Nanoscale Signaling: Messages across time and space
Homeostasis – the ability of an organism to maintain a stable internal environment despite changes in the outside world – is essential for cells, tissues and organs to work properly. Regulating body temperature, maintaining blood pressure or monitoring blood sugar levels are all different forms of homeostasis, and they depend on specialized sensory cells. These cells monitor the environment and the body’s condition and, if necessary, chime in to keep the body functioning properly.
Sensory cells are, in turn, tightly regulated by so-called second messengers – small intracellular signaling molecules that control many different pathways within a cell. The β-cells in the pancreas, for example, are responsible for sensing elevated blood sugar levels and for releasing insulin. This hormone regulates how the body uses and stores glucose and fat. If excessive glucose concentrations are detected in the blood, second messenger molecules in the β-cells, such as calcium ions and cyclic adenosine monophosphate (cAMP), are modulated (Komatsu et al., 2013; Rorsman and Ashcroft, 2018). Temporal changes in the concentration of these two molecules cause the secretory granules (compartments within the β-cells that store insulin) to fuse with the plasma membrane, thereby releasing insulin into the blood stream (Rorsman and Ashcroft, 2018; Figure 1).
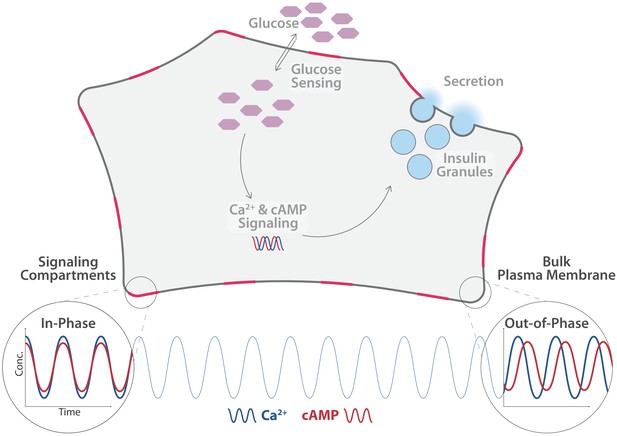
Distinct pools of cyclic adenosine monophosphate (cAMP) exist within individual β-cells.
cAMP and calcium ions (Ca2+) are important second messengers in cells. In the β-cells of the pancreas, they regulate the release of insulin (light blue) into the blood stream, . Tenner et al. found that the level of Ca2+ (blue line) oscillates uniformly across the cell. The level of cAMP (red line) in the cytosol oscillates in phase with the level of Ca2+, as does the level in free pools of cAMP in the plasma membrane (bottom right). However, the level in spatially constrained pools of cAMP in the plasma membrane (shown here in pink) oscillates out-of-phase with Ca2+ and cAMP in the cytosol in the plasmaand elswhere in the membrane.
In β-cells, the concentrations of calcium ions and cAMP oscillate to integrate external and internal signaling cues and encode them into parameters such as amplitude and frequency (Parekh, 2011; Schöfl et al., 1994). Depending on these parameters, different processes are elicited by the activity of second messengers. Previous research has shown that second messengers are not only controlled at various times, they are also spatially divided within the cells. So far, however, it remains unclear how the spatial organization of these highly diffusible molecules is achieved. Now, in eLife, Jin Zhang and colleagues from the University of California, San Diego – including Brian Tenner as first author – report new insights into second messenger oscillations (Tenner et al., 2020).
Tenner et al. used targeted biosensors to measure cAMP levels in various areas within β-cells. They identified different, spatially separated pools of cAMP, which were set apart by different oscillation patterns and concentrations of cAMP. More specifically, cAMP pools situated near specific protein complexes in the plasma membrane oscillate out-of-phase with cAMP pools located freely in the plasma membrane or in the cytosol. In comparison, calcium ion levels oscillated uniformly in the entire cell.
Using super resolution microscopy, the researchers found that the enzymes that produce and degrade cAMP play an important role in the oscillation process. The enzyme that synthesizes cAMP forms clusters in the plasma membrane, while the enzyme that degrades cAMP is dispersed in the cytosol. Based on these observations, Tenner et al. compiled a mathematical model, which confirmed that the different distributions of the two enzymes enables cAMP to form compartmentalized pools close to the plasma membrane clusters, where it is produced.
An enzyme known as protein kinase A further processes the signals encoded in the second messenger concentrations by helping calcium ions to enter the cell, thereby affecting calcium ion concentrations in the entire cell. Tenner et al. demonstrated that this effect of protein kinase A is dependent on the clustering of enzymes that produce cAMP: when these clusters were disrupted, the oscillations of calcium ions in the cytosol were less sustained and asynchronous. This suggests that modulating the oscillations of second messengers in distinct signaling compartments can affect communication in the entire cell.
While this work adds important new insights into oscillatory second messenger networks, it is based on observations made using an ammonium salt to stimulate a signaling responses. This pharmacological treatment might induce responses inside the cell that could differ from those observed after a physiological stimulation. In the future, it will be important to examine how the compartments of cAMP react to relevant physiological cues such as elevated blood sugar or blood fat levels. It will also be crucial to investigate whether the observed effects on calcium ions in the cell ultimately influence the production of insulin.
Nevertheless, Tenner et al. describe a new mechanism that may change how we think about cell signaling. Oscillatory second messenger networks integrate external and internal signals and are therefore crucial for cellular information processing. Amplitude and frequency of second messenger oscillations have long been established as parameters relevant for encoding informationIt is well known that the amplitude and frequency of these oscillations can encode information (Parekh, 2011; Schöfl et al., 1994). The findings of Tenner et al., however, suggest that the relative oscillatory phase of spatially discrete cAMP pools may constitute an additional mode for encoding information. In such a conceptual framework, a third mode of information processing would significantly increase the cell’s capability to integrate information and respond to changes in its environment (Waltermann and Klipp, 2011).
References
-
Glucose-stimulated insulin secretion: a newer perspectiveJournal of Diabetes Investigation 4:511–516.https://doi.org/10.1111/jdi.12094
-
Decoding cytosolic Ca2+ oscillationsTrends in Biochemical Sciences 36:78–87.https://doi.org/10.1016/j.tibs.2010.07.013
-
Pancreatic β-Cell electrical activity and insulin secretion: of mice and menPhysiological Reviews 98:117–214.https://doi.org/10.1152/physrev.00008.2017
-
Mechanisms of cellular information processingTrends in Endocrinology & Metabolism 5:53–59.https://doi.org/10.1016/1043-2760(94)90002-7
-
Information theory based approaches to cellular signalingBiochimica Et Biophysica Acta (BBA) - General Subjects 1810:924–932.https://doi.org/10.1016/j.bbagen.2011.07.009
Article and author information
Author details
Publication history
Copyright
© 2020, Kuhn and Nadler
This article is distributed under the terms of the Creative Commons Attribution License, which permits unrestricted use and redistribution provided that the original author and source are credited.
Metrics
-
- 1,119
- views
-
- 100
- downloads
-
- 1
- citations
Views, downloads and citations are aggregated across all versions of this paper published by eLife.
Download links
Downloads (link to download the article as PDF)
Open citations (links to open the citations from this article in various online reference manager services)
Cite this article (links to download the citations from this article in formats compatible with various reference manager tools)
Further reading
-
- Biochemistry and Chemical Biology
- Microbiology and Infectious Disease
Malaria parasites have evolved unusual metabolic adaptations that specialize them for growth within heme-rich human erythrocytes. During blood-stage infection, Plasmodium falciparum parasites internalize and digest abundant host hemoglobin within the digestive vacuole. This massive catabolic process generates copious free heme, most of which is biomineralized into inert hemozoin. Parasites also express a divergent heme oxygenase (HO)-like protein (PfHO) that lacks key active-site residues and has lost canonical HO activity. The cellular role of this unusual protein that underpins its retention by parasites has been unknown. To unravel PfHO function, we first determined a 2.8 Å-resolution X-ray structure that revealed a highly α-helical fold indicative of distant HO homology. Localization studies unveiled PfHO targeting to the apicoplast organelle, where it is imported and undergoes N-terminal processing but retains most of the electropositive transit peptide. We observed that conditional knockdown of PfHO was lethal to parasites, which died from defective apicoplast biogenesis and impaired isoprenoid-precursor synthesis. Complementation and molecular-interaction studies revealed an essential role for the electropositive N-terminus of PfHO, which selectively associates with the apicoplast genome and enzymes involved in nucleic acid metabolism and gene expression. PfHO knockdown resulted in a specific deficiency in levels of apicoplast-encoded RNA but not DNA. These studies reveal an essential function for PfHO in apicoplast maintenance and suggest that Plasmodium repurposed the conserved HO scaffold from its canonical heme-degrading function in the ancestral chloroplast to fulfill a critical adaptive role in organelle gene expression.
-
- Biochemistry and Chemical Biology
- Cell Biology
Activation of the Wnt/β-catenin pathway crucially depends on the polymerization of dishevelled 2 (DVL2) into biomolecular condensates. However, given the low affinity of known DVL2 self-interaction sites and its low cellular concentration, it is unclear how polymers can form. Here, we detect oligomeric DVL2 complexes at endogenous protein levels in human cell lines, using a biochemical ultracentrifugation assay. We identify a low-complexity region (LCR4) in the C-terminus whose deletion and fusion decreased and increased the complexes, respectively. Notably, LCR4-induced complexes correlated with the formation of microscopically visible multimeric condensates. Adjacent to LCR4, we mapped a conserved domain (CD2) promoting condensates only. Molecularly, LCR4 and CD2 mediated DVL2 self-interaction via aggregating residues and phenylalanine stickers, respectively. Point mutations inactivating these interaction sites impaired Wnt pathway activation by DVL2. Our study discovers DVL2 complexes with functional importance for Wnt/β-catenin signaling. Moreover, we provide evidence that DVL2 condensates form in two steps by pre-oligomerization via high-affinity interaction sites, such as LCR4, and subsequent condensation via low-affinity interaction sites, such as CD2.