Antidepressants: Where ketamine and dopamine collide
1987 was a watershed year in the history of antidepressants, with Prozac being the first selective serotonin reuptake inhibitor drug to be approved for use in the US. Prozac and other drugs that limit the reuptake of neurotransmitters such as serotonin, norepinephrine and dopamine would dominate the market for the next three decades. These treatments were marked improvements over their predecessors, but it has become clear that they are not the silver bullet they were once touted to be: moderate efficacy, insensitive populations, and untenable side effects have all limited their clinical utility (Warden et al., 2007).
Enter Ketamine. First synthesized over 75 years ago, this small, unassuming compound had so far been relegated to veterinary clinics as a pet anesthetic, while also doubling as a club drug that could induce dissociation and euphoria. In the early 2000s, however, reports started to emerge suggesting that a single dose of ketamine could have profound and lasting antidepressant effects (Berman et al., 2000). After over a decade of research, the US Food and Drug Administration (FDA) finally approved ketamine, in the form of ‘esketamine’, for the treatment of depression (Carboni et al., 2021). However, the mechanisms that drive the antidepressant effects of ketamine are poorly understood: this is unusual for an FDA-approved drug, although not unheard of for molecules used to treat affective disorders such as depression. How can a compound used to anesthetize cats or induce a psychedelic-like high have clinical utility?
Dopamine, the so-called ‘pleasure or reward neuromodulator’, is indispensable for regulating responses to rewards such as delicious foods, sex or addictive substances; however, it has also been implicated in certain mood disorders (Berridge, 2018). In fact, the emergence of depression has been linked to disruption in the activity of the dopamine-producing neurons present in the ventral tegmental area (VTA) of the brain, but few studies have examined whether ketamine elicits its antidepressant effects by altering the activity of these cells (Hamon and Blier, 2013). Now, in eLife, Yevgenia Kozorovitskiy and colleagues at Northwestern University – including Mingzheng Wu as first author – report how ketamine can strengthen brain circuits that include the VTA (Wu et al., 2021).
The researchers used a ‘learned helplessness’ experimental mouse model which mimics the blunted behavioral or emotional reactions that are one of the hallmarks of depression (Bylsma et al., 2008). The rodents were repeatedly exposed to mild electric shocks to the foot that were impossible to escape: over time, they learn that it was pointless to try to avoid this stressor, and they froze rather than try to escape. Antidepressants – including ketamine, as Wu et al. now show – alleviate this helplessness and restore escape behaviors. The researchers had also genetically manipulated the rodents to introduce a bioengineered molecule that emits light when neurons become activated, with the change in fluorescence being used as a proxy for neuronal activity (Inoue, 2020). The experiments revealed that in mice with learned helplessness, the activity pattern of VTA neurons was abnormal: however, ketamine treatment could reverse this disruption (Figure 1).
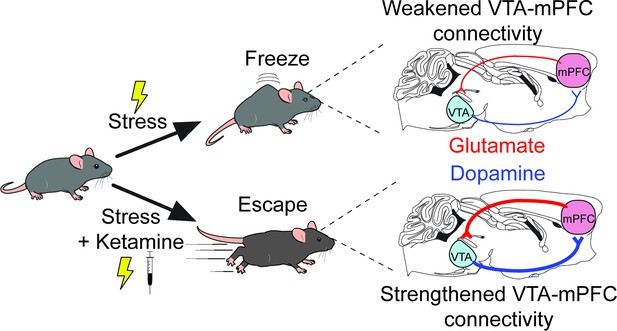
A new mechanism for the antidepressant effects of ketamine.
Over time, mice that are exposed to repeated mild electric shocks from which it is not possible to escape stop trying to avoid this stressor; instead, they develop learned helplessness and ‘freeze’ rather than attempt to escape, a behavior reminiscent of the reduced behavioral responses observed in depression (top). This exposure to stress disrupts the activity of a circuit formed by dopamine-producing projections (blue lines) and glutamate-producing projections (red lines) that connect the ventral tegmental area (VTA) and the medial prefrontal cortex (mPFC). Receiving ketamine (bottom), however, has an antidepressant effect. The drug alleviates learned helplessness and allows the mice to display active coping behaviors such as trying to escape the stimuli, while also reversing the disruption in the circuits between VTA and mPFC.
These data clearly demonstrated that VTA dopamine neurons are involved in responding to stressors, but whether ketamine acts by changing the activity of the neurons themselves remained unresolved. To test for a causal link, Wu et al. selectively inhibited VTA neurons by using another bioengineered molecule, a receptor called DREADD that can only be selectively activated by a synthetic molecule (Dobrzanski and Kossut, 2017). Ketamine had no effect when the VTA neurons were inhibited, demonstrating that VTA dopamine neuron activity is necessary for the drug to have an antidepressant effect.
Further physiology experiments were then conducted in the VTA to determine how ketamine could impact neuronal activity, with, somewhat unexpectedly, few noticeable effects emerging. This suggested that rather than acting locally, ketamine most likely worked by modulating the activity of an upstream brain region that influences VTA activity. The medial prefrontal cortex (mPFC for short) represented an attractive target because it sends dense projections to the VTA, and because it mediates, in part, the antidepressant effects of katamine (Vertes, 2004; Moda-Sava et al., 2019).
Indeed, Wu et al. discovered that injecting ketamine specifically into the mPFC (but not other VTA-projecting brain regions) replicated the improvements observed when the mice were administered the drug systemically. In addition, antidepressant-like effects that mirrored those induced by ketamine emerged when mPFC neurons that express the receptor for dopamine were artificially activated using excitatory DREADDs.
The research presented by Wu et al. provides a unique understanding of the antidepressant effects of ketamine on canonical reward circuits. Specifically, the results suggest that the drug acts by strengthening a recurrent neural circuit between the VTA and the mPFC, which allows ketamine’s antidepressant properties to persist long after the compound has been cleared from the body. The data pave the way for future studies that directly examine how the effects of ketamine are mediated by mPFC projections to the VTA. Ultimately, this knowledge will help to find and design more selective compounds which can target these circuits to treat depression and related disorders.
References
-
Antidepressant effects of ketamine in depressed patientsBiological Psychiatry 47:351–354.https://doi.org/10.1016/S0006-3223(99)00230-9
-
Evolving concepts of emotion and motivationFrontiers in Psychology 9:1647.https://doi.org/10.3389/fpsyg.2018.01647
-
A meta-analysis of emotional reactivity in major depressive disorderClinical Psychology Review 28:676–691.https://doi.org/10.1016/j.cpr.2007.10.001
-
Repurposing ketamine in depression and related disorders: can this enigmatic drug achieve success?Frontiers in Neuroscience 15:657714.https://doi.org/10.3389/fnins.2021.657714
-
Application of the DREADD technique in biomedical brain researchPharmacological Reports 69:213–221.https://doi.org/10.1016/j.pharep.2016.10.015
-
Monoamine neurocircuitry in depression and strategies for new treatmentsProgress in Neuro-Psychopharmacology and Biological Psychiatry 45:54–63.https://doi.org/10.1016/j.pnpbp.2013.04.009
-
The STAR*D project results: a comprehensive review of findingsCurrent Psychiatry Reports 9:449–459.https://doi.org/10.1007/s11920-007-0061-3
Article and author information
Author details
Publication history
Copyright
© 2021, Marcus and Bruchas
This article is distributed under the terms of the Creative Commons Attribution License, which permits unrestricted use and redistribution provided that the original author and source are credited.
Metrics
-
- 17,446
- views
-
- 263
- downloads
-
- 7
- citations
Views, downloads and citations are aggregated across all versions of this paper published by eLife.
Download links
Downloads (link to download the article as PDF)
Open citations (links to open the citations from this article in various online reference manager services)
Cite this article (links to download the citations from this article in formats compatible with various reference manager tools)
Further reading
-
- Neuroscience
Complex structural and functional changes occurring in typical and atypical development necessitate multidimensional approaches to better understand the risk of developing psychopathology. Here, we simultaneously examined structural and functional brain network patterns in relation to dimensions of psychopathology in the Adolescent Brain Cognitive Development dataset. Several components were identified, recapitulating the psychopathology hierarchy, with the general psychopathology (p) factor explaining most covariance with multimodal imaging features, while the internalizing, externalizing, and neurodevelopmental dimensions were each associated with distinct morphological and functional connectivity signatures. Connectivity signatures associated with the p factor and neurodevelopmental dimensions followed the sensory-to-transmodal axis of cortical organization, which is related to the emergence of complex cognition and risk for psychopathology. Results were consistent in two separate data subsamples and robust to variations in analytical parameters. Although model parameters yielded statistically significant brain-behavior associations in unseen data, generalizability of the model was rather limited for all three latent components (r change from within- to out-of-sample statistics: LC1within=0.36, LC1out=0.03; LC2within=0.34, LC2out=0.05; LC3within=0.35, LC3out=0.07). Our findings help in better understanding biological mechanisms underpinning dimensions of psychopathology, and could provide brain-based vulnerability markers.
-
- Neuroscience
In amniotes, head motions and tilt are detected by two types of vestibular hair cells (HCs) with strikingly different morphology and physiology. Mature type I HCs express a large and very unusual potassium conductance, gK,L, which activates negative to resting potential, confers very negative resting potentials and low input resistances, and enhances an unusual non-quantal transmission from type I cells onto their calyceal afferent terminals. Following clues pointing to KV1.8 (Kcna10) in the Shaker K channel family as a candidate gK,L subunit, we compared whole-cell voltage-dependent currents from utricular HCs of KV1.8-null mice and littermate controls. We found that KV1.8 is necessary not just for gK,L but also for fast-inactivating and delayed rectifier currents in type II HCs, which activate positive to resting potential. The distinct properties of the three KV1.8-dependent conductances may reflect different mixing with other KV subunits that are reported to be differentially expressed in type I and II HCs. In KV1.8-null HCs of both types, residual outwardly rectifying conductances include KV7 (Knq) channels. Current clamp records show that in both HC types, KV1.8-dependent conductances increase the speed and damping of voltage responses. Features that speed up vestibular receptor potentials and non-quantal afferent transmission may have helped stabilize locomotion as tetrapods moved from water to land.