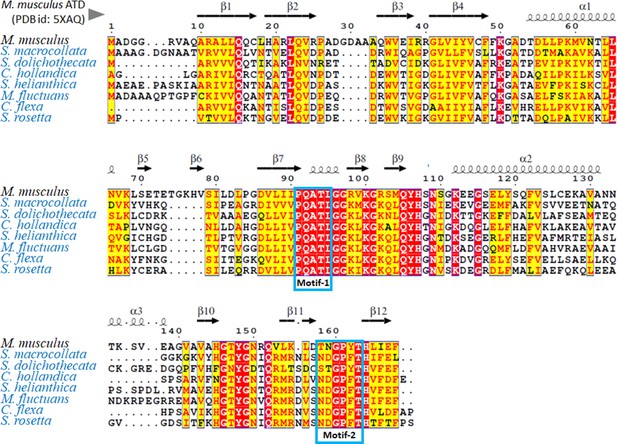
ATD in transition state.
Structure based sequence alignment of different ATD sequences obtained from transcriptome data of different Choanoflagellates (names in blue) using M. musculus ATD (PDB id: 5XAQ) as template and the motifs are highlighted using blue boxes. The ATD-specific PQATL (motif 1) is conserved across all Choanoflagellates, while the motif 2 is mostly NDGPFTH which is DTD-specific.
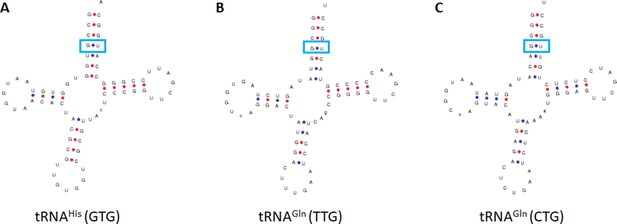
Hydra vulgaris tRNAs containing G4•U69.
The predicted tRNAs from the H. vulgaris genome containing tRNAThr isodecoders on two tRNAs with different anticodons (A) CTG and (B) AGT.
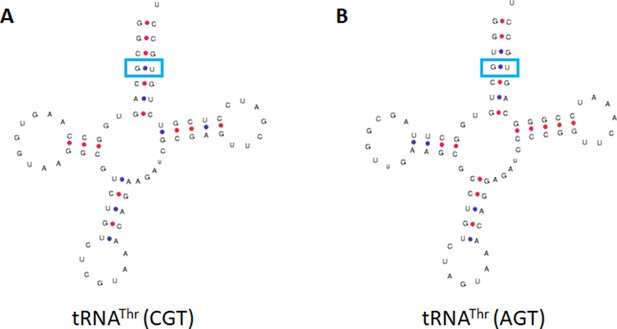
Salpingoeca rosetta tRNAs containing G4•U69.
The predicted tRNAs from the S. rosetta genome containing G4•U69 in (A) tRNAHis and (B and C) tRNAGln.
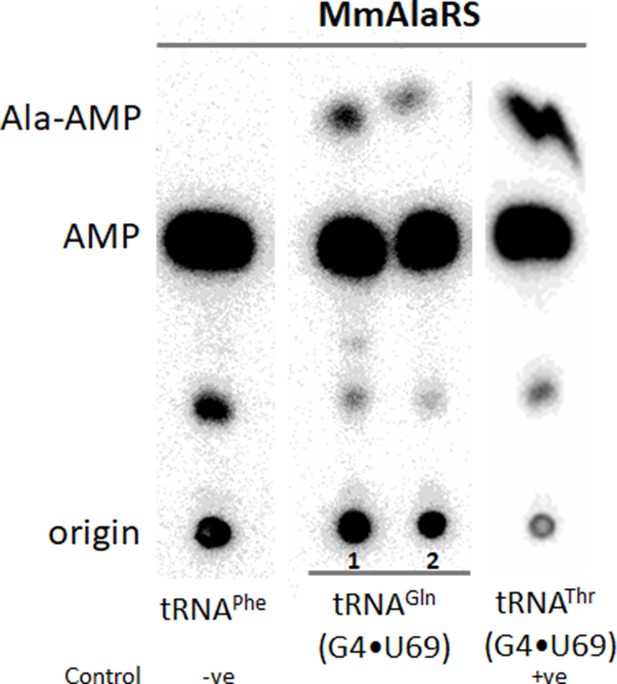
Aminoacylation of S. rosetta tRNAGln.
TLC of S1 digested aminoacylation reaction products showing aminoacylation of tRNAGln(G4•U69) by M. musculus AlaRS (1 and 2 represent the two clones of tRNAGln). tRNAPhe (does not have either G4•U69 nor G3•U70) and tRNAThr(G4•U69) are used as negative and positive control respectively.
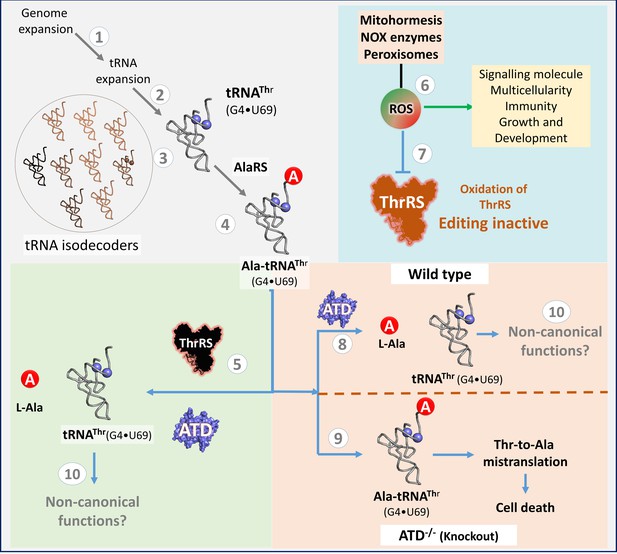
The confluence of tRNA isodecoder expansion and oxidative stress necessitates the recruitment of ATD in kingdom Animalia.
(1) Genome expansion leads to the appearance of (2,3) tRNA isodecoders which are used in different canonical and non-canonical functions, of which tRNAThr(G4•U69) is (4) mischarged by AlaRS, to give the tRNA misselection product L-Ala-tRNAThr(G4•U69). (5) in vitro these misselection products are cleared by ThrRS and ATD. 6) In multicellular animals, ROS acts as an important cellular metabolite performing various physiological functions by triggering the appropriate signaling pathway. 7) ROS generated internally in cells oxidizes the active site cysteine of ThrRS editing domain thereby affecting its proofreading activity on L-Ala-tRNAThr. 8) To avoid mistranslation ATD is recruited in Animals and Choanoflagellates as it is inert towards ROS and robustly proofreads L-Ala-tRNAThr to avoid (9) Thr-to-Ala mistranslation at the proteome level followed by cell death. 10) The tRNA isodecoders that are deacylated by ATD can either go for fresh aminoacylation or can be rerouted for other non-canonical functions.
Tables
Rate of deacylation by MmATD and MmThrRS.
Deacylation rates of L-Ala-tRNAThr (200 nM) by MmATD and MmThrRS. The kobs values are calculated by fitting the deacylation curves (Figures 2A and 3F) to the first-order exponential decay equation using GraphPad Prism.
Enzymes | 5 mM H2O2 | [Enzyme] | kobs (min−1) |
---|---|---|---|
MmATD | - | 1 nM | 0.127 ± 0.004 |
MmATD | + | 1 nM | 0.152 ± 0.005 |
MmThrRS | - | 10 nM | 0.142 ± 0.004 |
MmThrRS | + | 10 nM | No activity |
ATD is one of the key genomic innovations that arose in Choanoflagellates which is important for multicellularity.
The symbol • (dot) indicates the presence of the gene, while a white box indicates the absence of the gene in that particular organism. In the case of Porifera and Nematoda which are marked with sky blue color fill, ATD is absent and the tRNA misselection inducing species tRNAThr(G4•U69) is also absent. ATD and tRNAThr(G4•U69) genes are absent in few Annelids and Arthropods hence the boxes are lightly shaded. The data for genes other than ATD are retrieved from King et al., 2003; Nichols et al., 2012; Richter et al., 2018; Sebé-Pedrós et al., 2010.
Process | Cell cycle regulation | HIF pathway | Immune response | Quality Control | |
---|---|---|---|---|---|
Phylum | Organism | ||||
Choanoflagellate | S. rosetta | ● | ● | ● | ● |
Porifera | X. testudinaria | ● | ● | ||
Cnidaria | H. vulgaris | ● | ● | ● | ● |
Nematoda | C. elegans | ● | ● | ● | |
Arthropda | I. scapularis | ● | ● | ● | ● |
Annelida | C. teleta | ● | ● | ● | ● |
Platyhelminthus | S. mansoni | ● | ● | ● | ● |
Mollusca | O. vulgaris | ● | ● | ● | ● |
Echinodermata | S. purpuratus | ● | ● | ● | ● |
Pisces | D. rerio | ● | ● | ● | ● |
Amphibia | X. tropicalis | ● | ● | ● | ● |
Aves | G. gallus | ● | ● | ● | ● |
Reptiles | N. Naja | ● | ● | ● | ● |
Mammals | H. sapiens | ● | ● | ● | ● |
Protein/Gene | p53 | PHD | NF-κB | ATD |
Reagent type (species) or resource | Designation | Source or reference | Identifiers | Additional information |
---|---|---|---|---|
Strain, strain background (Escherichia coli) | BL21(DE3) | GE Healthcare | GE27-1542-01 | - |
Strain, strain background (Escherichia coli) | Rosetta(DE3) | Sigma-Aldrich | 70954 | - |
Cell line (Homo sapiens) | HEK293T | ATCC | CRL-3216 (RRID:CVCL_0063) | - |
Cell line (Mus musculus) | E14Tg2a | ATCC | CRL-1821 (RRID:CVCL_9108) | - |
Antibody | Anti-ATD (Rabbit and polyclonal) | Invitrogen | PA524053 (RRID:AB_2541553) | 1:2000 |
Antibody | Anti HSP-70 (Mouse and monoclonal) | Abcam | ab47454 (RRID:AB_881521) | 1:5000 |
Antibody | Anti-GFP (Rabbit and monoclonal) | CST | 2956S (RRID:AB_1196615) | 1:5000 |
Antibody | Anti-GAPDH (mouse and Monoclonal) | Abcam | ab8245 (RRID:AB_2107448) | 1:2000 |
Antibody | Anti-β-actin (Rabbit and monoclonal) | Santacruz | Sc47778 (RRID:AB_2714189) | 1:2000 |
Antibody | Anti-mouse IgG (Rabbit and monoclonal) | GE Healthcare | NA931-1ML (RRID:AB_772210) | 1:2000 |
Antibody | Anti-rabbit IgG (Mouse and monoclonal) | Santacruz | sc-2357 (RRID:AB_628497) | 1:10000 |
Recombinant DNA reagent | Escherichia coli ThrRS (Plasmid) | PMID:26113036 | UniProt id: P0A8M3 | Cloned in pET28b (N-terminal 6X-His tag) |
Recombinant DNA reagent | Danio rerio ThrRS (Plasmid) | This paper | Uniprot id: A2BIM7 | Cloned in pET28b (N-terminal 6X-His tag) |
Recombinant DNA reagent | Homo sapiens ThrRS (Plasmid) | This paper | Uniprot id: P26639 | Cloned in pET28b (N-terminal 6X-His tag) |
Recombinant DNA reagent | Mus musculus ThrRS (Plasmid) | PMID:29579307 | - | Cloned in pET28a (N-terminal 6X-His tag) |
Recombinant DNA reagent | Hydra vulgaris ATD (Plasmid) | This paper | - | Cloned in pET28b (C-terminal 6X-His tag) |
Recombinant DNA reagent | Salpingoeca rosetta ATD (Plasmid) | This paper | - | Cloned in pET28b (C-terminal 6X-His tag) |
Recombinant DNA reagent | FlucDM-EGFP (Plasmid) | PMID:21892152 | - | - |
Recombinant DNA reagent | Mus musculus ATD (Plasmid) | This paper | Uniprot id: Q8BHA3 | Cloned in pEGFP-N1 (C-terminal FLAG tag) |
Recombinant DNA reagent | Mus musculus ATD G115F (Plasmid) | This paper | - | Cloned in pEGFP-N1 (C-terminal FLAG tag) |
Commercial assay or kit | Lipofectamine 3000 | ThermoFisher Scientific | L3000015 | - |