Activity-dependent Golgi satellite formation in dendrites reshapes the neuronal surface glycoproteome
Figures
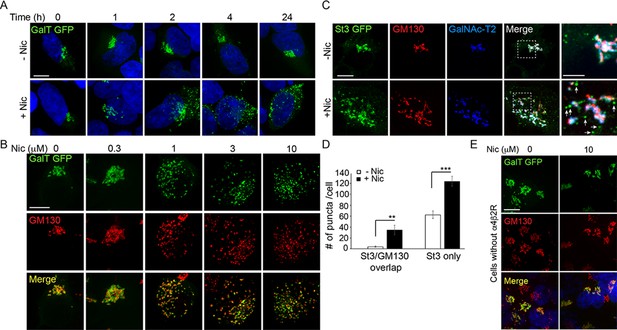
Golgi dispersal by nicotine exposure in non-excitable, α4β2R-expressing human embryonic kidney (HEK) cells.
(A) Time course of Golgi dispersal by nicotine. HEK293 cells stably expressing α4β2Rs (α4β2R cells) were transfected with GFP-tagged galactosyltransferase (GalT, green) and imaged at the indicated times after nicotine exposure (10 μM). Cells were also labeled with DAPI (blue) after fixation. Scale bar, 10 μm. (B) Dose dependence of Golgi dispersal by nicotine. Cells were fixed after 17 hr of nicotine exposure at the indicated concentrations and then stained with anti-GM130 antibody (red) and DAPI (blue) before imaging. Scale bar, 10 μm. (C) Heterogeneity of Golgi components in nicotine-dispersed Golgi fragments. α4β2R cells were transfected with GFP-GalT (blue) and St3-GFP (green) and nicotine-treated as in A prior to fixation and staining for GM130 (red). Scale bar, 10 μm. (D) Numbers of dispersed Golgi puncta per cell that contain both GM130 and St3, or only St3. Total number of puncta per cell, data are shown as mean ± SEM. For left graph, untreated cells, 3.9 ± 1.1; nicotine-treated cells, 34.6 ± 9.0 (n = 8–10 cells per group, **p < 0.05). For right graph, control cells, 62.6 ± 6.9; nicotine cells, 124.1 ± 8.9 (n = 10 cells per group, ***p < 0.00001). (E) Nicotine-treatment does not alter Golgi morphology in cells that do not express α4β2R. HEK293 cells without α4β2Rs were transfected with GalT-GFP and then treated with or without nicotine (10 μM) for 17 hr and imaged. Scale bar, 10 μm.
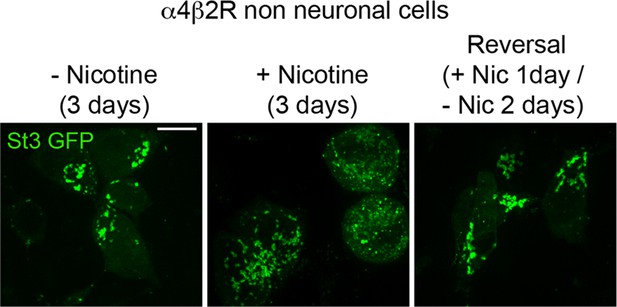
Reversal of nicotine-induced Golgi dispersal.
α4β2R cells were transfected with St3-GFP for 24 hr and treated with or without 10 μM nicotine for 1 day. To visualize reversal, cells were washed with media to remove nicotine and maintained in drug-free media for 2 days. A parallel set of nicotine-treated cells were maintained in nicotine for the duration of the experiment. Untreated cells were maintained in nicotine-free media. Representative images of the cells under the three conditions are displayed. Scale bar, 10 μm.
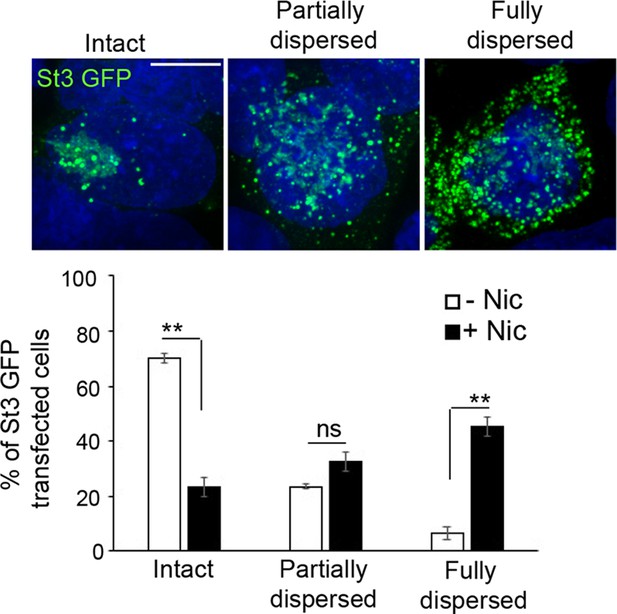
Classification and quantification of cells into three categories (intact, partially dispersed, and fully dispersed) based on Golgi integrity and morphology.
α4β2R cells were transfected with St3-GFP (green) for 24 hr and treated with or without 10 μM nicotine for 17 hr. Cells were fixed and stained with DAPI (blue). Top, representative images of cells with intact, partially dispersed and fully dispersed Golgi. Bottom, percentage of St3-GFP transfected cells with each phenotype were quantified under nicotine-treated and untreated conditions. Data represents mean ± SEM (n = 4 independent experiments; total number of St3-GFP transfected cells analyzed; control cells, 487; nicotine cells, 469; percentage of cells with intact Golgi, control cells, 69% ± 1.7%; nicotine cells, 23.3% ± 3.6%, con vs nic, **p < 0.0002; partially dispersed Golgi, control cells, 23.3% ± 1%; nicotine cells, 32.3% ± 3.4%, con vs nic, p = 0.14663, not significant; dispersed Golgi, control cells, 6.2% ± 2.2%; nicotine cells, 45.2% ± 3.2%, con vs nic, **p < 0.0002). Scale bar, 10 μm.
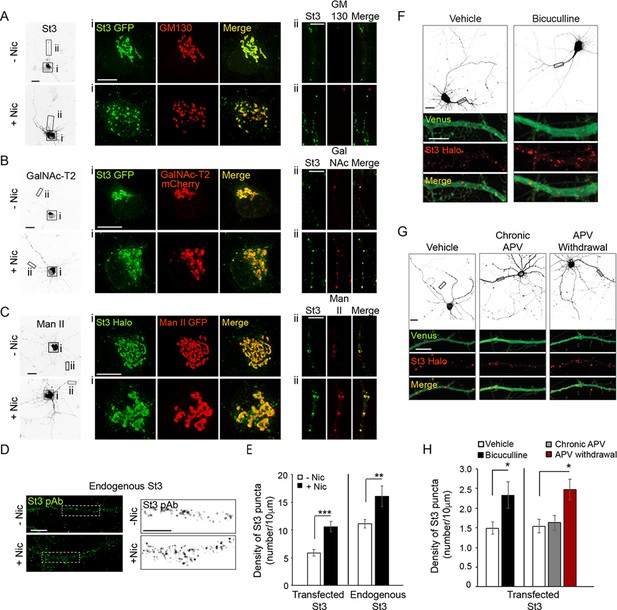
Nicotine- and activity-dependent Golgi dispersal and Golgi satellite formation in cultured neurons.
(A–E) Nicotine-dependent changes. Scale bars, 10 µm. (A) Neurons were treated with or without nicotine, and the Golgi was imaged with St3 and GM130 markers. DIV10 cortical neurons (E18 rat pups) were transfected with St3-GFP (green) and HA-tagged α4β2R subunits. Cultures were fixed and stained for GM130 (red). Low magnified images of neurons treated with (bottom, +Nic) or without (top, -Nic) 1 μM nicotine for 17 hr. (i) Images of St3- and GM130-containing Golgi in somata. (ii) Images of St3- and GM130-containing Golgi in dendrites. (B) Neurons were treated with or without nicotine, and the Golgi was imaged using St3 and GalN as markers. Cultures were transfected, nicotine-treated or untreated, and analyzed as in A, with the additional transfection of GalN-mCherry (red) replacing the GM130 marker. (i) Images of St3- and GalN-containing Golgi in the soma. (ii) Images of St3- and GalNAc-T2-containing Golgi in dendrites. (C) Neurons were treated with or without nicotine and the Golgi was imaged using St3 and Man markers. Cultures were transfected, nicotine-treated or untreated, and analyzed as in B, except with transfection of Man II GFP (Man-GFP; red) replacing GalNAc-T2-mCherry. (i) Images of St3- and Man II-containing Golgi in the somata ii. Images of St3- and Man II-containing Golgi in dendrites. (D) Images of nicotine-treated (+Nic) and untreated (-Nic) dendrites, with endogenous St3 labeled with polyclonal anti-St3 antibody. Boxed regions (left panels) are displayed as magnified grayscale images in right panels. (E) Quantification of both endogenous St3 puncta density from D and transfected St3 puncta density from C plotted as number of St3 puncta per 10 μm. Data are displayed as mean ± SEM for transfected St3 in untreated cells (5.9 ± 0.6) or for nicotine-treated cells (10.6 ± 1.0) (n = 9 neurons and 18 dendrites per group, ***p < 0.000001); and for endogenous St3 in untreated cells (11.1 ± 0.8) or nicotine-treated cells (16.1 ± 1.9) (n = 8–11 neurons per group, **p < 0.008). (F–H) Activity-dependent changes. (F) Neurons transfected with St3-Halo and Venus were treated with bicuculline to increase synaptic activity and Golgi structures (St3-Halo) and cytoplasm (Venus) imaged. Low magnified images of neurons treated with (right) or without (vehicle, left) 20 μM bicuculline for 17 hr. Scale bar, 20 µm. Below are images of corresponding dendrites (black rectangles above) transfected with St3-Halo and Venus. Scale bar, 5 µm. (G) Neurons transfected with St3-Halo and Venus were treated first with 200 μM APV for 2 days to block NMDA-type glutamate receptors and then the APV was either continued (chronic APV) or withdrawn for 1 day to increase synaptic activity (APV withdrawal). The Golgi was then imaged using St3-Halo. Low magnified images show untreated neurons (vehicle), neurons treated chronically with APV, and neurons having APV withdrawed. Scale bar, 20 µm. The boxed regions are shown as enlarged images below this, and show the increase in St3-Halo labeled puncta in response to APV withdrawal. Scale bar, 5 µm. (H) Quantification of data from F and G measuring number of St3 puncta in dendrites per 10 μm. Data are displayed as mean ± SEM. Left: untreated cells, 1.5 ± 0.2; bicuculline-treated cells, 2.3 ± 0.3 (n = 12 neurons per group, *p < 0.04). Right: untreated cells, 1.5 ± 0.2; chronic APV cells, 1.6 ± 0.2; APV withdrawal cells, 2.5 ± 0.3 (n = 7 neurons per group, *p < 0.03). Quantitative analysis was conducted on two independent culture preparations.
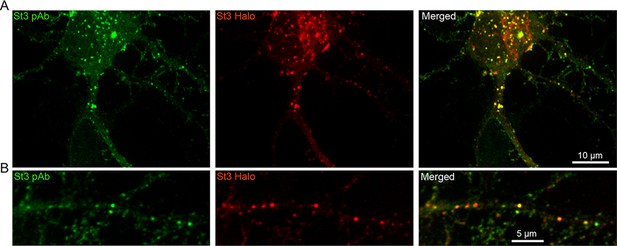
Specificity of the St3 polyclonal antibody (pAb).
Images of the soma and dendrites of a neuron expressing St3-Halo (red) and stained with the St3 pAb (green). Cultures were transfected with St3-Halo for 48 hr, then fixed and stained with the St3 pAb. The St3 pAb labeled the majority of St3-Halo vesicles in the somata (A) and dendrites (B).
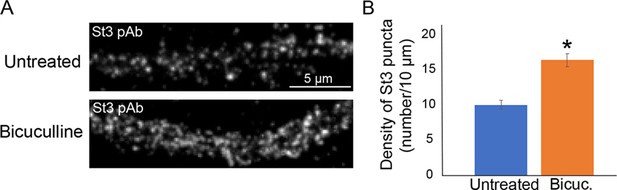
Bicuculline treatment of non-transfected neuronal cultures resulted in the same increase in the number of St3 antibody-stained puncta as nicotine treatment, which required exogenous expression of α4β2Rs to observe the increase.
(A) DIV12 cortical neurons were treated with (bottom panel) or without (top panel) 20 µM bicuculline for 17 hr to increase synaptic activity. Scale bar, 5 µm. (B) Quantification of endogenous St3 puncta density plotted as number of St3 puncta per 10 µm. Data are displayed as mean ± SEM for untreated cells (10.1 ± 0.6) or for bicuculline-treated cells (16.5 ± 1.0) (n = 7 neurons per group, *p < 0.0006).
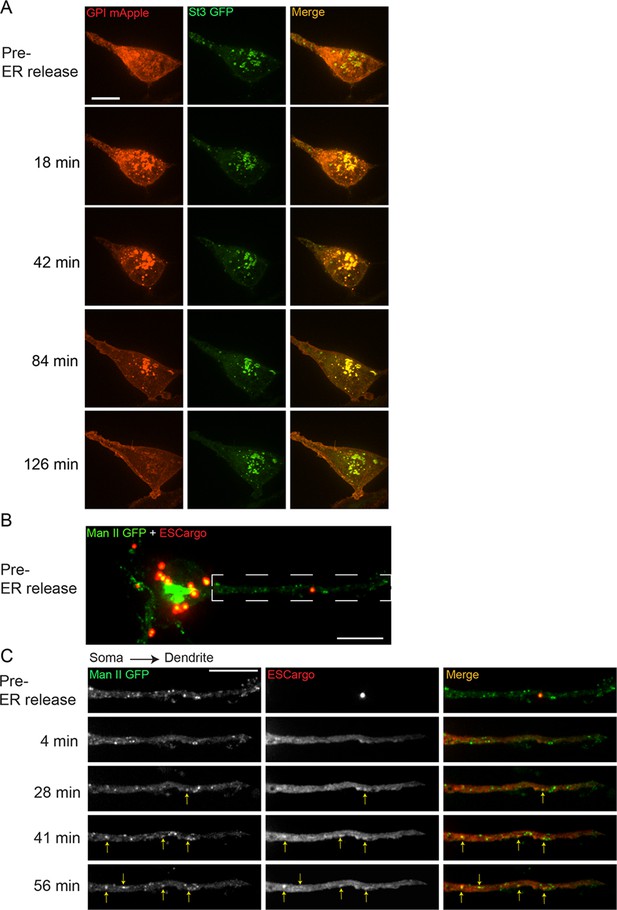
Released endoplasmic reticulum (ER) cargo traffic through Golgi satellites to the cell surface in nicotine-treated α4β2R-expressing cells and neurons.
(A) The retention using selective hooks (RUSH) system was used to synchronize release of ER cargo (RUSH-GPI-mApple) in nicotine-treated (10 mM, 17 hr) α4β2R cells. Cells were transfected with St3-GFP and RUSH-GPI-mApple (retained in the ER via an ER-targeted streptavidin/streptavidin binding peptide hook) for 24 hr and then treated with 10 μM nicotine for 17 hr. Scale bar, 10 µm. GPI-mApple traffics through dispersed Golgi elements following biotin-mediated release from ER. (B–C) Released ER cargo traffic through Golgi satellites in dendrites. Cortical cultures (DIV 10) were transfected with Man II-GFP, a modified ESCargo that forms aggregates in the ER lumen, and HA-tagged α4β2R subunits. After 24 hr, neurons were treated with nicotine for 17 hr and then imaged for Man II-GFP (green) and ESCargo (red), which is aggregated prior to ER release. Scale bar, 10 μm. (B) Image of the soma and dendrite before cargo release from the ER. Scale bar, 10 µm. (C) Live imaging of the dendrite boxed in B. Displayed are the ER cargo, ESCargo (red), and the Golgi satellites, marked by Man II-GFP (green) at the indicated times before (pre-release) and after addition of a synthetic ligand that dissolves the ER aggregates, allowing ER exit. Cargo trafficking through Golgi satellites are marked by yellow arrows. Image frames were acquired every 4 min for 1 hr. Scale bar, 10 µm.
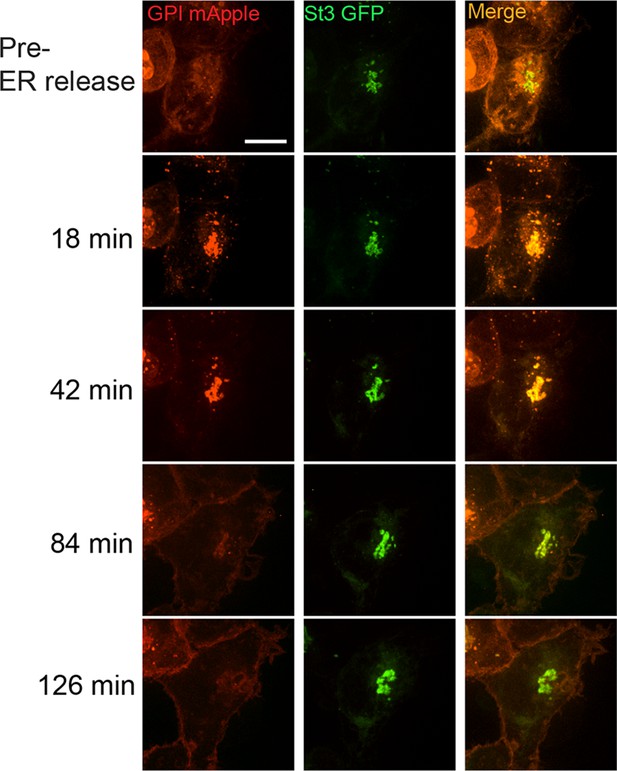
α4β2R cells were transfected with St3-GFP and retention using selective hooks (RUSH)-GPI-mApple (retained in the endoplasmic reticulum (ER) via an ER-targeted streptavidin/streptavidin binding peptide hook) for 24 hr.
Live imaging of cells showing transport of GPI-mApple to plasma membrane occurring via intact Golgi following biotin-mediated release from ER.
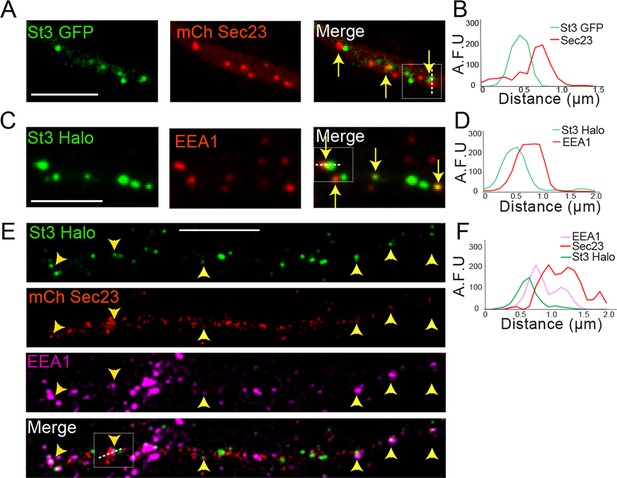
Dendritic Golgi satellites localize near endoplasmic reticulum exit sites (ERESs) and endosomes.
(A) Golgi satellites (St3-GFP) frequently pair with ERESs (mChSec 23) in dendrites. Primary cortical cultures were transfected with St3-GFP, mCh-Sec23, and HA-tagged α4β2R subunits. Neurons were treated with nicotine for 17 hr and fixed. Dendrites were imaged for Golgi satellites (St3-GFP, green) and ERESs (mChSec 23, red). Closely aligned Golgi satellites and ERESs are marked by yellow arrows. Scale bar, 5 µm. (B) An example of the close association between Golgi satellites (green) and ERESs (red). Signal intensity of the Golgi satellite and ERESs measured in arbitrary fluorescent units (AFU) in a line scan through the pair in the boxed area. (C) Golgi satellites (St3-GFP) frequently pair with early endosomes (EEA1 staining) in dendrites. Neurons were transfected with St3-GFP and after nicotine treatment, fixed and stained with antibodies to EEA1 before imaging as in A. Golgi satellites closely aligned with early endosomes are marked by yellow arrows. Scale bar, 5 µm. (D) An example of the close association between Golgi satellites (green) and early endosomes (red). Signal intensity of the two compartments was measured and displayed as in A. (E) Golgi satellites (St3-Halo) frequently form triads with ERESs (mChSec 23) and early endosomes (EEA1 staining) in dendrites. Neurons were transfected with St3-GFP, mCh-Sec23, and treated with nicotine for 17 hr before fixation and antibody labeling for EEA1 and imaging. Golgi puncta (green) closely aligned with ERESs (red) and early endosomes (mauve) are marked by yellow arrow heads. Scale bar, 10 µm. (F) An example of the close association between Golgi satellites (green), ERESs (red), and early endosomes (mauve). Signal intensity for markers of the three organelles was measured and displayed as in A and C.
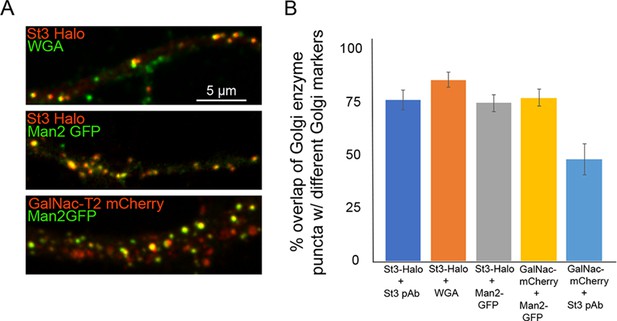
Moderate exogenous expression of the Golgi enzymes, Man II-GFP, GalNac-T2-mCherry, and St3-Halo in pairs, or singly, along with labeling for internalized fluorescently tagged wheat germ agglutinin (WGA) or an St3 antibody.
(A) Cortical cultures were transfected with the indicated pairs of probes or were singly transfected and then either surface-labeled with WGA-Alexa 488 for 20 min, washed, then incubated at 37°C for 2 hr, or stained with a polyclonal antibody (pAb) against St3. Scale bar, 5 µm. (B) Quantification of the percentage of Golgi enzyme puncta that co-localized with different Golgi markers. Data are shown as mean ± SEM, St3-Halo and St3 pAb, 76.3 ± 4.9; St3-Halo and WGA, 85.8 ± 4.5; St3-Halo and Man II-GFP, 74.7 ± 3.6; GalNac-T2-mCherry and Man II-GFP, 77.4 + 3.9; GalNac-T2-mCherry and St3 pAb, 48.3 + 4.2. (n = 8–11 fields per group).
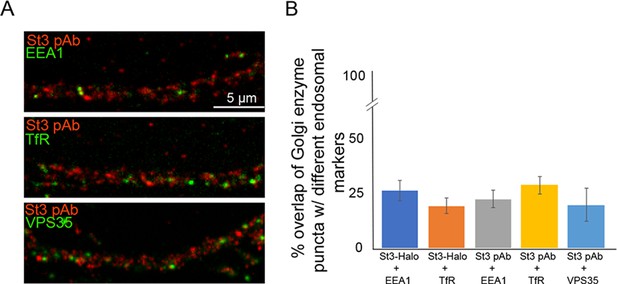
Measuring the punctal overlap between endogenous or expressed St3, and the endosomal markers, EEA1, internalized transferrin receptor, or VPS35.
(A) Cortical cultures fixed and stained with the St3 polyclonal antibody (pAb) or transfected with St3-Halo for 48 hr, were co-labeled with antibodies against EEA1 (early endosomes), VPS35 (retromer), or surface-internalized Tfr-Alexa 568 (recycling endosomes; cultures were live-labeled for 30 min, washed, and cells incubated at 37°C for 1 hr). Scale bar, 5 µm. (B) Quantification of the percentage of Golgi enzyme puncta that co-localized with different endosomal markers. Data are shown as mean ± SEM, St3-Halo and EEA1, 26.4 ± 4.8; St3-Halo and TfR, 19.4 ± 7.4; St3 pAb and EEA1, 22.6 ± 4.0; St3 pAb and TfR, 28.9 + 3.8; St3 pAb and VPS35, 19.9 + 2.6 (n = 5–10 fields per group).
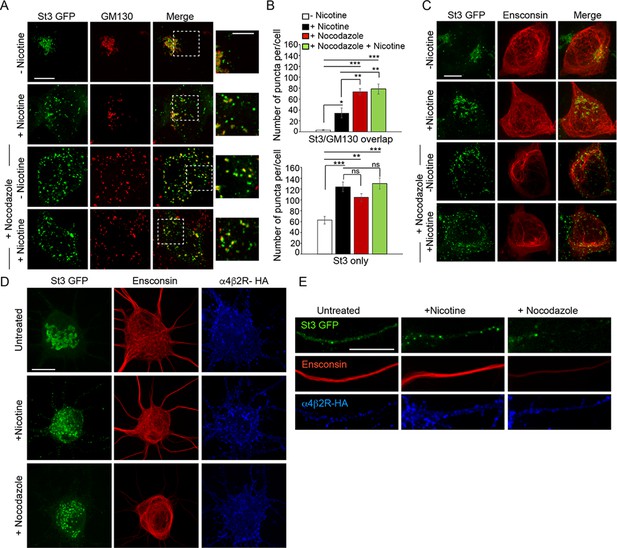
Similarities between Golgi dispersal induced by nicotine and nocodazole.
(A) Comparison of Golgi fragmentation in nocodazole-treated and/or nicotine-treated α4β2R cells. Cells were transfected with St3-GFP (green). Cells were treated (or left untreated) with 10 μM nicotine for 17 hr and further treated for 4 hr with 25 μM nocodazole. Cells were fixed, permeabilized, and immunostained with anti-GM130 antibody (red). Scale bar, 10 μm. Inset scale bar, 5 μm. (B) Quantification of the number of puncta per cell displaying St3/GM130 overlap (top) or St3 only (bottom). Data are shown as mean ± SEM, for St3/GM130 overlap, control cells, 3.1 ± 1.0; nicotine cells, 34.6 ± 9.0; nocodazole cells, 73.3 ± 6.0; nicotine and nocodazole cells, 78.5 ± 9.3 (n = 10 cells per group, con vs nic, *p < 0.035; con vs noc, ***p < 0.00003; con vs nic+ noc, ***p < 0.000003; nic vs noc, p < 0.007; nic vs nic+ noc, p < 0.003) and for St3 only, control cells, 62.6 ± 6.9; nicotine cells, 124.1 ± 8.9; nocodazole cells, 104.5 ± 6.9; nicotine and nocodazole cells, 130 ± 10 (n = 10 cells per group, con vs nic, ***p < 0.00005; con vs noc, **p < 0.004; con vs nic+ noc, ***p < 0.000005). (C) Effect of nicotine on microtubule stability. α4β2R cells were transfected with St3-GFP (green) and the microtubule binding protein, Ensconsin-mCherry (red). Cells were treated with nicotine and/or nocodazole as in A. Scale bar, 10 μm. (D–E) Comparison of the effect of nicotine and nocodazole on primary cortical cultures. Cultures of neurons (DIV 10) were transfected with HA-tagged α4β2R subunits, St3-GFP and Ensconsin-mCherry for 24 hr. Neurons were untreated, treated with 1 μM nicotine or 8 μM nocodazole for 17 hr. Surface α4β2Rs on neurons were labeled with anti-HA antibody (α4β2R-HA; blue). Scale bars, 10 μm. (D) Images of Ensconsin-mCherry (red), St3-GFP (green), and α4β2R-HA (blue) in the somata of untreated (top), nicotine- (middle) and nocodazole- (bottom) treated neurons. (E) Same as in D except for dendrites.
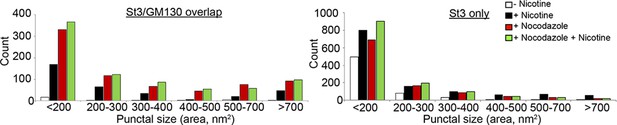
Histograms displaying the size distribution of St3/GM130 (left) and St3-only (right) puncta (8–10 cells per group).
α4β2R cells were treated with 10 μM nicotine for 17 hr, and further treated for 4 hr with 25 μM nocodazole. Parallel sets of cells were left untreated, treated with nicotine alone (17 hr), or nocodazole alone (4 hr).
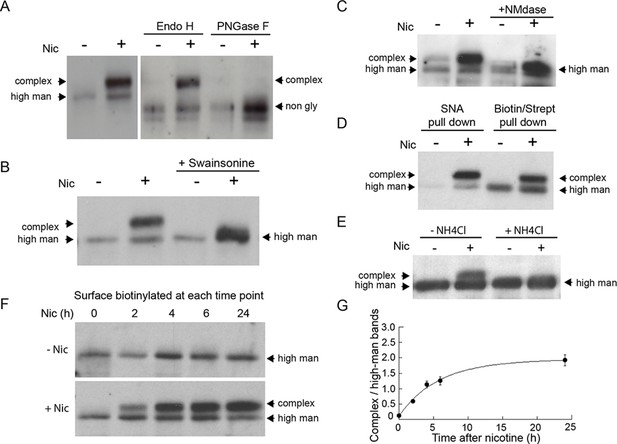
Nicotine exposure induces the modification of α4β2R N-linked glycans to complex forms.
(A) Endo H and PNGase F cleavage of surface α4 subunits from untreated or nicotine-treated α4β2R cells. Cells were untreated or nicotine-treated for 17 hr. Proteins on the cell surface were then biotinylated, solubilized, precipitated with streptavidin agarose and glycosidase-treated with Endo H or PNGase F enzymes on the agarose. Afterward, eluted proteins were analyzed on sodium dodecyl sulfate (SDS)-polyacrylamide gel (PAGE) and immunoblotted using anti-α4 antibody. (B) Swainsonine treatment blocks nicotine-induced glycan modification of surface α4 subunits. α4β2R cells were treated with the α-mannosidase inhibitor, swainsonine, for 2 hr and then swainsonine and nicotine for 17 hr. Afterward, samples were prepared as in A. (C) Neuraminidase (NMdase) cleavage of surface α4 subunits from untreated (-nicotine) or nicotine-treated (+nicotine) α4β2R cells. α4β2R cells were prepared as in A except with NMdase, which cleaves sialic acid, replacing the other glycosidase enzymes. (D) Sambucus Nigra (SNA) lectin recognizes α4 subunits after nicotine treatment. Samples were prepared as in A, and after solubilization, proteins were precipitated with the agarose-conjugated sialic acid-recognizing, lectin SNA and then analyzed by immunoblotting with anti-α4 antibody (left) and compared to samples precipitated with with streptavidin agarose as in A–C (right). (E) Ammonium chloride treatment blocks nicotine-induced glycan modification of surface α4 subunits. α4β2 cells were treated with or without ammonium chloride (NH4Cl; 10 mM) to inhibit activity of sialyltransferases that require an acidic environment to function. Samples were processed as in B, with NH4Cl replacing swainsonine. (F) Time course of surface α4 subunit glycan modification. α4β2R cells were nicotine-treated (+Nic) or untreated (-Nic) for displayed times, after which the cells were surface biotinylated and surface α4 subunits processed as in the previous panels. (G) Quantification of the time course of surface α4 subunit glycan modification. Densitometry of α4 subunit bands from three separate experiments, as in F, was preformed and the ratio of complex-trimmed (upper) band to the high-mannose (lower) bands are plotted as the mean ± SEM.
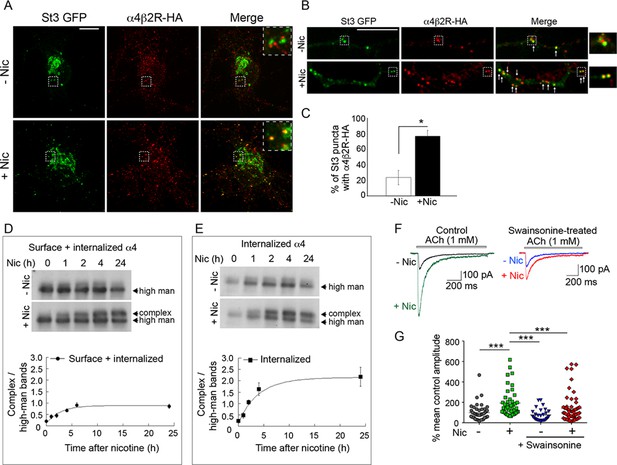
Surface α4β2Rs glycan modification and functional changes after endocytosis and trafficking to Golgi satellites.
(A–B) Endocytosed α4β2Rs co-localize with Golgi satellites in the somata (A) and dendrites (B) of neurons. Scale bars, 10 µm. Cortical neurons were transfected with St3-GFP (green) and HA-tagged α4β2R subunits and treated with (+Nic) or without (-Nic) 1 μM nicotine for 17 hr. To measure α4β2R endocytosis, cultures were labeled with anti-HA Ab for 30 min, washed, and cells incubated at 37°C for 2 hr. Afterward, cells were acid washed to remove surface receptor Abs, fixed, permeabilized, and endocytosed α4β2Rs visualized (red). Arrows mark where endocytosed α4β2Rs co-localize with Golgi satellites. (C) Quantification of the percentage of Golgi satellites that co-localized with endocytosed α4β2R. Data are displayed as mean ± SEM, control cells, 23.8 ± 9.0; nicotine cells, 76.9 ± 7.5 (n = 7–11 neurons per group, *p < 0.0002). Quantitative analysis was conducted on three independent culture preparations. (D) Time course of the α4 subunit glycan modification of the surface and internalized pools of α4β2Rs. Top: α4β2R cells were surface biotinylated with cleavable sulfo-NHS S-S biotin at time 0. Cultures were incubated at 37°C and followed in the presence or absence of 10 μM nicotine for the indicated times (hr). At each time point, biotinylated proteins, both cell surface and internalized/endocytosed, were precipitated with streptavidin agarose, and immunoblotted with anti-α4 antibody. Bottom: Densitometry of α4 subunit bands from four separate experiments as in top panel and plotted as in Figure 6G. (E) Time course of the α4 subunit glycan modification of the internalized pools of α4β2Rs. Top: Surface biotinylation was performed as in D at time 0. At each time point, surface biotin was cleaved using glutathione. The remaining internalized/endocytosed biotinylated receptors were isolated using streptavidin agarose and analyzed using immunoblot. Bottom: Densitometry of α4 subunit bands from three separate experiments as in top panel and plotted as in Figure 6G. (F–G) Block of α4β2R glycan modification by α-mannosidase inhibitor, swainsonine, prevents α4β2R functional upregulation by nicotine. (F) Swainsonine treatment blocks nicotine-induced increases in α4β2R current responses. Control (left): A 17–20 hr treatment with nicotine (Nic, 10 μM) induced an approximately fivefold, increase in ACh-evoked (1 mM ACh) current amplitudes in α4β2R-expressing HEK cells. Swainsonine-treated (right): ACh-evoked (1 mM ACh) current amplitudes for swainsonine and nicotine-treated cells. α4β2R cells were treated with swainsonine, for 2 hr and then swainsonine and nicotine for 17 hr. (G) Scatter plot of all ACh-evoked current amplitudes plotted as the percentage of mean control current amplitude. Control vs. nicotine p < 0.0001; nicotine vs. control and swainsonine-treated p < 0.001; nicotine vs. nicotine and swainsonine-treated p = 0.0054; control and swainsonine-treated vs. nicotine and swainsonine-treated p = 0.082 (not significant).
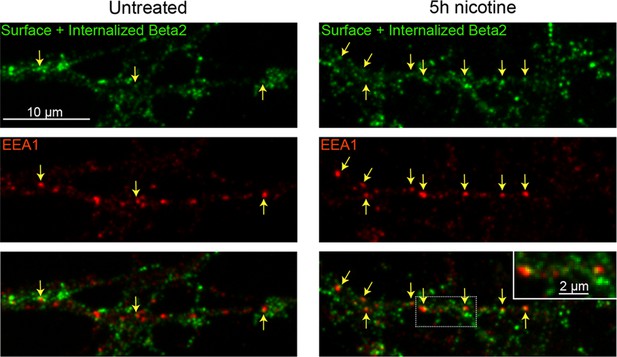
α4β2R-HA co-locolizes with EEA1-labeled endosomes in neurons 5 hr after endocytosis.
Cortical neurons were transfected for 2 days with HA-tagged α4β2R subunits and treated with (right panels) or without (left panels) 1 µM nicotine for 5 hr. To visualize α4β2R endocytosis, cultures were labeled with anti-HA Ab for 30 min, washed, and cells incubated at 37°C for 1.5 hr. Afterward, cells were fixed, permeabilized, and surface and endocytosed α4β2Rs detected with secondary antibodies (green). Early endosomes were labeled by immunostaining with anti-EEA1 antibody (red). Arrows mark where endocytosed α4β2Rs overlap with EEA1 puncta. Scale bar, 10 µm. Inset scale bar, 2 µm.
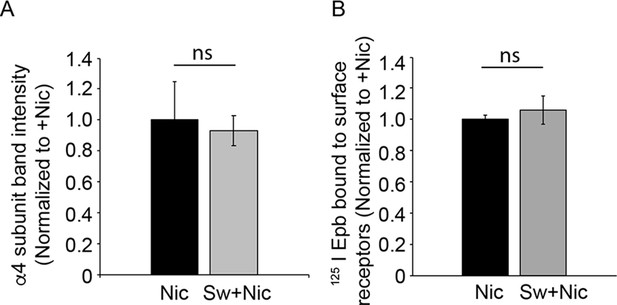
Effect of swainsonine on α4β2R surface expression.
(A) Swainsonine treatment along with nicotine did not significantly alter the level of α4 subunit on the cell surface compared to cells treated with nicotine alone. α4β2R cells were treated with the α-mannosidase inhibitor, swainsonine, for 2 hr and then swainsonine and nicotine for 17 hr. Cells were processed and surface α4 subunits prepared. Briefly, proteins were surface biotinylated, solubilized, precipitated with streptavidin agarose. After, eluted proteins were analyzed on sodium dodecyl sulfate (SDS)-polyacrylamide gel (PAGE) and immunoblotted using anti-α4 antibody. α4 subunit band intensity was quantified from four independent experiments. Densitometry of α4 subunit bands from four separate experiment was preformed and plotted as the mean ± SEM. t-test, p = 0.7, ns. (B) Cells were processed as in A. Cell surface proteins were biotinylated and precipitated with streptavidine agarose and subjected to 125I epibatidine binding assay to measure the high affinity nicotine binding sites on the cell surface. Data represents experiments performed in triplicates from two independent experiments. t-test, p = 0.15, ns.
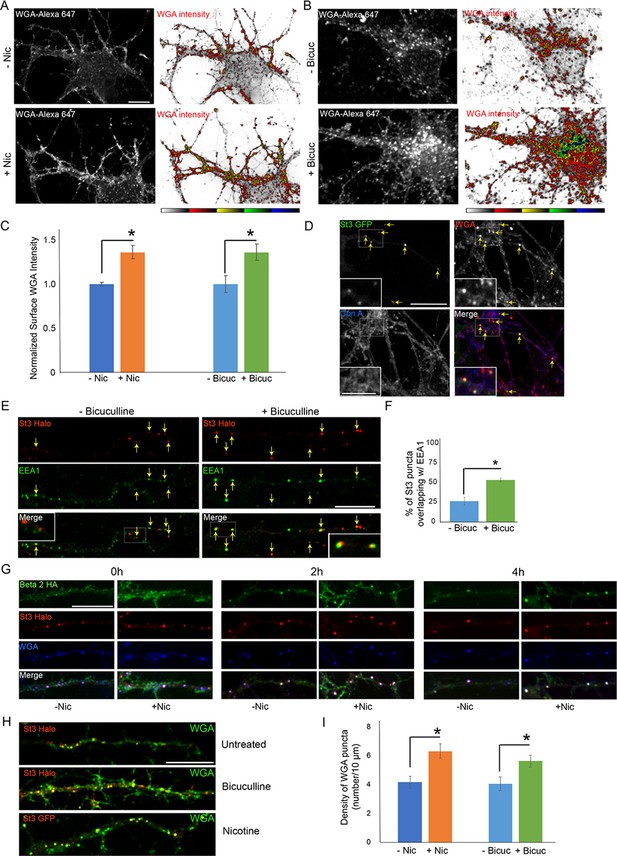
Neuronal stimulation increases lectin binding and its endocytosis into Golgi satellites.
(A–B) Neuronal stimulation by nicotine exposure (A) or increased synaptic input with bicuculline treatment (B) increased levels of wheat germ agglutinin (WGA) surface staining. Scale bars, 10 µm. (A) Cortical cultures were transfected with HA-tagged α4β2R subunits, treated with or without 1 μM nicotine for 17 hr and cultures were live-labeled with WGA-Alexa 647 for 20 min. Images displaying surface WGA labeling were obtained after cultures were fixed. Pseudocolor intensity profiles are shown in the right panels. (B) Following overnight bicuculline treatment, untransfected cultures were processed, surface labeled, and displayed as in A. (C) Quantification of surface WGA intensities from A and B. Data are shown as normalized mean ± SEM, left; nicotine experiment, control neurons, 1.0 ± 0.02; nicotine-treated neurons, 1.4 ± 0.07 (n = 7–11 neurons per group, *p < 0.0007), right; bicuculline experiment, control neurons, 1.0 ± 0.1; bicuculline-treated neurons, 1.4 ± 0.09 (n = 14–16 neurons per group, *p < 0.006). Quantitative analysis was conducted on two independent culture preparations. (D) Differences between Con-A and WGA surface labeling when endocytosis of lectins occurs. Cortical cultures transfected with St3-GFP were surface-labeled with WGA-Alexa 568 and Con A-Alexa 647 for 20 min, washed, then incubated at 37°C for 2 hr. WGA, but not Con A, was rapidly internalized and overlapped with St3-containing Golgi satellites (yellow arrows). Insets are higher magnification of hatched boxes. Scale bar, 10 µm. Inset scale bar, 2.5 µm. (E) Neuronal excitation leads to increased association of dispersed Golgi in dendrites with early endosomal membranes. Cultures were transfected with St3-Halo, treated with or without bicuculline for 17 hr, and fixed, permeabilized, and immunostained with anti-EEA1 antibody (green). Insets are higher magnification of hatched boxes. Scale bar, 10 µm. (F) Quantification of the percentage of St3 puncta that overlapped with EEA1. Data are shown as mean ± SEM, control neurons, 26.4 ± 4.8; bicuculline-treated neurons, 53.5 ± 2.3 (n = 10 fields per group, *p < 0.0007). (G) WGA is endocytosed with α4β2Rs and traffics into Golgi satellites. Cortical neurons were transfected with St3-Halo, HA-tagged α4β2R subunits and treated with 1 μM nicotine for 17 hr. α4β2R endocytosis was imaged by antibody feeding as in Figure 7A and B (anti-HA, green), and ST3-Halo labeled with Halo JF 594 (red), and WGA with WGA-Alexa 647 (blue). After labeling at time 0, neurons were incubated at 37°C for the indicated times to follow their endocytosis. As in D, WGA showed rapid internalization into St3 vesicles during the 30 min incubation period (0 hr), and over the time course, endocytosed β2HA showed increased co-localization with vesicles containing both WGA and St3-Halo. Scale bar, 10 µm. (H) Cultures were transfected with St3-Halo alone, or along with α4 and β2HA subunits (nicotine treatment), for 24 hr, then treated with and without bicuculline or nicotine for 17 hr, and surface labeled for 30 min with Halo JF 594 and WGA-Alexa 647. Cells were washed and incubated further for 2 hr to allow for WGA internalization. Scale bar, 10 µm. (I) Quantification of WGA puncta densities from F. Data are shown as mean ± SEM, left; nicotine experiment, control neurons, 4.1 ± 0.4; nicotine-treated neurons, 6.3 ± 0.5 (n = 6 fields per group, *p < 0.00003), right; bicuculline experiment, control neurons, 4.0 ± 0.5; bicuculline-treated neurons, 5.6 ± 0.4 (n = 6–8 fields per group, *p < 0.02). Quantitative analysis was conducted on two independent culture preparations.
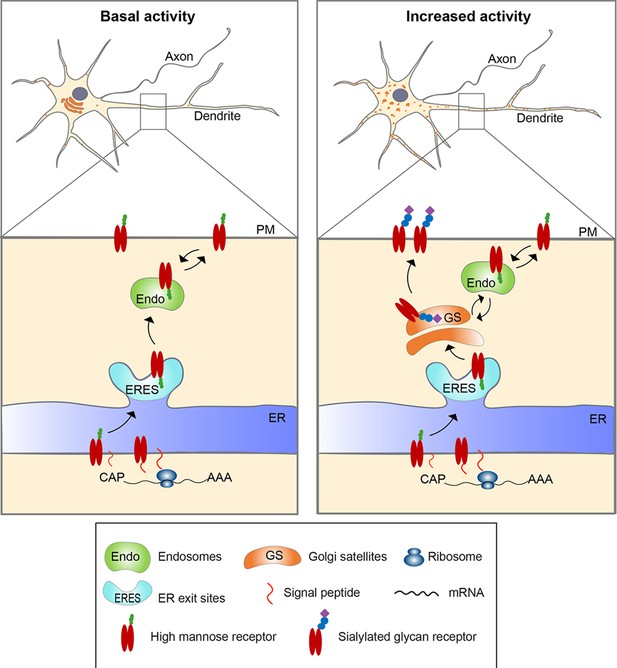
Model for activity-induced induction of Golgi satellites in dendrites.
In neurons with little excitatory activity, locally synthesized membrane and secreted proteins in dendrites appear to bypass Golgi satellites, trafficking through endosomes to the cell surface, as reported previously (Bowen et al., 2017). With increased excitatory activity, increasing numbers of Golgi satellites arise from local endoplasmic reticulum exit sites (ERESs). Locally synthesized membrane and secreted proteins then traffic through these Golgi satellites before reaching the cell surface, having their N-linked glycans modified from high-mannose types to sialic acid-containing, complex types. Glycoproteins already on the cell surface can also become modified to complex types by being endocytosed to endosomes that traffic cargo back to the nearby Golgi satellites.