Cellular Organisation: Putting organelles in their place
Cells contain an assortment of organelles which each have their own specialized role. To work correctly, most organelles need to be properly positioned within the cell. For example, mis-localization of the cell’s largest organelle, the nucleus, has been observed in neuromuscular diseases, such as Emery-Dreyfuss muscular dystrophy (Luxton and Starr, 2014).
Current models suggest that positioning of the nucleus relies on a complex called LINC (short for Linker of Nucleoskeleton and Cytoskeleton), which is made up of proteins that contain either a SUN or KASH domain. The SUN proteins (SAD1, UNC-84) sit across the inner nuclear membrane and connect to structures in the nucleus, such as chromatin and the nuclear lamina, and the KASH proteins (Klarsicht, ANC-1, Syne Homology) span across the outer nuclear membrane and interact with proteins in the cytoskeleton. The SUN and KASH domains of these proteins join together to form a bridge that mechanically couples the nucleus and cytoskeleton, which helps to anchor the nucleus in the right place (Kim et al., 2015).
This model of how nuclear positioning works is primarily based on experiments in Caenorhabditis elegans worms with mutations in the genes for either the UNC-84 or ANC-1 protein (Starr and Fridolfsson, 2010). The hypodermis of adult wild-type worms is made up of several huge hyp-7 cells (or syncytia) which each contain 139 evenly spaced nuclei (Shemer and Podbilewicz, 2000), making them a useful system for investigating nuclear anchorage. According to the model, if the nuclei in hyp-7 cells are exclusively anchored via the SUN–KASH bridge, then loss of the genes for UNC-84 or ANC-1 should have an identical effect and result in the same amount of nuclear clustering. However, in 2018, a group of researchers made a puzzling discovery: they found that deleting the gene for ANC-1 resulted in more severe nuclear clustering than removing the gene for UNC-84 (Cain et al., 2018). Now, in eLife, Daniel A Starr and co-workers from University California, Davis – including Hongyan Hao as first author – report that the model for how the nucleus is positioned may need re-defining (Hao et al., 2021).
The team (which includes some of the authors involved in the 2018 study) found, as expected, that removing the gene for ANC-1 led to nuclear clustering in hyp-7 cells, indicating that nuclear anchorage had been lost (Figure 1). The untethered nuclei also disrupted the network of microtubules in the cytoskeleton, and appeared much smaller and less rounded, suggesting that the cells lacked mechanical stability. In C. elegans, the ANC-1 protein contains multiple domains: an actin-binding domain at its N-terminus, several cytoplasmic domains that likely bind to other proteins, a transmembrane domain, and a KASH domain at its C-terminus (Gundersen and Worman, 2013; Starr and Han, 2002). To gain a better understanding of how ANC-1 positions the nucleus, Hao et al. deleted these different domains, either separately or in combination, to see how this affected the protein’s role in the cell.
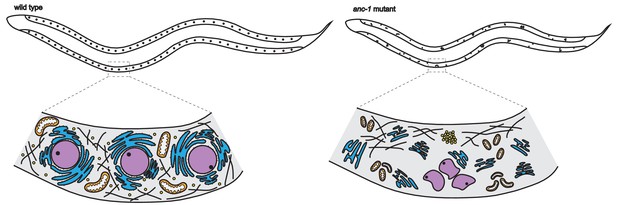
Loss of ANC-1 leads to unanchored and misshaped organelles and a smaller body size in worms.
The hypodermis of C. elegans worms (top schematic) is made up of hyp-7 cells which contain over a hundred nuclei (represented as black dots). In wild-type worms (left), the KASH protein ANC-1 (depicted as spikes) localizes to the membrane of the nucleus and endoplasmic reticulum (ER). As a result, the nuclei (purple) are spherical and evenly spaced, and the ER (blue), mitochondria (orange) and lipid droplets (yellow) are well anchored. The microtubule network (black lines) is also evenly distributed throughout the cytoplasm. Meanwhile, in mutant worms lacking the gene for ANC-1 (right), the ER and mitochondria are fragmented, and the nuclei are unanchored and clustered together. Lipid droplets are also clustered and the microtubule network is disrupted by the movement of the untethered organelles. This causes the mutant worm to have a smaller body size and the nuclei in its hyp-7 cells to be mispositioned.
Image credit: Patricia Ulm.
Deletion of the KASH domain only caused moderate nuclear clustering, and loss of the actin-binding domain did not generate any nuclear anchorage defects. In contrast, deleting parts of ANC-1 protein that sit within the cytoplasm and likely bind to other cytoskeleton proteins led to more severe positioning defects. Notably, nuclear mispositioning was greatly increased in double mutants lacking both a functional SUN domain in UNC-84 and a cytoplasmic domain of ANC-1. This synergistic effect suggests that nuclear positioning is likely controlled by cooperation between two distinct domains of ANC-1: the cytoplasmic domain and the domain that binds to UNC-84 in the SUN-KASH bridge.
Previous studies have shown that ANC-1 also controls the distribution of mitochondria (Starr and Han, 2002). Therefore, Hao et al. investigated whether ANC-1 is also essential for positioning the mitochondria and another organelle called the endoplasmic reticulum (or ER for short). In mutant worms lacking the gene for ANC-1, they found unanchored fragments of mitochondria and the ER along with clusters of lipid droplets (Figure 1). As seen for the nucleus, deleting the KASH domain only resulted in mild defects in ER positioning, suggesting that localization of the ER also mainly relies on parts of the ANC-1 protein outside the KASH domain.
These findings suggest that ANC-1 positions the nucleus and ER largely independently from the KASH domain. Further experiments revealed that the ANC-1 protein is also located on the membrane of the ER. This led Hao et al. to propose a new cytoplasmic integrity model in which ANC-1 localizes to both the ER and nuclear membranes, and reaches out to correctly position organelles via an interconnecting network that permeates the entire cytoplasm.
This study raises the question of how ANC-1 can position and hold organelles in place with little or no help from its KASH and actin-binding domains. Notably, studies in mice have also shown that the actin-binding domains of Nesprin1 (mouse ANC-1) are not essential to anchor the nucleus within the cytoplasm (Stroud et al., 2017). Therefore, some important questions remain: which cytoskeletal component(s) work with ANC-1 to correctly position organelles and confer mechanical stability to the cell? Are the neuromuscular diseases associated with mutations in the LINC complex the result of alterations in cytoplasmic integrity rather than nuclear anchorage defects? Further experiments using C. elegans as a model system may help to answer these questions and shed further light on how ANC-1 anchors organelles in place.
References
-
Making the LINC: SUN and KASH protein interactionsBiological Chemistry 396:295–310.https://doi.org/10.1515/hsz-2014-0267
-
KASHing up with the nucleus: novel functional roles of KASH proteins at the cytoplasmic surface of the nucleusCurrent Opinion in Cell Biology 28:69–75.https://doi.org/10.1016/j.ceb.2014.03.002
-
Fusomorphogenesis: Cell fusion in organ formationDevelopmental Dynamics 218:30–51.https://doi.org/10.1002/(SICI)1097-0177(200005)218:1<30::AID-DVDY4>3.0.CO;2-W
-
Interactions between nuclei and the cytoskeleton are mediated by SUN-KASH nuclear-envelope bridgesAnnual Review of Cell and Developmental Biology 26:421–444.https://doi.org/10.1146/annurev-cellbio-100109-104037
-
Nesprin 1α2 is essential for mouse postnatal viability and nuclear positioning in skeletal muscleJournal of Cell Biology 216:1915–1924.https://doi.org/10.1083/jcb.201612128
Article and author information
Author details
Publication history
- Version of Record published: May 21, 2021 (version 1)
Copyright
© 2021, Ulm and Jantsch
This article is distributed under the terms of the Creative Commons Attribution License, which permits unrestricted use and redistribution provided that the original author and source are credited.
Metrics
-
- 1,456
- views
-
- 114
- downloads
-
- 1
- citations
Views, downloads and citations are aggregated across all versions of this paper published by eLife.
Download links
Downloads (link to download the article as PDF)
Open citations (links to open the citations from this article in various online reference manager services)
Cite this article (links to download the citations from this article in formats compatible with various reference manager tools)
Further reading
-
- Cell Biology
Asymmetric cell divisions (ACDs) generate two daughter cells with identical genetic information but distinct cell fates through epigenetic mechanisms. However, the process of partitioning different epigenetic information into daughter cells remains unclear. Here, we demonstrate that the nucleosome remodeling and deacetylase (NuRD) complex is asymmetrically segregated into the surviving daughter cell rather than the apoptotic one during ACDs in Caenorhabditis elegans. The absence of NuRD triggers apoptosis via the EGL-1-CED-9-CED-4-CED-3 pathway, while an ectopic gain of NuRD enables apoptotic daughter cells to survive. We identify the vacuolar H+–adenosine triphosphatase (V-ATPase) complex as a crucial regulator of NuRD’s asymmetric segregation. V-ATPase interacts with NuRD and is asymmetrically segregated into the surviving daughter cell. Inhibition of V-ATPase disrupts cytosolic pH asymmetry and NuRD asymmetry. We suggest that asymmetric segregation of V-ATPase may cause distinct acidification levels in the two daughter cells, enabling asymmetric epigenetic inheritance that specifies their respective life-versus-death fates.
-
- Cell Biology
- Stem Cells and Regenerative Medicine
Extramedullary erythropoiesis is not expected in healthy adult mice, but erythropoietic gene expression was elevated in lineage-depleted spleen cells from Cd47−/− mice. Expression of several genes associated with early stages of erythropoiesis was elevated in mice lacking CD47 or its signaling ligand thrombospondin-1, consistent with previous evidence that this signaling pathway inhibits expression of multipotent stem cell transcription factors in spleen. In contrast, cells expressing markers of committed erythroid progenitors were more abundant in Cd47−/− spleens but significantly depleted in Thbs1−/− spleens. Single-cell transcriptome and flow cytometry analyses indicated that loss of CD47 is associated with accumulation and increased proliferation in spleen of Ter119−CD34+ progenitors and Ter119+CD34− committed erythroid progenitors with elevated mRNA expression of Kit, Ermap, and Tfrc. Induction of committed erythroid precursors is consistent with the known function of CD47 to limit the phagocytic removal of aged erythrocytes. Conversely, loss of thrombospondin-1 delays the turnover of aged red blood cells, which may account for the suppression of committed erythroid precursors in Thbs1−/− spleens relative to basal levels in wild-type mice. In addition to defining a role for CD47 to limit extramedullary erythropoiesis, these studies reveal a thrombospondin-1-dependent basal level of extramedullary erythropoiesis in adult mouse spleen.