Colicin E1 opens its hinge to plug TolC
Figures
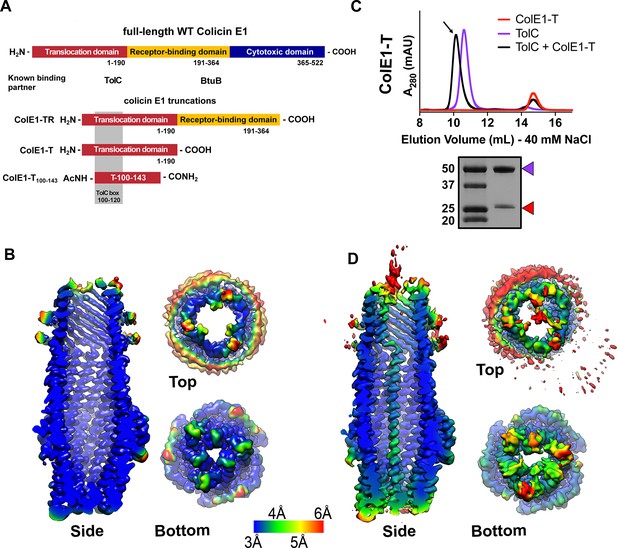
Colicin E1-T binds within the lumen of TolC.
(A) Architecture of full-length colicin E1 showing domains and their known binding partners. Three truncation constructs used in this study. (B) CryoEM structure of TolC embedded in nanodiscs. Side, top, and bottom views are colored by local resolution, as computed in cryoSPARC from the final half-maps. The side view is cropped to display the particle interior. (C) SEC chromatogram of ColE1-T (red line) and TolC (purple line). The arrow indicates the co-elution (black line) fractions (eluted in buffer containing 40 mM NaCl) that were analyzed by SDS-PAGE. On the SDS-PAGE gel (bottom), red arrows indicate the presence of the colicin E1 construct that has co-eluted with TolC. SEC chromatogram of TolC purification (Figure 1—figure supplement 1) and co-elution with 200 mM NaCl (Figure 1—figure supplement 2). (D) The CryoEM structure of ColE1-T bound to TolC and colored by local resolution as in (B). CryoEM data collection, refinement, and validation statistics in Figure 1—source data 1. cryoEM, cryo-electron microscopy; SEC, size exclusion chromatography.
-
Figure 1—source data 1
CryoEM data collection, refinement, and validation statistics.
- https://cdn.elifesciences.org/articles/73297/elife-73297-fig1-data1-v2.xlsx
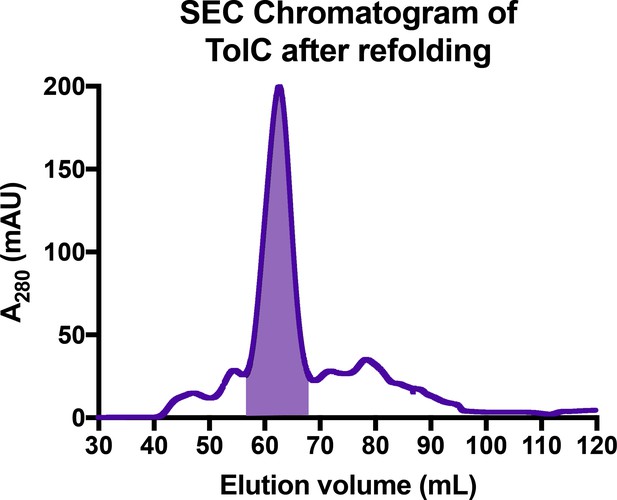
Sample SEC chromatogram of TolC purification after refolding.
Elution volumes 57–68 ml (shaded) were pooled for further experimentation with TolC. Refolded TolC applied onto a HiLoad 16/60 Superdex 200 pg column on an ÄKTA Pure FPLC system, and eluted with 1.5 column volumes in 20 mM Tris pH 8.0, 100 mM NaCl, and 0.05% n-dodecyl-β-D-maltoside. SEC, size exclusion chromatography.
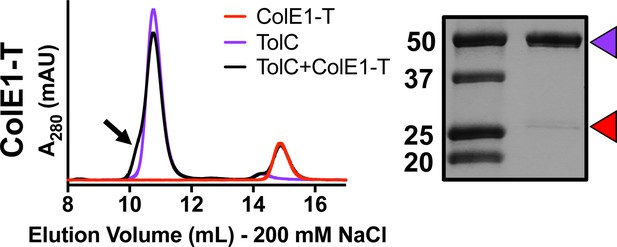
Co-elution of ColE1-T with TolC at 200 mM NaCl (black).
Under this higher salt concentration, when TolC (purple) and ColE1-T (red) are mixed, there is a smaller peak shift than that seen with ColE1-TR and the presence of a shoulder (black arrow). SDS-PAGE of the shoulder shows the presence of both TolC (purple arrow) and ColE1-T (red arrow). Although some binding was detected, this higher salt concentration prevents full binding as indicated by a much fainter band for ColE1-T than seen at the lower salt concentration (Figure 4A).
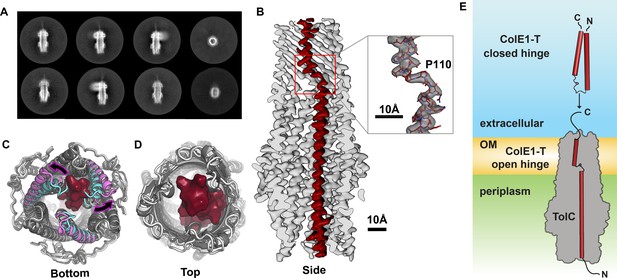
Colicin E1 binds to TolC as a single-pass kinked helix.
(A) 2D class averages of ColE1-T bound to TolC embedded in nanodiscs. (B) Cutaway side view of the TolC (light gray) interior. ColE1-T (red) binds asymmetrically in an open-hinge conformation. The hinge region of ColE1-T including residue P110 shown in stick representation (inset). (C, D) The apo cryoEM structure of TolC (light gray) compared to holo cryoEM structure (dark gray). (C) Periplasmic aperture. Two of the TolC helices in the bound structure (magenta) dilate outwards compared to those two helices in the unbound state (cyan). (D) The extracellular aperture remains more similar between the bound and unbound structures. (E) In the unbound state, ColE1-T (red cylinders) exists as a closed hinge. When bound, ColE1-T is in an open-hinge conformation through TolC with the N-terminus into the pore of TolC (gray). The side chain of the hinge proline is shown making a small pentagon in both the closed and open hinge states. The model of ColE1-T in its solution state was built using AlphaFold (Jumper et al., 2021) and is consistent with previous experiments (Zakharov et al., 2016). cryoEM, cryo-electron microscopy.
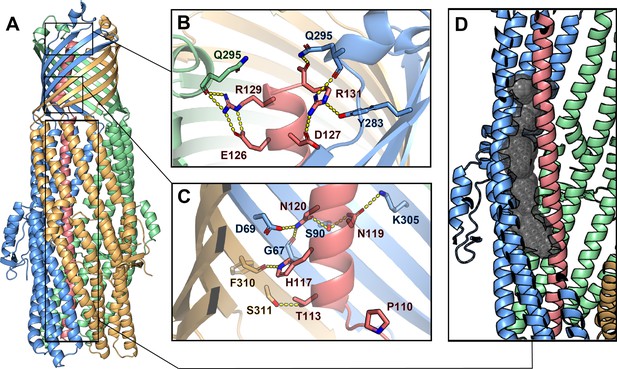
Inter-chain contacts between ColE1 T and TolC.
(A) Molecular dynamics simulation refined structure of the ColE1-T:TolC complex colored by chain (ColE1-T: light red, TolC: light blue, light green, light gold). (B, C) Polar interaction network of ColE1-T and TolC on the C-terminal side of the proline kink in the β-barrel region. Locations of previous mutagenesis data near the TolC box (Figure 3—figure supplement 1 and Figure 3—video 1). (B) Near the extracellular opening of TolC and (C) in the TolC barrel near the transition to the TolC periplasmic helical region. Residues involved in forming polar interactions and proline 110 are shown in sticks. These interactions support binding such that even when the hinge proline is mutated to alanine (P110A) binding is maintained (Figure 3—figure supplement 2). (D) ColE1-T spanning the TolC helical barrel does not make full contact with the side of the TolC barrel. The cavity (black spheres) between colicin (light red) and one chain of TolC (light blue) was detected using GHECOM (Kawabata, 2010; Kawabata, 2019; Kawabata and Go, 2007).
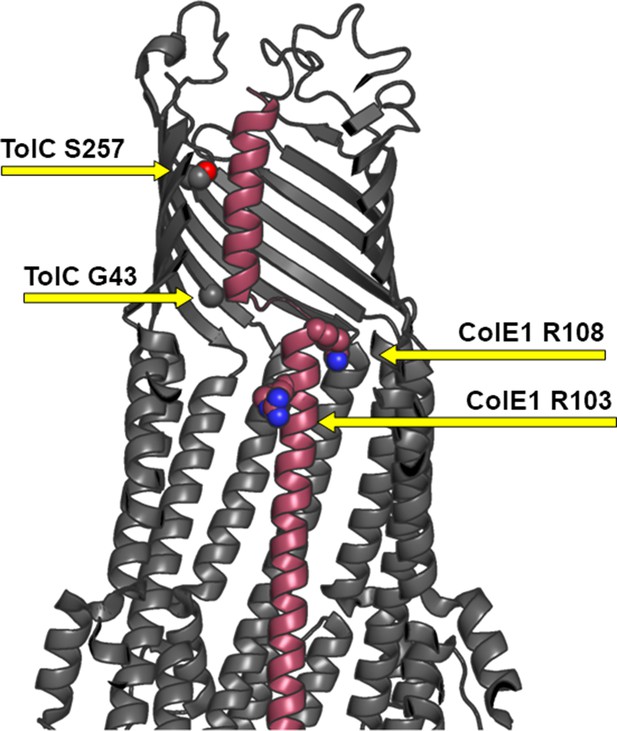
Cut away side-view of TolC:ColE1-T complex.
Mutations at positions G43 and S257 (spheres) in the TolC barrel (gray) make direct contact with ColE1-T (pink). TolC Mutations to bulkier side chains G43D and S257P were previously shown to abolish WT ColE1 function (Masi et al., 2007) likely due to the introduction of steric clashes that prevent binding. Mutations to ColE1 side chains R108 and R103 (spheres) to glutamine do not abrogate its activity (Jakes, 2017).
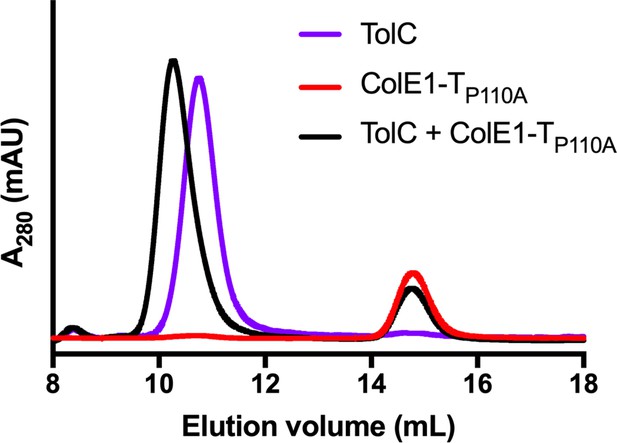
ColE1-TP110A retains binding to TolC.
SEC chromatogram of ColE1-TP110A (red line) and TolC (purple line). The arrow indicates the co-elution (black line) fractions (eluted in buffer containing 40 mM NaCl).
Pan through the atomic model of the TolC/colE1-T complex, from the extracellular aperture to the periplasmic aperture.
Carbon atoms of the three TolC polypeptides are colored three different shades of gray and carbon atoms of the colE1-T polypeptide are colored maroon, colE1-T residues of note are colored purple and labeled; field-standard coloring is used for heteroatoms.
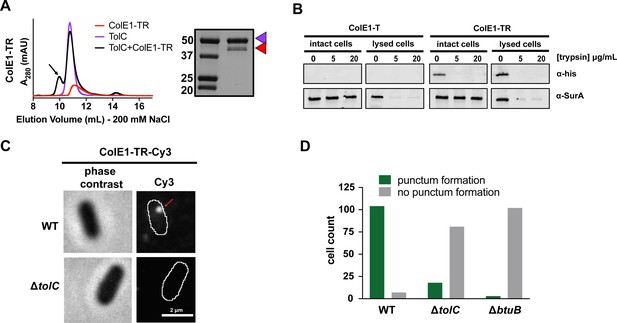
Colicin E1-TR localizes on the outside of the cell.
(A) SEC chromatogram of ColE1-TR (red line) and TolC (purple line). The arrow indicates the co-elution (black line) fractions that were analyzed by SDS-PAGE. On the SDS-PAGE gels (right), red arrow indicates the presence of colicin E1 construct that has co-eluted with TolC (purple arrow). (B) Extracellular protease digestion assay with two colicin E1 truncation constructs incubated with Escherichia coli, each construct is labeled with a C-terminal His-Tag. Periplasmic SurA was used as a membrane integrity control. After incubation with colicin fragments, cells were left intact or lysed with lysozyme. Proteins degraded by trypsin in intact cells are not protected by the outer membrane. (C) Fluorescence image (right) of ColE1-TR-Cy3 overlaid on outlines of living E. coli cells from phase-contrast microscopy (left) for WT and ΔtolC. Red arrow points to a punctum. A larger variety of images is also available (Figure 4—figure supplement 1). Similar localization was seen for ColE1-TR-GFP (Figure 4—figure supplement 2) demonstrating that the effect was not caused by the fluorophore, and ColE1-TRΔ1–40-Cy3 (Figure 4—source data 1, Figure 4—figure supplement 3) demonstrating that the effect is not TolA dependent. The puncta were stable and were found to remain intact for more than 5 min. The punctum shown here remained intact for at least 30 s (Figure 4—video 1). (D) Cell counts where ColE1-TR-Cy3 punctum formation was observed for WT, ΔtolC, and ΔbtuB. Number of cells observed, n=111, 91, 105, respectively. SEC, size exclusion chromatography.
-
Figure 4—source data 1
Comparison of percent cells with puncta and average number of puncta per cell between ColE1-TR-Cy3 and ColE1-TRΔ1–40-Cy3 with WT ΔtolC and ΔbtuB.
- https://cdn.elifesciences.org/articles/73297/elife-73297-fig4-data1-v2.xlsx
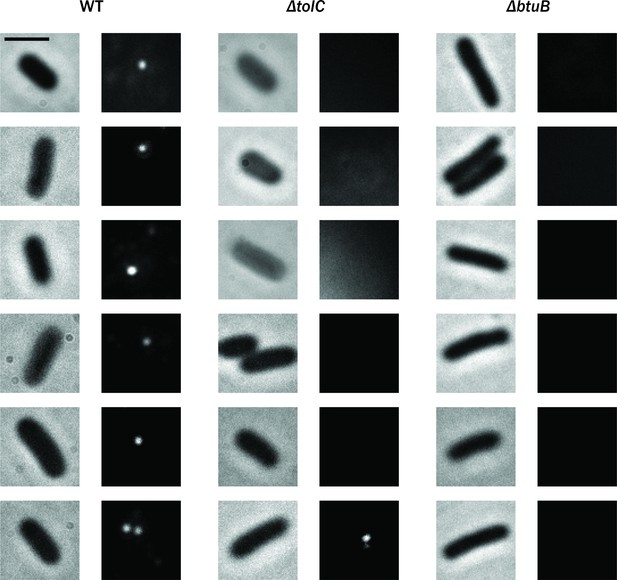
Additional examples of cells observed during imaging described in Figure 4C and D.
Brightfield phase-contrast images of living Escherichia coli cells (left) and fluorescence images (right) of ColE1-TR-Cy3 for WT, ΔtolC, and ΔbtuB. Scale bar: 2 µm.
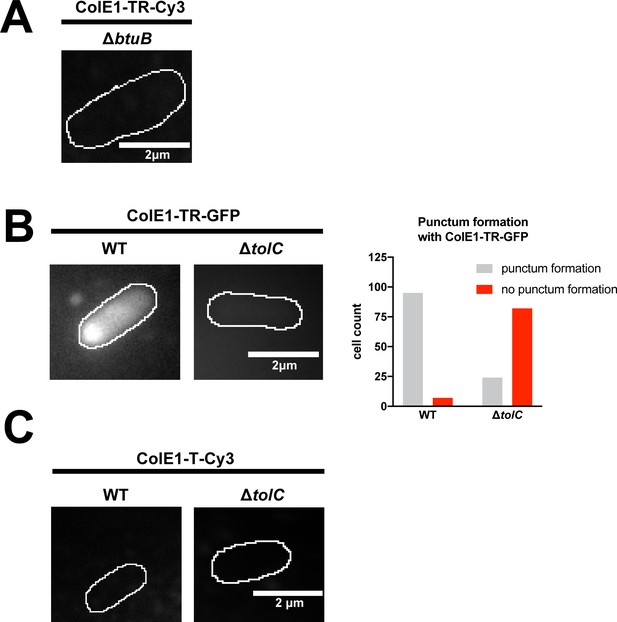
Single-molecule microscopy.
Fluorescence images overlaid on outlines of living Escherichia coli cells from phase-contrast microscopy for WT and ΔtolC for ColE1-TR-GFP and counts of cells where ColE1-TR-GFP punctum formation was observed. (A) 97% of cells showed no binding of Cy3-labeled ColE1-TR to ΔbtuB (B) ColE1-TR-GFP forms similar puncta as Cy3-labeled ColE1-TR (main text Figure 4C). (C) No binding of Cy3-labeled ColE1-T to WT or ΔtolC cells was detected with Cy3-labeled ColE1-T.
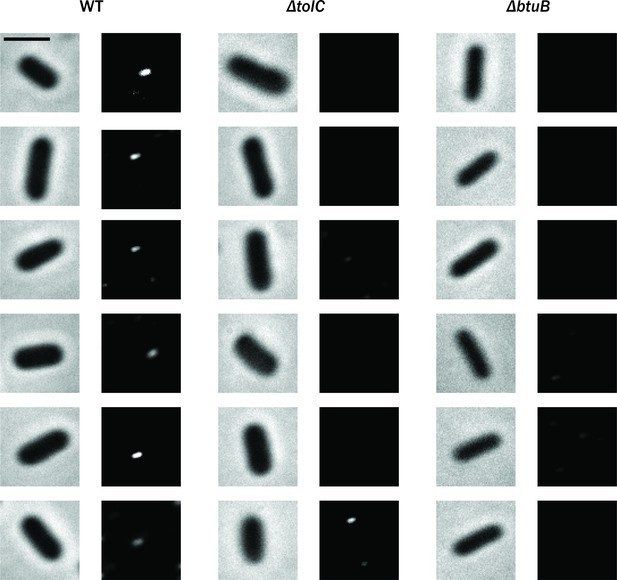
Examples of cells observed with ColE1-TRΔ1–40-Cy3.
Brightfield phase-contrast images of living Escherichia coli cells (left) and fluorescence images of ColE1-TR-Cy3 for WT, ΔtolC, and ΔbtuB. Scale bar: 2 µm.
A representative 30-s movie of ColE1-TR bound to a live cell.
ColE1-TR localizes on, and remains bound to, the extracellular surface of Escherichia coli. Fluorescence movie of Cy3-labeled ColE1-TR on living WT E. coli overlaid on outline of the E. coli cell from phase-contrast microscopy. Continuous imaging at 25 frames per second; the movie plays in real time at the same speed. This movie corresponds to the first 30 s of data used to attain the WT image in Figure 4C. Scale bar: 2 µm.
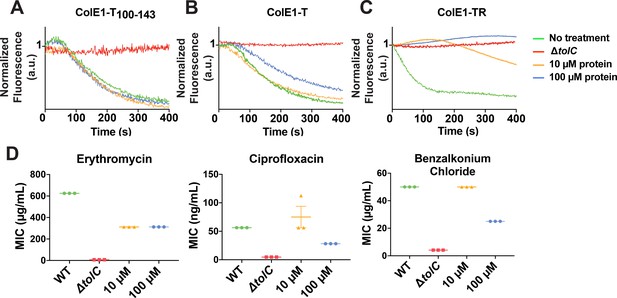
Colicin E1 fragments inhibit efflux and potentiate antibiotics.
(A–C) Effect of colicin E1 fragments on efflux: WT with no protein (green), ΔtolC (red), WT + 10 μM (orange), and WT + 100 μM protein (blue). (A) ColE1-T100–143, (B) ColE1-T, and (C) ColE1-TR. All efflux experiments used 10 μM of fluorescent reporter dye NNN. (D) Antibiotic susceptibilities in the absence (green) and presence of ColE1-TR added to WT at 10 μM (orange) and 100 μM (blue). MICs for ΔtolC (red) are included as a reference. Data shown from three biological replicates reported as the mean and individual data points. Bars indicate standard error of the mean. Data values shown in Figure 5—source data 1. MIC, minimum inhibitory concentration.
-
Figure 5—source data 1
Mean minimum inhibitory concentrations (MICs) of antimicrobials in the presence of ColE1-TR.
- https://cdn.elifesciences.org/articles/73297/elife-73297-fig5-data1-v2.xlsx
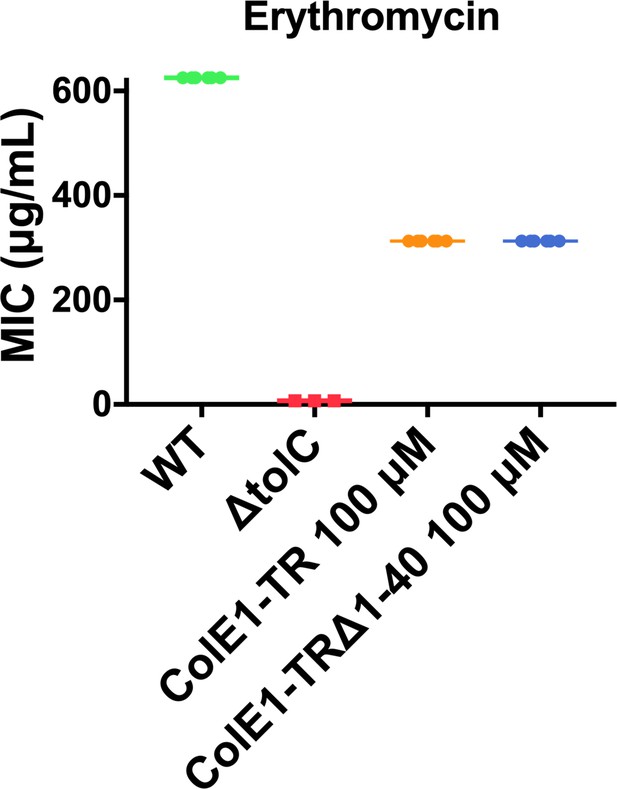
Minimum inhibitory concentration (MIC) of erythromycin with ColE1-TR TolA box deletion construct.
The MIC for erythromycin with 100 μM ColE1-TR or ColE1-TRΔ1–40 is identical indicating that TolA engagement by ColE1-TR does not contribute to antibiotic susceptibility.
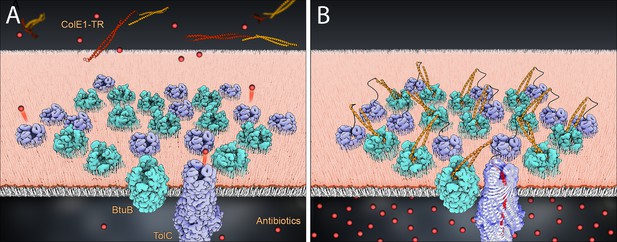
Model of colE1-TR inhibition of efflux.
The R domain of ColE1-TR (orange) binds to BtuB (cyan) with high affinity and anchors colE1-TR to the surface of the cell. The T domain (red) then inserts into TolC (lavender), plugging the channel and blocking exit of antibiotics (spheres). We only see this interaction when ~20 colE1-TR bind in the same cluster though for simplicity only 12 BtuB and 12 TolC proteins are pictured here. (A) Before binding, antibiotics are effluxed. (B) After binding the colicin E1 fragments prevent some antibiotic efflux.
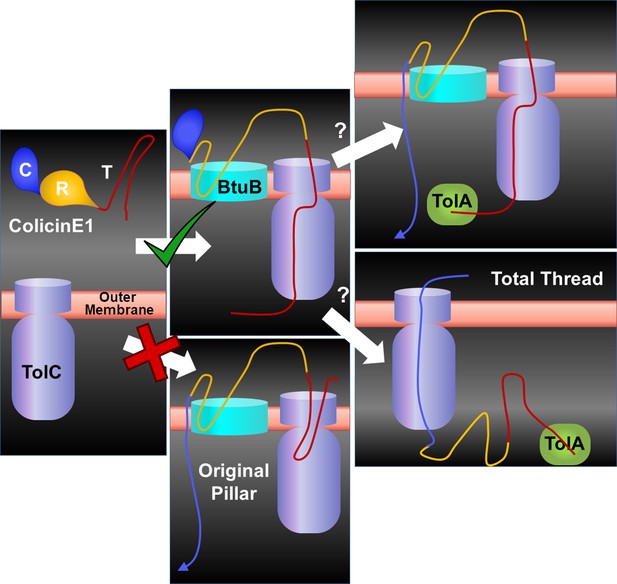
ColE1 TR sticks to the surface of Escherichia coli but the mechanism of C domain insertion is unclear.
The binding between ColE1-R to BtuB and ColE1-T to TolC are likely similar regardless of the presence of the ColE1 C domain. Our work excludes the possibility of the original pillar model. However, because our work did not utilize the C domain, we cannot distinguish between a modified version of the pillar model and the total thread model.
Tables
Reagent type (species) or resource | Designation | Source or reference | Identifiers | Additional information |
---|---|---|---|---|
Antibody | THE HisTag mAb Mouse (mouse monoclonal) | GenScript | Cat#A00186-100; RRID:19J001957 | (1:2000) |
Antibody | Anti-SurA Rabbit polyclonal (rabbit polyclonal) | Cusabio | Cat#CSB-PA359693HA01ENV; RRID:E0109A | (1:2500) |
Antibody | IRDye 800CW goat anti-mouse IgG secondary (goat polyclonal) | LI-COR | Cat#925-32210; RRID:C81106-01 | (1:10,000) |
Antibody | IRDye 800CW Donkey anti-Rabbit (donkey monoclonal) | LI-COR | Cat#925-32213; RRID:C80829-10 | (1:10,000) |
Cell line (Escherichia coli) | BW25113 | Coli Genetic Stock Center | Cat#7636 | |
Cell line (E. coli) | JW5503-1 | Coli Genetic Stock Center | Cat#11430 | |
Cell line (E. coli) | BL21(DE3) | Coli Genetic Stock Center | Cat#12504 | |
Recombinant DNA reagent | pTrc99a-NoSS-TolC | This paper | Addgene: 155179 | Addgene: 155179 |
Recombinant DNA reagent | pET303-colE1-T | This paper | Addgene: 155180 | Addgene: 155180 |
Recombinant DNA reagent | pET303-colE1-TR | This paper | Addgene: 155181 | Addgene: 155181 |
Recombinant DNA reagent | pET303-colE1-T-E192C | This paper | Addgene: 180233 | Addgene: 180233 |
Recombinant DNA reagent | pET303-colE1-TR-E366C | This paper | Addgene: 180234 | Addgene: 180234 |
Recombinant DNA reagent | pET303-colE1-TR-E366C-∆1–40 | This paper | Addgene: 180235 | Addgene: 180235 |
Recombinant DNA reagent | pET303-colE1-TR-∆1–40 | This paper | Addgene: 180236 | Addgene: 180236 |
Recombinant DNA reagent | pET303-colE1-T-P110A | This paper | Addgene: 180237 | Addgene: 180237 |
Recombinant DNA reagent | MSP1D1 | Addgene | Addgene: 20061 | |
Software, algorithm | cryoSPARC 2v2.12 | Punjani et al., 2017 | https://cryosparc.com/ | |
Software, algorithm | phenix v1.14-3260 | Afonine et al., 2018 | https://www.phenix-online.org/ | |
Software, algorithm | serialEM v3.7 | Mastronarde, 2018 | https://bio3d.colorado.edu/SerialEM/ | |
Software, algorithm | coot v0.8.9.2-re 7766 | Emsley et al., 2010 | https://www2.mrc-lmb.cam.ac.uk/personal/pemsley/coot/ | |
Software, algorithm | ISOLDE v1.0b4.dev1 | Croll, 2018 | https://isolde.cimr.cam.ac.uk/static/isolde/doc/tools/ISOLDE.html | |
Software, algorithm | UCSF ChimeraX | Goddard et al., 2018 | https://www.cgl.ucsf.edu/chimerax/ | |
Software, algorithm | PyMOL | Schrödinger, LLC | https://pymol.org/2/ |