Conserved visual capacity of rats under red light
Abstract
Recent studies examine the behavioral capacities of rats and mice with and without visual input, and the neuronal mechanisms underlying such capacities. These animals are assumed to be functionally blind under red light, an assumption that might originate in the fact that they are dichromats who possess ultraviolet and green cones, but not red cones. But the inability to see red as a color does not necessarily rule out form vision based on red light absorption. We measured Long-Evans rats’ capacity for visual form discrimination under red light of various wavelength bands. Upon viewing a black and white grating, they had to distinguish between two categories of orientation: horizontal and vertical. Psychometric curves plotting judged orientation versus angle demonstrate the conserved visual capacity of rats under red light. Investigations aiming to explore rodent physiological and behavioral functions in the absence of visual input should not assume red-light blindness.
Introduction
Rats, like many rodents, are largely crepuscular and, even during daylight, are usually to be found in poorly illuminated environments (Macdonald et al., 1994). Their retina is rod dominated, with cones making up as little as 1% of photoreceptors (Jacobs et al., 2001; La Vail, 1976). Rods are about 100 times more sensitive (Yau, 1994) and allow vision under low-light conditions, with a peak spectral absorption of rhodopsin in rods in rats and mice at 498 nm (Bridges, 1959; Govardovskii et al., 2000; Lyubarsky et al., 2004). Although rats are not color-blind (Jacobs et al., 2001; Lemmon and Anderson, 1979; Muenzinger and Reynolds, 1936; Munn and Collins, 1936; Walton and Bornemeier, 1938), they perform poorly in discriminating between nearby wavelengths, compared to humans (Walton, 1933). Rats’ color sensitivity is based on two sets of cones with peak sensitivities in the range of ultraviolet (UV cones; peak absorption at 358–359 nm) and green (M cones; peak absorption at 509–510 nm) (Deegan and Jacobs, 1993; Jacobs et al., 1991; Szél and Röhlich, 1992). Recently, electroretinogram (ERG) responses of the photopic spectral sensitivity curves of photoreceptors of rats and mice were measured throughout the UV–visible spectrum (300–700 nm) (Rocha et al., 2016). These measurements identified two sensitivity peaks in Wistar rats: 362 and 502 nm; no significant response to long wavelength light (above 620 nm) was detected. This study reinforced the already existing notion that red light is experienced as a total absence of usable light. In contrast, another study (Niklaus et al., 2020) showed significant scotopic and photopic ERG responses to red light even at low intensities. In the present study, we challenge the notion of form vision blindness under red light and find, contrary to expectation, good behavioral performance.
Results
Rats were required to categorize the orientation of a solid disk-like object with a circular boundary and raised parallel bars, alternately colored white and black, thus forming a square-wave grating (Figure 1A). Orientations in the range of 0°– 45° were rewarded as horizontal and orientations in the range of 45°– 90° as vertical (Figure 1B). Figure 1C illustrates the sequence of events in the behavioral task. Each trial started with the rat’s head poke, which triggered the opening of an opaque gate, followed by illumination with light sources of various wavelengths. A transparent panel in front of the object prevented the rat from generating tactile cues. After observing the object, the rat turned its head toward one spout (L or R) and licked. The boundary angle, 45°, was rewarded randomly on left or right. Illumination was by a white LED array or else by monochrome LEDs with peak intensities at 626 nm, 652 nm, 729 nm, 854 nm, and 930 nm as measured by spectrometer and verified by the manufacturer’s datasheet (Figure 1D; see Materials and methods). Half widths were 12.1–49.1 nm.
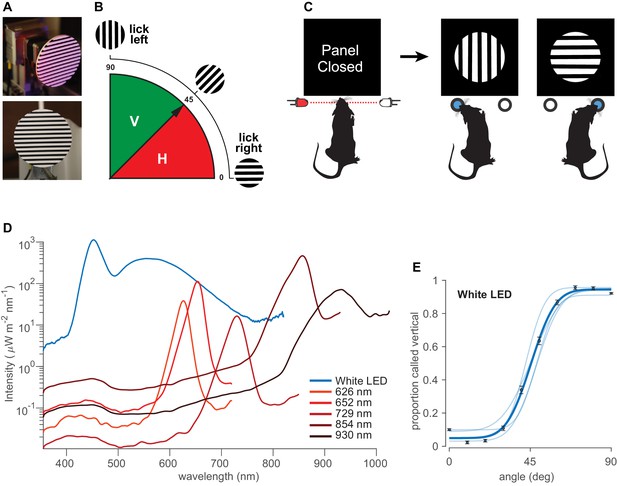
Orientation categorization task.
(A) Discriminandum viewed at an oblique angle (top) and exactly from the front (bottom), the latter approximating the perspective of the rat. (B) Schematic of the orientations of the stimuli and rule of the categorization task. 0°–45° (red) rewarded as ‘horizontal’ and 45°–90° (solid green) rewarded as ‘vertical.’. (C) Sequential steps in the behavioral task. Each trial started with a head poke that interrupted a light beam and triggered the opening of an opaque gate, followed by visual access to the object. After probing the stimulus, the rat turned its head toward one spout, in this illustration left for vertical and right for horizontal. See Figure 1—figure supplement 1 for the experimental setup. (D) Irradiance for each LED, measured by a spectrometer at the stimulus delivery area. Equivalent photon count values are shown in Figure 1—figure supplement 2. Integrating the power under the curves at wavelengths <580 nm reveals that the infrared LEDs emitted from 2 to 50 times higher intensity as compared to red LEDs, in spite of the infrared LEDs being centered at longer wavelengths (infrared 854 nm and 930 nm LEDs emitted 1.95 and 0.48 mW/cm2, respectively, while red 626, 652, and 729 nm LEDs emitted 0.12, 0.28, and 0.04 mW/cm2, respectively). See Figure 1—figure supplement 3 for normalized irradiance for each LED along with rat’s photopigment spectral sensitivity. (E) Pale curves give the performance of four rats under white light. Dark data points and curves show the average over all rats. Error bars are 95% binomial confidence intervals. See Figure 1—figure supplement 1A for the experimental setup.
© 2018, Nikbakht et al. Figure 1A-C has been reproduced from Figure 1 of Nikbakht et al., 2018, under the terms of the Creative Commons Attribution - NonCommercial - NoDerivs (CC BY-NC-ND 4.0) license.
To quantify rats’ performance, we used a cumulative Gaussian function to fit psychometric curves to the data of each rat (see Materials and methods). Figure 1E reveals that all rats (N = 4, pale curves; average curve in dark blue) performed well under white LED illumination.
Sessions with white light were interspersed with sessions illuminated by various narrow-band monochrome LEDs in the range of red, far-red, and infrared (Figure 1D). It is important to perform behavioral testing under illumination with narrow-band monochromatic light sources to eliminate the possibility of off-peak illumination through the tail of a wide power spectral distribution. Figure 2A,B shows that dark-adapted rats performed the visual categorization task under 626 nm and 652 nm LEDs (perceived as red by humans) with accuracy equivalent to that under white light, refuting the common belief of functional blindness under red light. Rats performed well even under peak 729 nm (Figure 2C), perceived as far-red by humans, though clearly diminished with respect to white. They performed poorly under infrared illumination, comprising 854 nm and 930 nm light (Figure 2D,E).
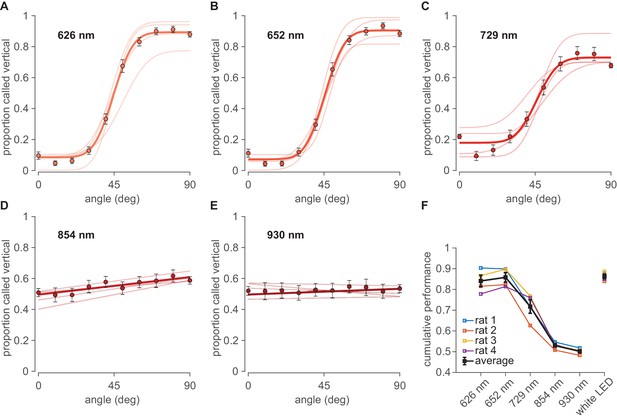
Performance under far-red and infrared illumination.
(A–E) Psychometric curves obtained under illumination with monochrome LEDs in the range of far-red to infrared with peak wavelengths at 626 nm, 652 nm, 729 nm, 854 nm and 930 nm, respectively. Pale curves depict the performance of four rats. Dark data points and lines or curves show the average over all rats. Error bars are 95% binomial confidence intervals. (F) Summary cumulative performance (proportion correct) for all four rats (colored) and the average rat (black) in each illumination condition. Data from all angles (except 45°, uninformative about discriminative capacity) are pooled. See Figure 2—figure supplement 1 for tests of significance.
The performance (N=four rats) under different illumination wavelengths is summarized by the cumulative proportion of trials categorized correctly across all orientations (Figure 2F). Average performance was 87 ± 2% correct under white light (confidence interval is SEM across rats). Average performance was 84 ± 3% under 626 nm illumination (p=0.158 compared to white light; bootstrap tests illustrated in Figure 2—figure supplement 1), 86 ± 2% under 652 nm (p=0.419 compared to white light), 72 ± 3% under 729 nm (p=0.00 compared to white light), 53 ± 2% under 854 nm (p=0.257 compared to chance), and 50 ± 1% under 930 nm average performance (p=0.316 compared to chance).
Visual acuity under the red LEDs (626 nm and 652 nm) cannot be explained by unintended ‘leakage’ toward shorter wavelengths. Figure 1D shows that the infrared LEDs (854 nm and 930 nm) emitted ~2–50 times higher intensity at shorter wavelength than did the red LEDs, yet performance under illumination by IR LEDs was at chance. Therefore, performance under red LEDs is better explained by positing that enough red light was absorbed to guide the discrimination.
Discussion
Rodent behavioral experiments done under red light are believed to place the animal in dark conditions while allowing the experimenter to observe the preparation directly or by video recording (Celikel and Sakmann, 2007; Cloke et al., 2015; Diamond et al., 1999; Englund et al., 2020; van Goethem et al., 2012; Harris and Diamond, 2000; Harris et al., 1999; Jacklin et al., 2016; Nikbakht et al., 2012; Pacchiarini et al., 2020; Reid et al., 2014; Salaberry et al., 2017; Sieben et al., 2015; Vasconcelos et al., 2011; Winters and Reid, 2010). The relevant literature includes place-cell studies of rats navigating under dim red light, taken as the absence of visual input (O'Keefe, 1976; Save et al., 2000; Zhang and Manahan-Vaughan, 2015; Zhang et al., 2014). In a developmental study of the visual cortex, mice were dark-reared but were allowed very brief daily exposure to red light (Kowalewski et al., 2021). Furthermore, red illumination is often used in reverse light cycle conditions in animal husbandry settings with the assumption that it will not affect rodent circadian rhythms (Emmer et al., 2018). In all of these cases, the animals may acquire more visual input than previously believed.
Rodents lack red cones (Deegan and Jacobs, 1993; Jacobs et al., 1991; Szél and Röhlich, 1992), but from the inability to see red as a color, it does not necessarily follow that they cannot absorb red light through their rod-dominated retina to support form vision. A recent study (Niklaus et al., 2020) examined the retinal responses mediated by rods and cones of pigmented (Brown Norway) and albino (Wistar) rats in response to monochromatic far-red light of 656 ± 10 nm in both photopic (light-adapted) and scotopic (dark-adapted) settings. Both rat strains showed significant scotopic and photopic ERG responses to red light even at low intensities. These results hinted that even far-red light may provide effective illumination for rats. However, whether the photoreceptor activation by red light can lead to functionally meaningful signals has not yet been established. Excitation of retinal receptors by red light does not, by itself, indicate behavioral availability of the signal. For instance, red light might act to entrain circadian rhythms, unconscious to the animal. The sensitivity of mice is emphasized by the finding that red illumination delivered into the brain in an optogenetic protocol evoked a behavioral artifact in mice performing a visually guided discrimination task (Danskin et al., 2015).
Although our study does not include direct measures of photoreceptor sensitivity, the results indicate that mechanisms must exist for converting miniscule quantities of receptor activation into meaningful signals, perhaps through some cross-retina population code. It is interesting to note the possibility that photoreceptors could respond to longer wavelengths through a nonlinear optical process including two-photon activation of photopigments (Palczewska et al., 2014; Vinberg et al., 2019), although the emission intensities used in our work are many orders of magnitude lower than those used in two-photon studies (Palczewska et al., 2014).
In the present work, Long-Evans rats demonstrated substantial visual form capacity under illumination by LEDs emitting long-wavelength red and far-red light – LEDs that are low cost, easy to use, and thus common in the laboratory. Object discrimination began to degrade when illumination wavelength increased from 652 to 729 nm and was almost nil at 854 nm. The performance curves suggest the optimal conditions for vision-excluded behavioral studies: illumination at 850 nm did not support visual form capacity, yet remains within the sensitivity range of inexpensive silicone detectors (CMOS and CCDs). Thus, behaviors may be documented by video while the animal performs without visual cues.
Rats and mice are the most frequently used laboratory mammals, ideal for research on spatial navigation (Frank et al., 2000; MacDonald et al., 2011; Moser et al., 2017; O’keefe and Nadel, 1978; Pastalkova et al., 2008; Wood et al., 2000) and the processing of tactile (Diamond et al., 2008; Fassihi et al., 2017; Zuo and Diamond, 2019) and olfactory (Chae et al., 2019; Koldaeva et al., 2019; Uchida and Mainen, 2003; Uchida et al., 2006) information. Neuroscientists have not traditionally attributed to rodents the wide range of visual perceptual functions characteristic of primates. However, there is growing interest in the use of rodents for the study of vision, alone or combined with other modalities (Gharaei et al., 2018; Nikbakht et al., 2018; Nikbakht Nasrabadi, 2015; Sieben et al., 2015; Zoccolan, 2015). Rats under broad-wavelength conditions spontaneously recognize an object even when views differ by angle, size, and position Zoccolan, 2015; such generalization is a hallmark of authentic visual perception and was once believed to belong only to primates. Importantly, they achieve high-level sensory-perceptual cognition through the workings of neuronal circuits that are accessible (Crochet et al., 2019; Matteucci and Zoccolan, 2020; Matteucci et al., 2019; Steinmetz et al., 2019; Tafazoli et al., 2017). The present study extends the range of visual perceptual functions for which rats can serve as models, characterizing their performance in judging object orientation and indicating the longest illumination wavelengths at which this capacity remains intact. A complete understanding of the visual processing of these animals is important not only in the design and control of the behavioral and physiological experiments but also to ensure optimal environmental lighting conditions for their well-being in laboratory settings.
Materials and methods
Experimental subject details
Request a detailed protocolFour male Long–Evans rats (Charles River Laboratories, Calco, Italy) were used. They were caged in pairs and maintained on a 12/12 hr light/dark cycle; experiments were conducted during the light phase. Upon arrival, they were 8 weeks old, weighing approximately 250 g, and typically grew to over 600 g over the course of the study. They had free access to food in the cage. To promote motivation in the behavioral task, rats were water-deprived on days of training/testing. During each session, they received 17–22 mL of pear juice diluted in water (one unit juice: four units water) as reward. After the session, they were given access to water ad libitum for 1 hr, though they were rarely thirsty and then they were placed for several hours in a large, multistory-enriched environment to interact with other rats. Animals were examined weekly by a veterinarian. Protocols conformed to international norms and were approved by the Ethics Committee of SISSA and by the Italian Health Ministry (license numbers 569/2015-PR and 570/2015-PR).
Behavioral method details
Apparatus
The main chamber of the apparatus, custom-built in opaque white Plexiglas, measured 25 × 25 × 37 (H × W × L, cm) (Figure 1—figure supplement 1). The rat started a trial by interrupting an infrared beam detected by a phototransistor (Figure 1C). Beam interruption triggered fast opening of an opaque panel (through a rotational motion of 40° in 75 ms), actuated by a stepper motor, uncovering a circular hole (diameter 5 cm) in the front wall through which the rat could extend its head to see the object. The stimulus was 3 cm behind the opaque panel (further details below), and the reward spouts were 2 cm lateral to the edge of the stimulus. A transparent panel prevented direct touch.
The apparatus was in a Faraday cage that, with the door closed, provided acoustic, visual, and electromagnetic isolation. An array of 12 infrared emitters (λ = 930 nm, OSRAM Opto Semiconductors GmbH, Germany) illuminated the stimulus port to permit the investigator to monitor behavior and to execute video recording. Such illumination did not provide visual cues for the rat (see Results). For visual testing, different light sources were used to illuminate the stimulus: a pair of six white LED arrays or various high-power monochrome LED arrays (Roithner LaserTechnik, GmbH) with peak intensities at 626 nm (LED620-66-60), 652 nm (LED660-66-60), 729 nm (LED735-66-60), 854 nm (LED850-66-60), and 930 nm (LED940-66-60) (see Figure 1D for Gaussian fits to the measured spectrum in ambient temperature). The power spectral distributions were measured with a calibrated spectrometer and the associated software (Model: FLAME-S-XR1-ES, OceanOptics, Rochester, NY) via a visible-NIR fiber (core diameter 200 µm) attached to a cosine corrector. The cosine corrector acts as an optical diffuser that couples to the optical fiber and spectrometer to collect signals from 180° field of view. The spectrometer was calibrated using a Deuterium-Halogen calibration light source unit (DH-3 plus OceanOptics, Rochester, NY). This light source provides known absolute intensity values at several wavelengths, expressed in µW/cm²/nm. The spectral intensities emitted by this source are calibrated according to the intensity standard of the National Institute of Standards and Technology (NIST). The spectrometer calibration procedure accounts for the different sensitivities of the spectrometer across different wavelengths. The spectrometer we used has a software suite that reports the values measured from the calibrated spectrometer as spectral irradiance (the power received by a surface per unit area of the measurement probe per wavelength [in units of µW/cm2/nm]).
Two infrared-sensitive video systems (Point Grey Flea, Edmund Optics, Barrington, NJ) registered the rat’s actions. The first camera, equipped with a macro lens (Fujinon TV HF25HA-1B Lens, Fujifilm, Tokyo) mounted 25 cm above the stimulus delivery area (distance with respect to the center of stimulus), monitored the rat’s interaction with the object. In some sessions, this camera was set to 250 f/s to monitor head, snout, and whisker position and movement during behavior. The second camera provided a wide-angle view (Fujinon HF9HA-1B Lens, Fujifilm, Tokyo) and monitored the entire setup, illuminated with adjustable infrared LEDs, at 30 f/s.
Reward spouts included custom-made infrared diode sensors interrupted by the tongue. Only the licking signal from the correct spout triggered the pump motor (NE-500 programmable OEM; New Era Pump Systems, mounted on a vibration-cancellation pedestal) to extrude the reward, 0.05 mL per trial of diluted pear juice. Licking marked the end of the trial, accompanied by the closure of the opaque front panel. Before the next trial began, the motor on which the stimulus was mounted rotated to generate the next orientation.
Custom-made software was developed using LabVIEW (National Instruments, Austin, TX). An AVR32 board (National Instruments) and multiple Arduino Shields (National Instruments) acquired all sensor signals and controlled the motors, LEDs, and the reward syringe pumps. All the sensors, actuators (including motors and pumps), and lights were interfaced with the computer program allowing full control over a wide range of parameters governing the flow of the training and testing. Although fully automatic, the software allowed the experimenter to modify all the parameters of the task and control the lights, sensors, and motors online as needed.
Visual stimulus presentation
Request a detailed protocolThe stimulus was a black and white square-wave grating within a circular 9.8 cm diameter circumference, built in-house by a 3D printer (3D Touch, BFB Technologies, Figure 1A). It was mounted on a stepper motor and rotated to generate the trial’s intended orientation (Figure 1B). The stimulus stepper motor was controlled through a feedback system with a digital step counter to maintain the exact desired orientation. In the study that originally explored orientation judgment (Nikbakht et al., 2018), tactile exploration of the object was allowed in some trials, but the tactile condition is not considered in the present work; only visual, touch-free data are included. Within behavioral testing sessions, each stimulus orientation was sampled from a uniform distribution in 5° steps between −45° and 135° and presented in a semi-random fashion (sampling without replacement). For analysis, we binned the angles every 10°. Visual acuity is measured in cycles per degree (cpd), an assessment of the number of lines that can be seen as distinct within a degree of the visual field:
where is the width of each line in the stimulus and is the distance from the eye. Considering the 3 cm distance behind the opaque panel (Figure 1—figure supplement 1), at the moment of panel opening, each cycle of the 14 cycles would occupy of visual angle, for a total stimulus coverage of about 117°. The spatial frequency of the gratings would be cycles per degree of visual angle. As the normal visual acuity of Long–Evans rats has been estimated as ∼1 cpd (Prusky et al., 2002), the bars would be expected to be resolvable. Rats also have a large depth of focus, from 7 cm to infinity (Powers and Green, 1978). The width of the binocular field directly in front of the rat’s nose, generally considered the animal’s binocular viewing area (Mei et al., 2012), ranges from approximately 40°–110°, depending on head pitch (Wallace et al., 2013). The 117° stimulus should thus completely cover the rat’s binocular visual field.
When all illumination sources were turned off, the ambient light magnitude was 0 cd/mm2 (Konica Minolta LS-100 luminance meter, Tokyo).
Quantification of LED emission characteristics
Request a detailed protocolFollowing the procedure of Franke et al., 2019, for each LED, we computed the following measurements, which we have included in Supplementary table 2.
Using the spectrophotometer measurements (), we can calculate the spectral power density for each LED:
The energy of a single photon at wavelength is , where is the speed of light, and is Planck's constant.
Then we computed the spectral photon count for each LED ():
Given the distance between the LEDs and the stimulus delivery area, the rat’s dilated pupil area of , the rat’s retinal surface area of , and the rat’s rod outer segment area of (Mayhew and Astle, 1997), we approximated the photon flux density (C) of an isotropic LED radiation pattern at each photoreceptor as:
Then, the photon flux per photoreceptor is:
Behavioral task and training
Request a detailed protocolDuration of training to reach stable performance was typically 4–6 weeks, with one session per day, and varied according to individual differences in rate of learning. The sequence of sessions for each rat was randomized, with each day’s session illuminated by either red, infrared, or white LEDs, with equal likelihood. The standard training was done with stimulus illumination by white LEDs. The training protocol proceeded across a sequence of stages given in Nikbakht et al., 2018. Exclusion of unintended stimulus cues (olfaction, rotating motor noise) was controlled as given in Nikbakht et al., 2018. Rats were dark-adapted in a light-free environment for 20–30 min prior to each session.
Quantification and statistical analysis
Analysis of behavioral data
Request a detailed protocolWe analyzed the behavioral data in MATLAB (MathWorks, Natick, MA) and LabVIEW. To quantify a single rat’s performance, we fit psychometric curves to its choice data. For a given orientation, we calculated the proportion of trials categorized as vertical. Ideally, rats would categorize all trials with angle greater than 45° as vertical and all trials with angle less than 45° as horizontal. For 45° trials, choices should be evenly distributed between vertical and horizontal. However, task difficulty grows in the vicinity of 45°, such that real performance is better described by a sigmoid function with an inflection point at the point of subjective equality (PSE), the orientation at which subjects report the stimulus with equal likelihood as horizontal or vertical. In unbiased rats, the PSE should be at 45°. We generated psychometric functions using a cumulative Gaussian function with the general form given in the equation below based on (Wichmann and Hill, 2001). The parameter estimation was then performed in MATLAB using maximum-likelihood estimation:
The two-parameter function, , is defined by a cumulative Gaussian distribution, as follows:
where is the stimulus orientation, is the lower bound of the function , and is the lapse rate. Often, and are considered to arise from stimulus-independent mechanisms of guessing and lapsing. is the mean of the probability distribution that determines the displacement along the abscissa of the psychometric function – a reflection of the subject’s bias – and is the standard deviation of the cumulative Gaussian distribution. determines the slope of the psychometric function, a common measure of acuity.
Where fitting with a sigmoid was not appropriate (Figure 2D,E), data were fit with the line , where is the stimulus orientation, is the slope, and is the y-intercept.
Test of significance for the fitted psychometric curves
Request a detailed protocolErrors around the performance value for each orientation and modality condition were expressed as a 95% binomial proportion confidence interval computed by approximating the distribution of errors about a binomially distributed observation, , with a normal distribution:
where is the proportion of correct trials (Bernoulli) and is the number of trials.
For statistical tests of significance, we performed a non-parametric test based on bootstrapping, as follows. We computed a distribution of the performance values from the fitted psychometric functions based on 1000 resamples of the behavioral data. We then performed pairwise comparisons between all the performance values generated via bootstrapping from fitted psychometric functions of each experimental condition, calculated the overlap between the distributions and computed the p-values.
Data availability
All data generated or analyzed during this study will be included in the manuscript and supporting files. Source code files will be provided for Figures 1 and 2 at https://github.com/nadernik/nikbakht_diamond_elife (copy archived at https://archive.softwareheritage.org/swh:1:rev:4ba735b86cbcb4bb0094d1d8531a36297561752a).
References
-
The neural bases of crossmodal object recognition in non-human primates and rodents: a reviewBehavioural Brain Research 285:118–130.https://doi.org/10.1016/j.bbr.2014.09.039
-
Neural circuits for Goal-Directed sensorimotor transformationsTrends in Neurosciences 42:66–77.https://doi.org/10.1016/j.tins.2018.08.011
-
On the identity of the cone types of the rat retinaExperimental Eye Research 56:375–377.https://doi.org/10.1006/exer.1993.1049
-
'Where' and 'what' in the whisker sensorimotor systemNature Reviews Neuroscience 9:601–612.https://doi.org/10.1038/nrn2411
-
Effects of light at night on laboratory animals and research outcomesBehavioral Neuroscience 132:302–314.https://doi.org/10.1037/bne0000252
-
Transformation of Perception from Sensory to Motor CortexCurrent Biology 27:1585–1596.https://doi.org/10.1016/j.cub.2017.05.011
-
In search of the visual pigment templateVisual Neuroscience 17:509–528.https://doi.org/10.1017/S0952523800174036
-
Cone-based vision of rats for ultraviolet and visible lightsJournal of Experimental Biology 204:2439–2446.https://doi.org/10.1242/jeb.204.14.2439
-
Survival of some photoreceptor cells in albino rats following long-term exposure to continuous lightInvestigative Ophthalmology 15:64–70.
-
Behavioral correlates of constant light-induced retinal degenerationExperimental Neurology 63:35–49.https://doi.org/10.1016/0014-4886(79)90184-5
-
The natural history of rodents: preadaptations to pestilenceRodent Pests and Their Control 1:22.
-
Nonlinear processing of shape information in rat lateral extrastriate cortexThe Journal of Neuroscience 39:1938-18–1670.https://doi.org/10.1523/JNEUROSCI.1938-18.2018
-
Spatial representation in the hippocampal formation: a historyNature Neuroscience 20:1448–1464.https://doi.org/10.1038/nn.4653
-
Color vision in white rats: I. sensitivity to redThe Pedagogical Seminary and Journal of Genetic Psychology 48:58–71.https://doi.org/10.1080/08856559.1936.10533711
-
Discrimination of red by white ratsThe Pedagogical Seminary and Journal of Genetic Psychology 48:72–87.https://doi.org/10.1080/08856559.1936.10533712
-
ConferenceVisual tactile integration in rats and underlying neuronal mechanismsBernstein Conference 2016At: Berlin.https://doi.org/10.12751/nncn.bc2016.0138
-
Place units in the Hippocampus of the freely moving ratExperimental Neurology 51:78–109.https://doi.org/10.1016/0014-4886(76)90055-8
-
Whisker-mediated texture discrimination learning in freely moving miceJournal of Experimental Psychology: Animal Learning and Cognition 46:40–46.https://doi.org/10.1037/xan0000212
-
Variation in visual acuity within pigmented, and between pigmented and albino rat strainsBehavioural Brain Research 136:339–348.https://doi.org/10.1016/S0166-4328(02)00126-2
-
The Clock Gene Rev-Erbα Regulates Methamphetamine Actions on Circadian Timekeeping in the Mouse BrainMolecular Neurobiology 54:5327–5334.https://doi.org/10.1007/s12035-016-0076-z
-
Two cone types of rat retina detected by anti-visual pigment antibodiesExperimental Eye Research 55:47–52.https://doi.org/10.1016/0014-4835(92)90090-F
-
Seeing at a glance, smelling in a whiff: rapid forms of perceptual decision makingNature Reviews Neuroscience 7:485–491.https://doi.org/10.1038/nrn1933
-
Speed and accuracy of olfactory discrimination in the ratNature Neuroscience 6:1224–1229.https://doi.org/10.1038/nn1142
-
Object recognition testing: Rodent species, strains, housing conditions, and estrous cycleBehavioural Brain Research 232:323–334.https://doi.org/10.1016/j.bbr.2012.03.023
-
Color vision and color preference in the albino rat. II. the experiments and resultsJournal of Comparative Psychology 15:373–394.https://doi.org/10.1037/h0072748
-
Further evidence of color discrimination in rodentsThe Pedagogical Seminary and Journal of Genetic Psychology 52:165–181.https://doi.org/10.1080/08856559.1938.10532519
-
The psychometric function: I. fitting, sampling, and goodness of fitPerception & Psychophysics 63:1293–1313.https://doi.org/10.3758/BF03194544
-
A Distributed Cortical Representation Underlies Crossmodal Object Recognition in RatsJournal of Neuroscience 30:6253–6261.https://doi.org/10.1523/JNEUROSCI.6073-09.2010
-
Phototransduction mechanism in retinal rods and cones the friedenwald lectureInvestigative Ophthalmology & Visual Science 35:9–32.
-
Spatial representations of place cells in darkness are supported by path integration and border informationFrontiers in Behavioral Neuroscience 8:222.https://doi.org/10.3389/fnbeh.2014.00222
-
Invariant visual object recognition and shape processing in ratsBehavioural Brain Research 285:10–33.https://doi.org/10.1016/j.bbr.2014.12.053
-
Texture Identification by Bounded Integration of Sensory Cortical SignalsCurrent Biology 29:1425–1435.https://doi.org/10.1016/j.cub.2019.03.017
Article and author information
Author details
Funding
European Research Council (294498)
- Mathew E Diamond
Human Frontier Science Program (RGP0015/2013)
- Mathew E Diamond
The funders had no role in study design, data collection and interpretation, or the decision to submit the work for publication.
Acknowledgements
We acknowledge the financial support of the Human Frontier Science Program (MED) (http://www.hfsp.org; project RGP0015/2013) and the European Research Council advanced grant CONCEPT (MED) (http://erc.europa.eu; project 294498). The Regional Laboratory for Advanced Mechatronics, LAMA FVG (http://lamafvg.it), supported the design and construction of custom instrumentation. The funders had no role in study design, data collection and analysis, decision to publish, or preparation of the manuscript. Erik Zorzin assisted in spectral measurements. We are grateful to members of the Diamond lab for fruitful comments and discussions.
Ethics
Animal experimentation: The rats were under the care of a consulting veterinarian. Study protocols conformed to international norms and were approved by the Ethics Committee of SISSA and by the Italian Health Ministry (license numbers 569/2015-PR and 570/2015-PR).
Copyright
© 2021, Nikbakht and Diamond
This article is distributed under the terms of the Creative Commons Attribution License, which permits unrestricted use and redistribution provided that the original author and source are credited.
Metrics
-
- 5,930
- views
-
- 454
- downloads
-
- 28
- citations
Views, downloads and citations are aggregated across all versions of this paper published by eLife.
Download links
Downloads (link to download the article as PDF)
Open citations (links to open the citations from this article in various online reference manager services)
Cite this article (links to download the citations from this article in formats compatible with various reference manager tools)
Further reading
-
- Neuroscience
Motivation depends on dopamine, but might be modulated by acetylcholine which influences dopamine release in the striatum, and amplifies motivation in animal studies. A corresponding effect in humans would be important clinically, since anticholinergic drugs are frequently used in Parkinson’s disease, a condition that can also disrupt motivation. Reward and dopamine make us more ready to respond, as indexed by reaction times (RT), and move faster, sometimes termed vigour. These effects may be controlled by preparatory processes that can be tracked using electroencephalography (EEG). We measured vigour in a placebo-controlled, double-blinded study of trihexyphenidyl (THP), a muscarinic antagonist, with an incentivised eye movement task and EEG. Participants responded faster and with greater vigour when incentives were high, but THP blunted these motivational effects, suggesting that muscarinic receptors facilitate invigoration by reward. Preparatory EEG build-up (contingent negative variation [CNV]) was strengthened by high incentives and by muscarinic blockade, although THP reduced the incentive effect. The amplitude of preparatory activity predicted both vigour and RT, although over distinct scalp regions; frontal activity predicted vigour, whereas a larger, earlier, central component predicted RT. The incentivisation of RT was partly mediated by the CNV, though vigour was not. Moreover, the CNV mediated the drug’s effect on dampening incentives, suggesting that muscarinic receptors underlie the motivational influence on this preparatory activity. Taken together, these findings show that a muscarinic blocker impairs motivated action in healthy people, and that medial frontal preparatory neural activity mediates this for RT.
-
- Neuroscience
Complex macro-scale patterns of brain activity that emerge during periods of wakeful rest provide insight into the organisation of neural function, how these differentiate individuals based on their traits, and the neural basis of different types of self-generated thoughts. Although brain activity during wakeful rest is valuable for understanding important features of human cognition, its unconstrained nature makes it difficult to disentangle neural features related to personality traits from those related to the thoughts occurring at rest. Our study builds on recent perspectives from work on ongoing conscious thought that highlight the interactions between three brain networks – ventral and dorsal attention networks, as well as the default mode network. We combined measures of personality with state-of-the-art indices of ongoing thoughts at rest and brain imaging analysis and explored whether this ‘tri-partite’ view can provide a framework within which to understand the contribution of states and traits to observed patterns of neural activity at rest. To capture macro-scale relationships between different brain systems, we calculated cortical gradients to describe brain organisation in a low-dimensional space. Our analysis established that for more introverted individuals, regions of the ventral attention network were functionally more aligned to regions of the somatomotor system and the default mode network. At the same time, a pattern of detailed self-generated thought was associated with a decoupling of regions of dorsal attention from regions in the default mode network. Our study, therefore, establishes that interactions between attention systems and the default mode network are important influences on ongoing thought at rest and highlights the value of integrating contemporary perspectives on conscious experience when understanding patterns of brain activity at rest.