Plant Ecology: Getting the metabolites right
All living organisms look different. Even within a species, individuals can show differences in their shape, size and coloring. This is true both for outward appearances and for physiological traits, such as the concentration of various metabolites. These variations are determined by the underlying genetics of the organism and by the environment it lives in. This results in phenotypic differences that affect the entire organism, allowing it to rapidly respond to changes in its environment. A well-known example of this is the change in color and pattern of butterfly wings under different temperatures, which is regulated by specific hormones (Bhardwaj et al., 2020).
The relationship between genotype and phenotype has been of interest since Mendel postulated the existence of 'internal factors' that are passed on to the next generation (Mendel, 1865), and Bateson originated the term 'genetics' (Bateson, 1909). This link is of major interest in fields ranging from evolutionary biology to molecular biology, and also medicine or agriculture, where untangling genetic effects on phenotypes from environmental effects can lead to beneficial interventions.
The mechanisms by which genetic differences translate into phenotypic differences involve many intermediate steps (Figure 1A); this so-called ‘in-between-ome’ consists of the transcriptome, the proteome and the metabolome. The integration of data from these different levels is necessary to understand how complex phenotypes evolve, and how they are regulated (Subramanian et al., 2020). In plants, the metabolome – all of the small molecules required for an organism to live – is particularly important for adaptation. This is because plants, being immobile, rely on the substances they can absorb from their environment and the derivatives they can produce. However, it is unclear how the variety of metabolites found in plants from different environments arose during evolution. Now, in eLife, Daniel J Kliebenstein, from the University of California Davis, along with colleagues from Austria, the United States and Germany – including Ella Katz as first author – report the factors that drive phenotypic differences in the chemical composition of a specific type of metabolites found in the plant Arabidopsis thaliana (Katz et al., 2021).
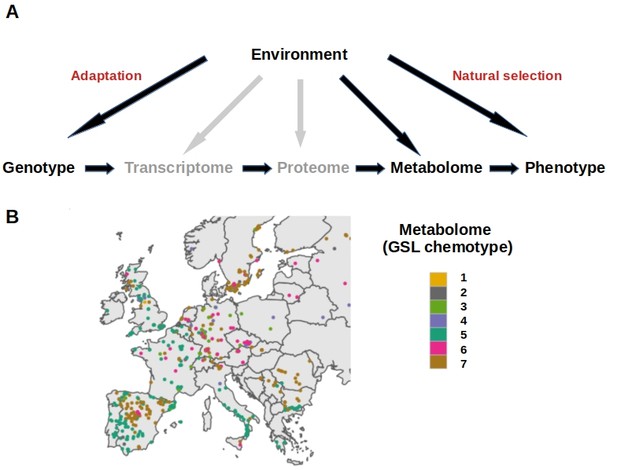
The complex interplay between genotype, phenotype and the environment.
(A) The environment of an organism influences its phenotype through natural selection, and its genome, which adapts to distinct environments. The mechanisms by which genetic differences translate into phenotypic variation are mediated by the ‘in-between-ome’. This consists of the transcriptome and the proteome, which are indirectly affected by the environment (shown in grey), and the metabolome, which is directly influenced by the environment (shown in black). (B) Map of Europe showing dots in different colors representing lines of A. thaliana with different glucosinolate profiles (GSL), also known as chemotypes. These chemotypes change depending on the environment, with plants from similar environments exhibiting similar chemotypes.
Katz et al. measured the levels of specialized metabolites called glucosinolates in nearly 800 lines of A. thaliana from different ecosystems in Europe. Glucosinolates are biologically active compounds that protect plants from pests and diseases. They are usually found in the Brassicaceae family (Rask et al., 2000), which includes plants like broccoli, cauliflower or mustard, and provide these plants with their characteristic taste. Additionally, plants use glucosinolates to counteract both biotic stress, caused by other living organisms such as herbivores that try to eat the plants, and abiotic stress, such as drought stress (Del Carmen Martínez-Ballesta et al., 2013). The fact that glucosinolates can protect plants from predators, pests and other stressors make it likely that the mechanisms that regulate the abundance of these compounds in individual plants are under strong selection and evolutionary constraints.
The analysis performed by Katz et al. revealed that plants from different regions in Europe had glucosinolates with different chemical compositions or ‘chemotypes’. The spatial distribution of the different chemotypes throughout Europe exhibits distinctive patterns, both at a local and a global scale (Figure 1B). But how did this trait variation evolve? What is its genetic basis and what phenotypic consequences does it have?
To answer these questions, Katz et al. used a technique called genome-wide association mapping (GWAS), which scans the genome of each plant for genetic markers that may be associated with the different chemotypes detected. This analysis found only two major sites in the genome that are responsible for variation in glucosinolate content. However, these previously known loci cannot explain all the variation observed, suggesting that additional loci must also be involved. Additionally, Katz et al. discovered previously unknown alleles at these sites and showed that different combinations of these alleles could lead to similar chemotypes in similar environments.
Based on these observations, Katz et al. suggest that these different combinations of alleles emerged through a mixture of evolutionary events. Some arose through parallel evolution, which occurs when mutations in the same gene lead to similar traits. Others are the result of convergent evolution, where independent mutations in different genes give rise to similar features. Apart from the genetic factors, Katz et al. also show how environmental parameters like climate and geography directly affect the chemotype of A. thaliana in different regions. Specifically, they found that specific environmental conditions were associated with certain chemotypes across different geographic locations.
Katz et al. show that many factors, both environmental and genetic, shape the chemotype of glucosinolates in A. thaliana. The findings highlight the complexity of the interplay between genetics and the environment, and how both contribute to the evolution of traits in natural populations. They also underline how complex traits appear in a population that is adapted to distinct environments. Additionally, this work demonstrates the need to correctly design studies that aim to explain and dissect complex traits, given that results can be confounded by individuals from the same species being adapted to distinct environmental conditions. Future work will show whether the findings of Katz et al. findings hold for other traits, and if they shed light on general principles of trait evolution.
References
-
BookHeredity and Variation in Modern Lights. Darwin and Modern ScienceCambridge University Press.https://doi.org/10.1017/CBO9780511693953.006
-
The physiological importance of glucosinolates on plant response to abiotic stress in BrassicaInternational Journal of Molecular Sciences 14:11607–11625.https://doi.org/10.3390/ijms140611607
-
Experiments in plant hybridizationProceedings of the Natural History Society of Brünn.
-
Myrosinase: gene family evolution and herbivore defense in BrassicaceaePlant Molecular Biology 42:93–114.https://doi.org/10.1023/A:1006380021658
-
Multi-omics data integration, interpretation, and its applicationBioinformatics and Biology Insights 14:117793221989905.https://doi.org/10.1177/1177932219899051
Article and author information
Author details
Publication history
Copyright
© 2021, Korte
This article is distributed under the terms of the Creative Commons Attribution License, which permits unrestricted use and redistribution provided that the original author and source are credited.
Metrics
-
- 1,241
- views
-
- 84
- downloads
-
- 2
- citations
Views, downloads and citations are aggregated across all versions of this paper published by eLife.
Download links
Downloads (link to download the article as PDF)
Open citations (links to open the citations from this article in various online reference manager services)
Cite this article (links to download the citations from this article in formats compatible with various reference manager tools)
Further reading
-
- Ecology
- Microbiology and Infectious Disease
Interspecies interactions involving direct competition via bacteriocin production play a vital role in shaping ecological dynamics within microbial ecosystems. For instance, the ribosomally produced siderophore bacteriocins, known as class IIb microcins, affect the colonization of host-associated pathogenic Enterobacteriaceae species. Notably, to date, only five of these antimicrobials have been identified, all derived from specific Escherichia coli and Klebsiella pneumoniae strains. We hypothesized that class IIb microcin production extends beyond these specific compounds and organisms. With a customized informatics-driven approach, screening bacterial genomes in public databases with BLAST and manual curation, we have discovered 12 previously unknown class IIb microcins in seven additional Enterobacteriaceae species, encompassing phytopathogens and environmental isolates. We introduce three novel clades of microcins (MccW, MccX, and MccZ), while also identifying eight new variants of the five known class IIb microcins. To validate their antimicrobial potential, we heterologously expressed these microcins in E. coli and demonstrated efficacy against a variety of bacterial isolates, including plant pathogens from the genera Brenneria, Gibbsiella, and Rahnella. Two newly discovered microcins exhibit activity against Gram-negative ESKAPE pathogens, i.e., Acinetobacter baumannii or Pseudomonas aeruginosa, providing the first evidence that class IIb microcins can target bacteria outside of the Enterobacteriaceae family. This study underscores that class IIb microcin genes are more prevalent in the microbial world than previously recognized and that synthetic hybrid microcins can be a viable tool to target clinically relevant drug-resistant pathogens. Our findings hold significant promise for the development of innovative engineered live biotherapeutic products tailored to combat these resilient bacteria.
-
- Ecology
For the first time in any animal, we show that nocturnal bull ants use the exceedingly dim polarisation pattern produced by the moon for overnight navigation. The sun or moon can provide directional information via their position; however, they can often be obstructed by clouds, canopy, or the horizon. Despite being hidden, these bodies can still provide compass information through the polarised light pattern they produce/reflect. Sunlight produces polarised light patterns across the overhead sky as it enters the atmosphere, and solar polarised light is a well-known compass cue for navigating animals. Moonlight produces an analogous pattern, albeit a million times dimmer than sunlight. Here, we show evidence that polarised moonlight forms part of the celestial compass of navigating nocturnal ants. Nocturnal bull ants leave their nest at twilight and rely heavily on the overhead solar polarisation pattern to navigate. Yet many foragers return home overnight when the sun cannot guide them. We demonstrate that these bull ants use polarised moonlight to navigate home during the night, by rotating the overhead polarisation pattern above homing ants, who alter their headings in response. Furthermore, these ants can detect this cue throughout the lunar month, even under crescent moons, when polarised light levels are at their lowest. Finally, we show the long-term incorporation of this moonlight pattern into the ants’ path integration system throughout the night for homing, as polarised sunlight is incorporated throughout the day.