Multi-Level Selection: The perils of cheating
Competition for resources, especially for nutrients, is pervasive in nature and can lead to both cooperation and conflict. This competition can take place at all levels of organisation – from organelles, cells and tissues to whole organisms and populations. Cheating is surprisingly common, with cheaters selfishly using common resources at the expense of others (Maynard Smith and Szathmary, 1997).
Changes in the nutritional environment often lead to competition for resources that can have a dramatic impact on the behaviour and dynamics of different groups (Pereda et al., 2017; Requejo and Camacho, 2011). This raises an important question: how are cooperation and cheating influenced by nutrition? Now, in eLife, Bryan Gitschlag, Ann Tate and Maulik Patel of Vanderbilt University report the results of experiments on the mitochondrial DNA of the nematode Caenorhabditis elegans that shed light on this question (Gitschlag et al., 2020).
Mitochondria are organelles that have their own DNA (because, it is thought, they were originally prokaryotic cells that became part of eukaryotic cells as a result of symbiosis). Indeed, mitochondria co-operate with their host cell by providing energy in exchange for a supply of molecular building blocks that are necessary to maintain and replicate mitochondrial DNA. However, a cell can contain different variants of mitochondrial DNA (mtDNA), which creates an environment where distinct mtDNA genomes have to compete to replicate first. Indeed, previous work has shown that cells can contain ‘cheater mitochondria’ that promote their own replication, even if this comes at the expense of the overall fitness of the organism (Klucnika and Ma, 2019).
Gitschlag et al. used genetically modified worms that harboured both wildtype and cheater mtDNA to confirm that the latter is able to replicate more successfully than wildtype mtDNA. Consequently, the offspring contained a higher proportion of cheater mtDNA than their parents (Figure 1). However, cheater mitochondria are not necessarily as efficient as wildtype mitochondria, and Gitschlag et al. found that worms with high levels of cheater mtDNA had decreased levels of mitochondrial function and fertility. So, while cheater DNA is better at invading the next generation, it does so at the expense of the whole organism, and across many generations, cheater mtDNA loses out to wildtype mtDNA at the population level (Figure 1).
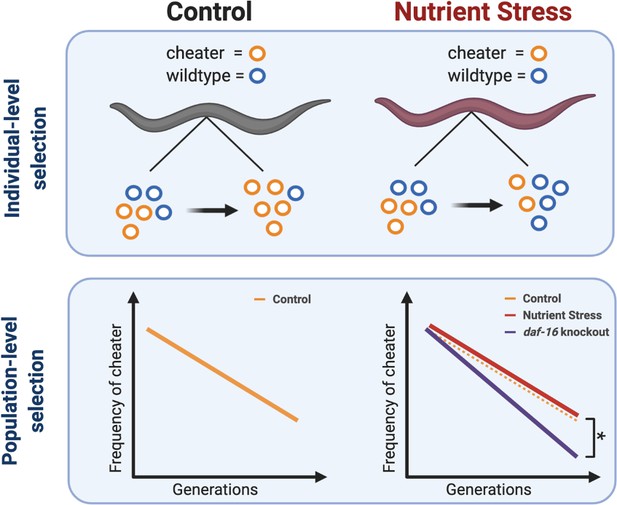
Multilevel selection of cheater mitochondria.
Cheater mitochondrial DNA (mtDNA) have a selective advantage that allows it to replicate faster than wildtype mtDNA in individual worms (top left), but this reduces fitness at the population level in successive generations, so the frequency of cheaters decreases with time (bottom left). A lack of nutrients reduces the selective advantage of cheater mtDNA within individual worms (top right), but this has little impact at the population level (bottom right; red and orange lines). However, knocking out a gene called daf-16/foxo leads to a greater reduction in the frequency of cheaters over time (bottom left; blue line) under conditions of nutrient stress.
To better understand how nutrition – and more specifically nutrient signalling – affected the worms, Gitschlag et al. applied two different strategies: restricting the supply of food to the worms and using genetic techniques to knock out a key nutrient-sensing gene (daf-16/foxo) in some worms. First, the researchers kept one group of worms on a nutrient-poor diet and a control group on a nutrient-rich diet. They found that worms raised on a restricted diet harboured less cheater mtDNA than those raised on a nutrient-rich diet. Second, without daf-16/foxo, the selective advantage of cheater mitochondria was also eliminated, and the worms had less cheater mtDNA. This suggests that cheater mtDNA depends on nutrient-sensing genes to proliferate.
Nutrient signalling also affected DNA dynamics at the population level. To study the impact of nutrient deprivation on cheater mtDNA over generations, wildtype and genetically modified worms were kept together in either nutrient-rich or nutrient-poor environments. In the nutrient-poor environments, the proportion of worms carrying cheater mtDNA decreased over generations, albeit at the same rate as in the control group, when daf-16/foxo was present. On the other hand, worms without daf-16/foxo suffered greatly when raised in nutrient-deprived environments, confirming the importance of this gene to cheater mitochondria.
Taken together, these results show that the ability to survive in stressful environments can foster tolerance to cheating, inadvertently prolonging the persistence of cheater genotypes. This suggests that across populations, the genetic response to lack of food can be exploited to partially shield cheater mitochondria from natural selection at the organismal level, which may be useful for understanding diseases associated with mitochondrial dysfunction.
References
-
Emergence and evolution of cooperation under resource pressureScientific Reports 7:45574.https://doi.org/10.1038/srep45574
-
Evolution of cooperation mediated by limiting resources: connecting resource based models and evolutionary game theoryJournal of Theoretical Biology 272:35–41.https://doi.org/10.1016/j.jtbi.2010.12.005
Article and author information
Author details
Publication history
Copyright
© 2020, Camus
This article is distributed under the terms of the Creative Commons Attribution License, which permits unrestricted use and redistribution provided that the original author and source are credited.
Metrics
-
- 1,321
- views
-
- 86
- downloads
-
- 1
- citations
Views, downloads and citations are aggregated across all versions of this paper published by eLife.
Download links
Downloads (link to download the article as PDF)
Open citations (links to open the citations from this article in various online reference manager services)
Cite this article (links to download the citations from this article in formats compatible with various reference manager tools)
Further reading
-
- Evolutionary Biology
The majority of highly polymorphic genes are related to immune functions and with over 100 alleles within a population, genes of the major histocompatibility complex (MHC) are the most polymorphic loci in vertebrates. How such extraordinary polymorphism arose and is maintained is controversial. One possibility is heterozygote advantage (HA), which can in principle maintain any number of alleles, but biologically explicit models based on this mechanism have so far failed to reliably predict the coexistence of significantly more than 10 alleles. We here present an eco-evolutionary model showing that evolution can result in the emergence and maintenance of more than 100 alleles under HA if the following two assumptions are fulfilled: first, pathogens are lethal in the absence of an appropriate immune defence; second, the effect of pathogens depends on host condition, with hosts in poorer condition being affected more strongly. Thus, our results show that HA can be a more potent force in explaining the extraordinary polymorphism found at MHC loci than currently recognised.
-
- Computational and Systems Biology
- Evolutionary Biology
As pathogens spread in a population of hosts, immunity is built up, and the pool of susceptible individuals are depleted. This generates selective pressure, to which many human RNA viruses, such as influenza virus or SARS-CoV-2, respond with rapid antigenic evolution and frequent emergence of immune evasive variants. However, the host’s immune systems adapt, and older immune responses wane, such that escape variants only enjoy a growth advantage for a limited time. If variant growth dynamics and reshaping of host-immunity operate on comparable time scales, viral adaptation is determined by eco-evolutionary interactions that are not captured by models of rapid evolution in a fixed environment. Here, we use a Susceptible/Infected model to describe the interaction between an evolving viral population in a dynamic but immunologically diverse host population. We show that depending on strain cross-immunity, heterogeneity of the host population, and durability of immune responses, escape variants initially grow exponentially, but lose their growth advantage before reaching high frequencies. Their subsequent dynamics follows an anomalous random walk determined by future escape variants and results in variant trajectories that are unpredictable. This model can explain the apparent contradiction between the clearly adaptive nature of antigenic evolution and the quasi-neutral dynamics of high-frequency variants observed for influenza viruses.