Viruses: Neutralizing SARS-CoV-2
November brought a weary world ample reasons for celebration, even as COVID-19 cases surged anew. Among the welcome tidings were reports that mRNA-based vaccines directed at the SARS-CoV-2 spike protein may have efficacies as high as 95%, including in the elderly and other important sub-populations (Jackson et al., 2020; Sahin et al., 2020; Walsh et al., 2020; Widge et al., 2020). In addition, there are early though less definitive suggestions that monoclonal antibodies – antibodies of single specificity generated by cloning and immortalizing a plasma B cell – that target the spike protein may be therapeutic if given early (Chen et al., 2020; Rogers et al., 2020), which is a welcome counterpoint to the generally disappointing results with convalescent plasma (that is, plasma from patients who have recovered from COVID-19; Simonovich et al., 2020). Much now depends upon understanding the human neutralizing antibody response to SARS-CoV-2.
One shortcoming of convalescent plasma is that the levels of neutralizing antibodies are extremely variable, and frequently very low (Muecksch et al., 2020), with higher levels of both immunoglobulin G and immunoglobulin A correlating with more severe disease (Cervia et al., 2020). Levels also decline rapidly, by more than 50% in the first three months (Muecksch et al., 2020; Seow et al., 2020). On the other hand, monoclonals with potent neutralization capacity have been consistently obtainable from recovered COVID-19 patients and the relatively low levels of somatic hypermutation – the process by which B cells optimize antibody affinity – observed in these antibodies suggests that they might be readily elicited with the right vaccine (Robbiani et al., 2020; Yuan et al., 2020). However, it is important to understand the probability that SARS-CoV-2 may evolve to escape neutralizing antibodies, whether they are natural, vaccine-induced, or administered monoclonals.
Now, in eLife, Theodora Hatziioannou, Paul Bieniasz and co-workers – including Yiska Weisblum and Fabian Schmidt, both of Rockefeller University, as joint first authors – report data that are timely and important in this context (Weisblum et al., 2020). The researchers performed experiments in which human cells were infected, in the presence of antibodies, with a hybrid virus that mimics SARS-CoV-2. The only virus particles that could survive to propagate onward were those that had mutated in a way that allowed them to escape the antibodies. Specifically, the envelope glycoprotein of an innocuous rabies family virus was substituted with the SARS-CoV-2 spike protein (Figure 1). The antibody neutralization sensitivity of this chimeric virus tracks remarkably close to that of SARS-CoV-2, and it also provides a number of additional advantages: it enables high-throughput analyses without requiring high levels of biosecurity; it can be monitored by GFP fluorescence; and it enables the rapid selection of escape mutants because the virus propagates to high titers and – unlike a coronavirus – does not proofread mistakes made during genome copying.
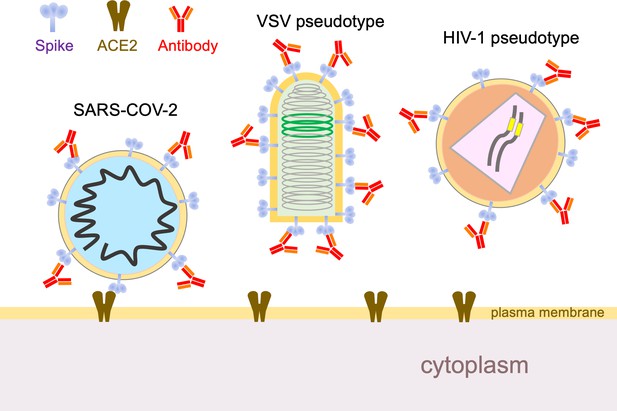
Using hybrid viruses to study SARS-CoV-2 escape from neutralizing antibodies.
The surface of the SARS-CoV-2 virion (left) contains spike proteins (pale blue) that bind to ACE2 receptors (brown), which leads to membrane fusion and entry into the cell. Neutralizing antibodies (red) can stop this happening by binding to the spike proteins, so viruses undergo reciprocal evolution to escape such antibodies. To better understand how viruses evolve to become resistant to different kinds of antibodies, Weisblum et al. developed two hybrid viruses that could be studied in the laboratory. The first was a hybrid rabies family virus (VSV, middle) that carries the SARS-CoV-2 spike protein rather than the normal envelope protein in its outer lipid envelope. This hybrid is replication-competent, carries a GFP transgene (green), and can be used for experiments in which it undergoes serial passage and selection in the presence of convalescent plasma or monoclonal antibodies. The second hybrid was an HIV-1 vector pseudotyped with the spike protein. This hybrid is replication-defective, carries a luciferase transgene (yellow), and completes a single cycle of infection. VSV: vesicular stomatitis virus.
In the presence of potent monoclonal antibodies that target the receptor binding domain of the spike protein, and some but not all convalescent plasmas, the researchers found that it took only two or three passages to select for specific resistance. (An excellent physical feel for these experiments can be had by looking at figure 1B in Weisblum et al., 2020 at higher magnification). When the escaped viruses were sequenced, mutations in the receptor binding domain – and some outside it as well – were identified. None of these mutations impaired replicative fitness in cultured cells in a discernible way.
Notably, mutations that potently blocked a given monoclonal antibody conferred little or no resistance to neutralization by plasma from the same individual (or others). Conversely, plasma from a given individual did not select for resistance to monoclonal antibodies derived from that individual. Finally, there was no overlap in the resistance selected by convalescent plasma from different individuals, suggesting that humoral immune responses are significantly heterogeneous between individuals. However, Weisblum et al. point out that they mostly tested immunoglobulin G in their experiments, whereas immunoglobulin A predominates in lung secretions and on the surfaces of respiratory epithelia, and may be particularly beneficial in the case of SARS-CoV-2 (Wang et al., 2020).
Weisblum et al. then asked an important question: do these mutant viruses exist in real SARS-CoV-2 in the general human population? For these experiments, they used a non-replicating hybrid virus, one based on HIV-1 (Figure 1). Moreover, in addition to the escape mutants selected in their first set of experiments, they used this system to test numerous naturally occurring mutations that have been identified in or near the ACE2 binding site (http://cov-glue.cvr.gla.ac.uk/#/home). This enabled them to identify additional escape mutants. Moreover, both sets of mutants are present, albeit at very low frequencies, in naturally circulating SARS-CoV-2. Thus, they 'pre-exist' and are available for selection to prominence under specific humoral immune pressure.
This situation brings to mind a lesson well learned about RNA viruses from HIV-1, which generates exceptional diversity. Not only is resistance to antiretroviral therapy quickly induced unless combinations of drugs are used, the virus in any one patient is virtually always resistant to the antibodies present in contemporaneous plasma. The problem is less severe for coronaviruses which, alone among RNA viruses, have the ability to proofread errors made during genome copying. Even so, it is likely that all possible single amino acid variants of SARS-CoV-2 currently exist many times over in the global population and perhaps even in many infected individuals. Following the paradigm of antiretroviral therapy, we can anticipate that combinations of therapeutic monoclonal antibodies will be required to securely prevent SARS-CoV-2 resistance. Indeed, Weisblum et al. go on to show that combinations of two antibodies can block the generation of resistance in vitro.
Whether antibody escape will become clinically significant for therapeutics or vaccines is not yet clear and depends on many factors, including the frequency of reinfection – which clearly happens with the four seasonal coronaviruses – and the duration of antibody responses after natural and vaccine-induced immunity. At present, however, there is no evidence that functionally significant SARS-CoV-2 variants have emerged as a result of immune pressure.
Finally, most patients – even those with low aggregate neutralizing activity in plasma – were found to have the ability to generate potent antibodies at low levels. Moreover, the genes of the potent monoclonal antibodies identified so far differ little from the germline sequence (Robbiani et al., 2020; Yuan et al., 2020). It thus seems likely that a properly designed vaccine can induce a durable and potent neutralizing antibody response, which is likely to be more effective and longer-lasting – and much more safely acquired – than responses that follow natural infection. The early mRNA vaccine clinical trial data certainly support initial effectiveness, with duration of immunity the major outstanding question to be answered.
One of the distinctive pleasures of being a virologist is the ability to see the most important and powerful idea in biology – natural selection – happen in real time. It’s even better when the experiments yield useful insights into an urgent medical problem. In this regard, Weisblum et al. have not disappointed.
References
-
Systemic and mucosal antibody responses specific to SARS-CoV-2 during mild versus severe COVID-19Journal of Allergy and Clinical Immunology.https://doi.org/10.1016/j.jaci.2020.10.040
-
SARS-CoV-2 neutralizing antibody LY-CoV555 in outpatients with Covid-19New England Journal of Medicine.https://doi.org/10.1056/NEJMoa2029849
-
An mRNA vaccine against SARS-CoV-2 — preliminary reportNew England Journal of Medicine 383:1920–1931.https://doi.org/10.1056/NEJMoa2022483
-
Longitudinal analysis of serology and neutralizing antibody levels in COVID19 convalescentsThe Journal of Infectious Diseases jiaa659.https://doi.org/10.1093/infdis/jiaa659
-
A randomized trial of convalescent plasma in Covid-19 severe pneumoniaNew England Journal of Medicine.https://doi.org/10.1056/NEJMoa2031304
-
Safety and immunogenicity of two RNA-Based Covid-19 vaccine candidatesNew England Journal of Medicine.https://doi.org/10.1056/NEJMoa2027906
-
Enhanced SARS-CoV-2 neutralization by dimeric IgAScience Translational Medicine eabf1555.https://doi.org/10.1126/scitranslmed.abf1555
-
Durability of responses after SARS-CoV-2 mRNA-1273 vaccinationNew England Journal of Medicine.https://doi.org/10.1056/NEJMc2032195
Article and author information
Author details
Publication history
Copyright
© 2020, Poeschla
This article is distributed under the terms of the Creative Commons Attribution License, which permits unrestricted use and redistribution provided that the original author and source are credited.
Metrics
-
- 2,373
- views
-
- 272
- downloads
-
- 4
- citations
Views, downloads and citations are aggregated across all versions of this paper published by eLife.
Download links
Downloads (link to download the article as PDF)
Open citations (links to open the citations from this article in various online reference manager services)
Cite this article (links to download the citations from this article in formats compatible with various reference manager tools)
Further reading
-
- Immunology and Inflammation
We analyzed here how formin-like 1 β (FMNL1β), an actin cytoskeleton-regulatory protein, regulates microtubule-organizing center (MTOC) and multivesicular bodies (MVB) polarization and exosome secretion at an immune synapse (IS) model in a phosphorylation-dependent manner. IS formation was associated with transient recruitment of FMNL1β to the IS, which was independent of protein kinase C δ (PKCδ). Simultaneous RNA interference of all FMNL1 isoforms prevented MTOC/MVB polarization and exosome secretion, which were restored by FMNL1βWT expression. However, expression of the non-phosphorylatable mutant FMNL1βS1086A did not restore neither MTOC/MVB polarization nor exosome secretion to control levels, supporting the crucial role of S1086 phosphorylation in MTOC/MVB polarization and exosome secretion. In contrast, the phosphomimetic mutant, FMNL1βS1086D, restored MTOC/MVB polarization and exosome secretion. Conversely, FMNL1βS1086D mutant did not recover the deficient MTOC/MVB polarization occurring in PKCδ-interfered clones, indicating that S1086 FMNL1β phosphorylation alone is not sufficient for MTOC/MVB polarization and exosome secretion. FMNL1 interference inhibited the depletion of F-actin at the central region of the immune synapse (cIS), which is necessary for MTOC/MVB polarization. FMNL1βWT and FMNL1βS1086D, but not FMNL1βS1086A expression, restored F-actin depletion at the cIS. Thus, actin cytoskeleton reorganization at the IS underlies the effects of all these FMNL1β variants on polarized secretory traffic. FMNL1 was found in the IS made by primary T lymphocytes, both in T cell receptor (TCR) and chimeric antigen receptor (CAR)-evoked synapses. Taken together, these results point out a crucial role of S1086 phosphorylation in FMNL1β activation, leading to cortical actin reorganization and subsequent control of MTOC/MVB polarization and exosome secretion.
-
- Immunology and Inflammation
- Neuroscience
While modern high efficacy disease modifying therapies have revolutionized the treatment of relapsing-remitting multiple sclerosis, they are less effective at controlling progressive forms of the disease. Meningeal inflammation is a recognized risk factor for cortical gray matter pathology which can result in disabling symptoms such as cognitive impairment and depression, but the mechanisms linking meningeal inflammation and gray matter pathology remain unclear. Here, we performed magnetic resonance imaging (MRI)-guided spatial transcriptomics in a mouse model of autoimmune meningeal inflammation to characterize the transcriptional signature in areas of meningeal inflammation and the underlying brain parenchyma. We found broadly increased activity of inflammatory signaling pathways at sites of meningeal inflammation, but only a subset of these pathways active in the adjacent brain parenchyma. Subclustering of regions adjacent to meningeal inflammation revealed the subset of immune programs induced in brain parenchyma, notably complement signaling and antigen processing/presentation. Trajectory gene and gene set modeling analysis confirmed variable penetration of immune signatures originating from meningeal inflammation into the adjacent brain tissue. This work contributes a valuable data resource to the field, provides the first detailed spatial transcriptomic characterization in a model of meningeal inflammation, and highlights several candidate pathways in the pathogenesis of gray matter pathology.