Disease: Deciphering the triad of infection, immunity and pathology
A fever, a cough, a splitting headache… Being sick often comes with tell-tale signs which worsen as the disease progresses and tissues become damaged. These symptoms result from complex interactions between the infecting pathogen, the inflammation process, and the response from the immune system. Tracking these mechanisms and how they interact, as well as identifying which factors determine when the disease recedes or progresses, is essential for establishing better treatment strategies.
In this effort, a more refined understanding of infection and immune responses has emerged from combining experimental and clinical measurements with mathematical models (Perelson, 2002). However, it is still difficult to link tissue pathology and disease severity with viral load or immune cell counts, which respectively measure the amount of virus and of certain immune actors in the body. Now, in eLife, Amber Smith and colleagues at St. Jude Children’s Research Hospital, the University of Tennessee Health Science Center and the Washington University School of Medicine – including Margaret Myers and Amanda Smith as joing first authors – report how viral infection, counteracting immune responses and lung pathology interact as mice fight off influenza A (Myers et al., 2021).
First, the team tracked how viral load and the number of CD8+ T cells, an important immune actor that helps to clear infected cells, progressed over time. In combination with mathematical models, these measurements allowed Myers et al. to estimate several parameters that reflect the pace at which the virus replicates, the strength of the immune response, and the interactions between these processes. While this had already been achieved in previous studies (e.g. Baccam et al., 2006), Myers et al. also analyzed the anatomy of the lung tissue over time, assessing the damage caused by infection and inflammation as well as how much the organ eventually regenerates.
Then, the team compared these data to values from their mathematical model that described viral load and CD8+ T cells counts, thereby linking viral load dynamics and specific immune responses to disease pathology and severity (Figure 1). In particular, the analysis shed light on how the relative number of immune cells correlates with the level of lung tissue cleared from the virus and, thus, the mice’s ability to recover from infection. These quantitative relationships could help to assess how well the virus is controlled within tissues simply by relying on easily accessible markers that are, for example, present in the blood. This would reduce the need for invasive tissue samples.
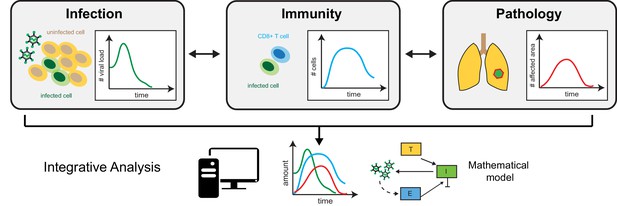
The triad of infection, immunity and disease pathology.
Separate data, such as viral load (left) – the quantity of virus present in a specific volume of fluid – immune cell counts (middle) and histological assessment of tissue sections (right) provide information on the dynamics of infection, immune responses and disease pathology. Mathematical modelling that integrates these measurements makes it possible to assess how the individual processes are connected, and to identify relevant prognostic markers that allow prediction of disease progression.
Individual molecular processes and specific aspects of viral replication can be studied extensively within in vitro cell culture systems. However, the full triad of infection, immunity and especially tissue pathology can only be reliably assessed within conditions that are physiologically relevant (Fackler et al., 2014). Indeed, simple cell culture systems insufficiently address the impact tissue structure can have on infection dynamics, immune activation and clearing mechanisms (Fackler et al., 2014; Imle et al., 2019).
Myers et al. used frequent samples and histological analyses to infer how infected tissues change over time. Yet, imaging technologies may continue to improve so that it becomes possible to observe the interactions between host and pathogen within tissues in real-time (Coombes and Robey, 2010). These approaches could help to investigate whether quantitative relationships as highlighted by Myers et al. also play a role in other infections and in other tissues. The expanding field of organoids – whereby simple, miniature organs are grown in the laboratory – also represents a promising step towards understanding how cells interact within structured, tissue-related environments (Gosselin et al., 2018; Bar-Ephraim et al., 2020). Combined with new technologies such as single-cell sequencing methods (Triana et al., 2021; Youk et al., 2020), these approaches will help to determine the molecular processes that govern disease progression, and how these might differ between patients.
Despite these new experimental and diagnostic technologies, data-driven mathematical modeling and analytical methods will continue to fulfil a key role for deciphering the interplay between infection, tissue pathology and disease severity. Using these models makes it possible to integrate different types of measurements from various places and times, and to disentangle the contributions of individual processes to the infection dynamics. It is only by understanding exactly how individual processes interact over time that scientists will be able to find and validate prognostic markers which predict disease progression.
References
-
Kinetics of influenza A virus infection in humansJournal of Virology 80:7590–7599.https://doi.org/10.1128/JVI.01623-05
-
Organoids in immunological researchNature Reviews Immunology 20:279–293.https://doi.org/10.1038/s41577-019-0248-y
-
Dynamic imaging of host-pathogen interactions in vivoNature Reviews Immunology 10:353–364.https://doi.org/10.1038/nri2746
-
Adding new dimensions: towards an integrative understanding of HIV-1 spreadNature Reviews Microbiology 12:563–574.https://doi.org/10.1038/nrmicro3309
-
Designing natural and synthetic immune tissuesNature Materials 17:484–498.https://doi.org/10.1038/s41563-018-0077-6
-
Modelling viral and immune system dynamicsNature Reviews Immunology 2:28–36.https://doi.org/10.1038/nri700
-
Single‐cell analyses reveal SARS‐CoV‐2 interference with intrinsic immune response in the human gutMolecular Systems Biology 17:e10232.https://doi.org/10.15252/msb.202110232
Article and author information
Author details
Publication history
Copyright
© 2021, Graw
This article is distributed under the terms of the Creative Commons Attribution License, which permits unrestricted use and redistribution provided that the original author and source are credited.
Metrics
-
- 798
- views
-
- 93
- downloads
-
- 3
- citations
Views, downloads and citations are aggregated across all versions of this paper published by eLife.
Download links
Downloads (link to download the article as PDF)
Open citations (links to open the citations from this article in various online reference manager services)
Cite this article (links to download the citations from this article in formats compatible with various reference manager tools)
Further reading
-
- Cancer Biology
- Computational and Systems Biology
Effects from aging in single cells are heterogenous, whereas at the organ- and tissue-levels aging phenotypes tend to appear as stereotypical changes. The mammary epithelium is a bilayer of two major phenotypically and functionally distinct cell lineages: luminal epithelial and myoepithelial cells. Mammary luminal epithelia exhibit substantial stereotypical changes with age that merit attention because these cells are the putative cells-of-origin for breast cancers. We hypothesize that effects from aging that impinge upon maintenance of lineage fidelity increase susceptibility to cancer initiation. We generated and analyzed transcriptomes from primary luminal epithelial and myoepithelial cells from younger <30 (y)ears old and older >55y women. In addition to age-dependent directional changes in gene expression, we observed increased transcriptional variance with age that contributed to genome-wide loss of lineage fidelity. Age-dependent variant responses were common to both lineages, whereas directional changes were almost exclusively detected in luminal epithelia and involved altered regulation of chromatin and genome organizers such as SATB1. Epithelial expression of gap junction protein GJB6 increased with age, and modulation of GJB6 expression in heterochronous co-cultures revealed that it provided a communication conduit from myoepithelial cells that drove directional change in luminal cells. Age-dependent luminal transcriptomes comprised a prominent signal that could be detected in bulk tissue during aging and transition into cancers. A machine learning classifier based on luminal-specific aging distinguished normal from cancer tissue and was highly predictive of breast cancer subtype. We speculate that luminal epithelia are the ultimate site of integration of the variant responses to aging in their surrounding tissue, and that their emergent phenotype both endows cells with the ability to become cancer-cells-of-origin and represents a biosensor that presages cancer susceptibility.
-
- Computational and Systems Biology
- Microbiology and Infectious Disease
Timely and effective use of antimicrobial drugs can improve patient outcomes, as well as help safeguard against resistance development. Matrix-assisted laser desorption/ionization time-of-flight mass spectrometry (MALDI-TOF MS) is currently routinely used in clinical diagnostics for rapid species identification. Mining additional data from said spectra in the form of antimicrobial resistance (AMR) profiles is, therefore, highly promising. Such AMR profiles could serve as a drop-in solution for drastically improving treatment efficiency, effectiveness, and costs. This study endeavors to develop the first machine learning models capable of predicting AMR profiles for the whole repertoire of species and drugs encountered in clinical microbiology. The resulting models can be interpreted as drug recommender systems for infectious diseases. We find that our dual-branch method delivers considerably higher performance compared to previous approaches. In addition, experiments show that the models can be efficiently fine-tuned to data from other clinical laboratories. MALDI-TOF-based AMR recommender systems can, hence, greatly extend the value of MALDI-TOF MS for clinical diagnostics. All code supporting this study is distributed on PyPI and is packaged at https://github.com/gdewael/maldi-nn.