Circadian programming of the ellipsoid body sleep homeostat in Drosophila
Figures
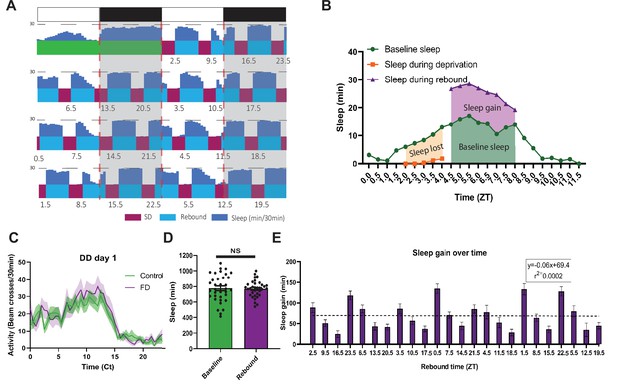
Drosophila forced desynchrony protocol can be used to illustrate time dependent rebound (A) Average WT sleep (N=32) over the final 8 days of SSD protocol with the time at which rebound begins (ZT) noted below each rebound period.
(B) Profiles of sleep metrics used to compare rebound at different times of day (example is rebound occurring at ZT4.5). Sleep lost is determined by the difference between baseline sleep and sleep during the SD. Sleep gain is determined by the difference between rebound and baseline sleep. (C) Average activity of WT flies over 24 hr of flies released into the dark following SSD stimulation (N=19) or control (N=19) that received no stimulation. WT Flies released into DD1 following SSD display a profile of activity similar to control flies. Shaded bands indicate SEM.(D) Average sleep during baseline and the average sleep per day during the 7-day SD-rebound period (individual flies shown circles). There is no significant difference between average baseline sleep and average sleep per day over the course of the SSD (p>0.08, paired t-test). (E) Average WT (N=32) sleep gain across the course of the experiment with rebound time (ZT) depicted on the x axis. Regression of WT sleep gain over the course of the experiment displays no significant trend (p>0.95 linear regression). Data are means +/- SEM.
-
Figure 1—source data 1
T7 Drosophila forced desynchrony protocol can be used to illustrate time dependent rebound.
- https://cdn.elifesciences.org/articles/74327/elife-74327-fig1-data1-v2.xlsx
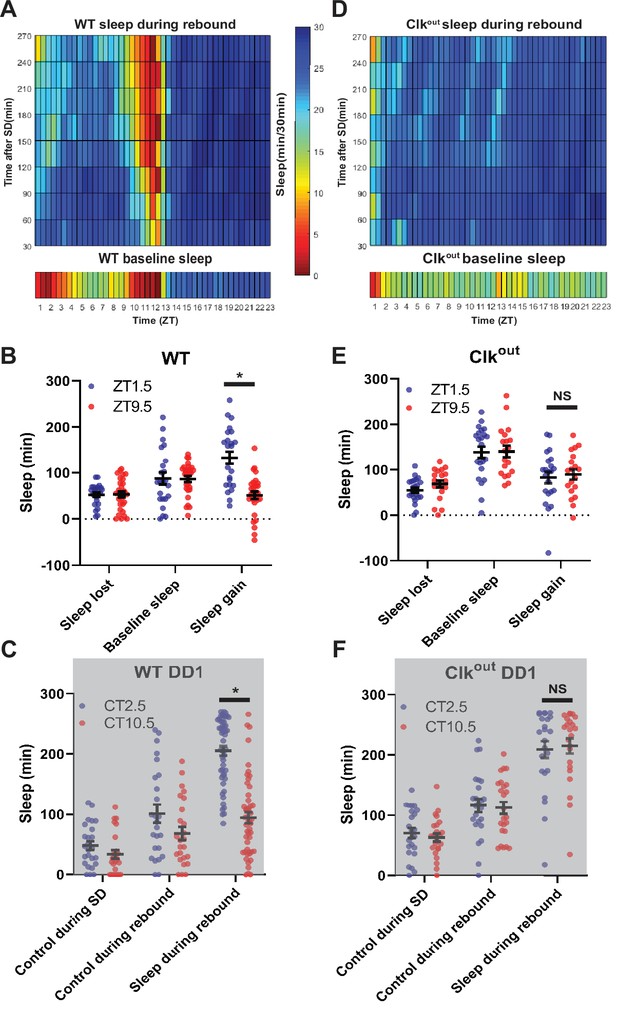
Sleep rebound is dependent on the molecular clock(A,D) Rebound sleep heatmaps (above) illustrate average sleep as a function of time of day when rebound occurred (ZT) and minutes after SSD episode.
Missing time points are filled using matlab linear interpolation function. Baseline sleep heatmaps (below) illustrate average sleep during 30 min bins. (A) WT (N=32) baseline displays low sleep following lights on and preceding lights off. Immediately following SD flies show high sleep except in the hours preceding lights off. Flies tend to sleep less as rebound time proceeds. (B,E) Comparison of sleep lost, baseline sleep, and sleep gain following deprivation at morning and evening timepoints. (B) Sleep gain is greater for WT (N=32) rebound at ZT1.5 compared to ZT9.5 (p<0.00001, paired t-test). (C,F) Two sleep measures in control flies (control during SD and control during rebound), along with sleep during rebound in SD with rebound at 2.5 and 10.5. (C) Rebound sleep is greater following deprivation at CT2.5 compared to CT10.5 (p<0.00001, paired t-test) in WT flies (N=49). (D) Clkout (N=40) baseline sleep (below) is nearly constant except for low sleep immediately following lights on. SD uniformly increases sleep and flies tend to sleep less as rebound time proceeds. (E) No difference between sleep gain at the two time points is observed in Clkout (N=40) (p>0.37, paired t-test). (F) No difference in rebound sleep is observed in Clkout (N=23) (p>0.75, paired t-test). Data are means +/- SEM.
-
Figure 2—source data 1
Sleep rebound is dependent on the molecular clock.
- https://cdn.elifesciences.org/articles/74327/elife-74327-fig2-data1-v2.xlsx
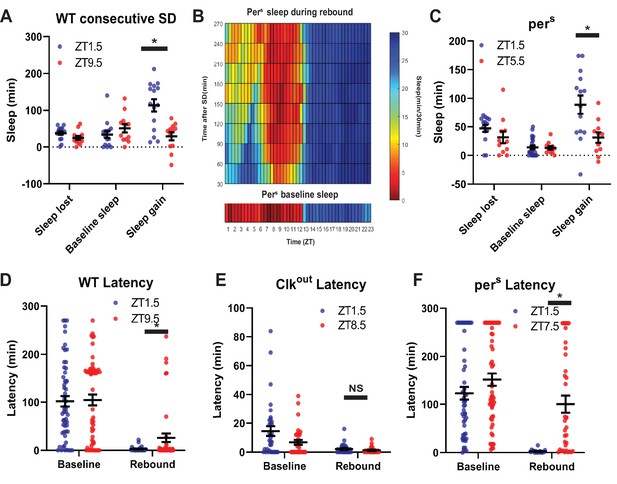
Total sleep and sleep latency vary as a function of time and SD (A) Comparison of sleep lost, baseline sleep, and sleep gain at morning (ZT1.5) and evening (ZT9.5) time points using abridged protocol with WT flies.
Sleep gain is greater for WT (N=18) at ZT1.5 compared to ZT9.5 (p<0.001, paired t-test). (B) Rebound sleep heatmap (above) illustrates average sleep as a function of time of day when rebound occurred (ZT) and minutes after SSD episode. Missing time points are filled using matlab linear interpolation function. Baseline sleep heatmaps (below) illustrate average sleep during 30 min bins. pers (N=45) baseline sleep displays low sleep following lights on and preceding lights off. Immediately following SD flies show increased sleep except in the hours preceding lights off. Flies tend to sleep less as rebound time proceeds. (C) FD sleep during two baseline time periods (sleep lost and baseline sleep) and sleep gain for rebound occurring at ZT1.5 and ZT5.5. Sleep gain is greater for pers (N=45) rebound at ZT1.5 compared to ZT5.5 (P<.01, paired t-test). (D–F) Morning and evening sleep latency at baseline and following deprivation for WT and circadian mutant flies. Morning times are matched with evening time points with similar baseline latency. (D) Following SD, WT (N=32) sleep latency is greater in the evening compared to matched morning time point (p<0.05, paired t-test). (E) No difference in sleep latency following SD between matched morning/evening time points in Clkout (N=40) (p>0.50, paired t-test). (F) Following SD, pers (N=45) sleep latency is greater in the evening compared to matched morning time point (p<0.0001, paired t-test). Data are means +/- SEM.
-
Figure 2—figure supplement 1—source data 1
Total sleep and sleep latency vary as a function of time and SD.
- https://cdn.elifesciences.org/articles/74327/elife-74327-fig2-figsupp1-data1-v2.xlsx
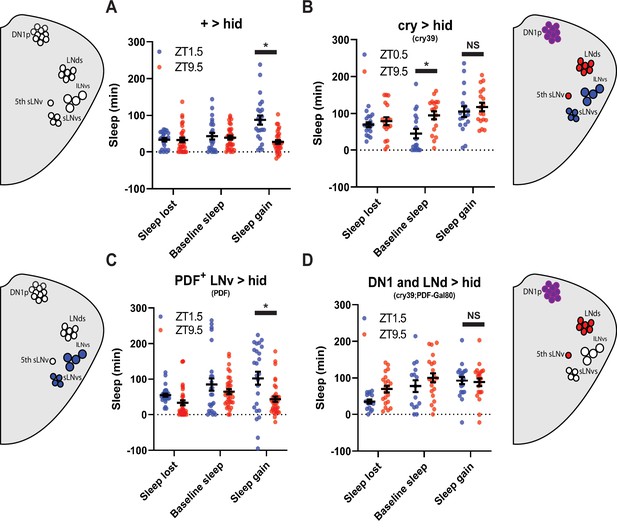
PDF+ neurons do not mediate morning/evening differences in rebound (A,B,C,D) Comparison of sleep lost, baseline sleep, and sleep gain following deprivation at morning and evening timepoints in clock neuron-ablated flies.
Morning times are matched with evening time points with similar baselines. (A) Control flies with no ablated neurons (+>hid) (N=27) exhibit greater rebound in the morning compared to matched evening time point (p<0.0001, paired t-test). (B) Flies with most clock neurons ablated (cry39 >hid) (N=19) exhibit no difference in sleep gain between matched morning/evening time points (p>0.70, paired t-test). (C) Files with PDF+ neurons ablated (pdf >hid) (N=35) exhibit greater rebound in the morning compared to a matched evening time point (p<0.01, paired t-test). (D) Flies with most clock neurons ablated except PDF+ neurons (cry39; pdf-Gal80 >hid) (N=22) exhibit no significant difference in sleep gain between matched morning/evening time points (p>0.97, paired t-test). Data are means +/- SEM.
-
Figure 3—source data 1
PDF+ neurons do not mediate morning/evening differences in rebound.
- https://cdn.elifesciences.org/articles/74327/elife-74327-fig3-data1-v2.xlsx
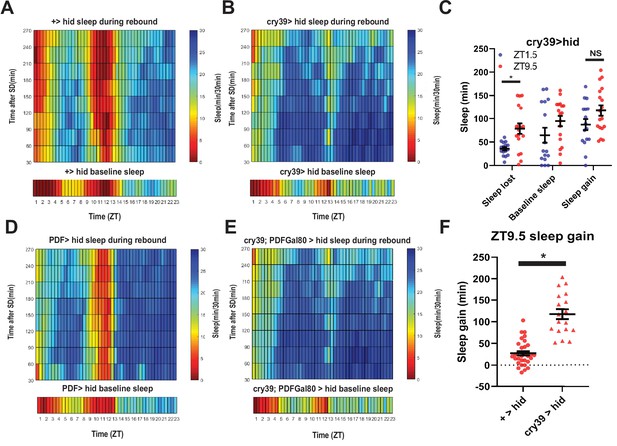
Non PDF neurons drive morning/evening differences in rebound(A,B,D,E) Rebound sleep heatmaps (above) illustrate average sleep as a function of time of day when rebound occurred (ZT) and minutes after SSD episode.
Missing time points are filled using matlab linear interpolation function. Baseline sleep heatmaps (below) illustrate average sleep during 30 min bins. (A) Control flies with no ablated neurons (+>hid) (N=27) displays low baseline sleep following lights on and preceding lights off. Immediately following SD flies show high sleep except in the hours preceding lights off. Flies tend to sleep less as rebound time proceeds. (B) Flies with most clock neurons ablated (cry39 >hid) (N=19) displays low baseline sleep during the day and high sleep at night (below). SD uniformly increases sleep and flies tend to sleep less as rebound time proceeds and following lights on (C) Comparison of sleep lost, baseline sleep, and sleep gain following deprivation at morning and evening timepoints in clock neuron-ablated flies. Morning times are matched with evening time points with similar baselines. Flies with most clock neurons ablated (cry39 >hid) (N=19) do not exhibit a significant difference in sleep gain between the morning and evening time points (P>0.01, paired t-test) however exhibit greater sleep lost in the evening (p<0.05, paired t-test). (D) Files with PDF+ neurons ablated (pdf >hid) (N=35) displays low baseline sleep following lights on and preceding lights off. Immediately following SD flies show high sleep except in the hours preceding lights off. (E) Flies with most clock neurons ablated except PDF+ neurons (cry39; pdf-Gal80 >hid) (N=22) display low baseline sleep during the day and high sleep at night (below). SD uniformly increases sleep and flies tend to sleep less as rebound time proceeds and following lights on. (F) Comparison of sleep gain at ZT9.5 between control flies and flies with most clock neurons ablated. clock neuron ablated flies exhibit significantly greater rebound at ZT9.5 compared to controls (p<0.0001, unpaired t-test).
-
Figure 3—figure supplement 1—source data 1
Non PDF neurons drive morning/evening differences in rebound.
- https://cdn.elifesciences.org/articles/74327/elife-74327-fig3-figsupp1-data1-v2.xlsx
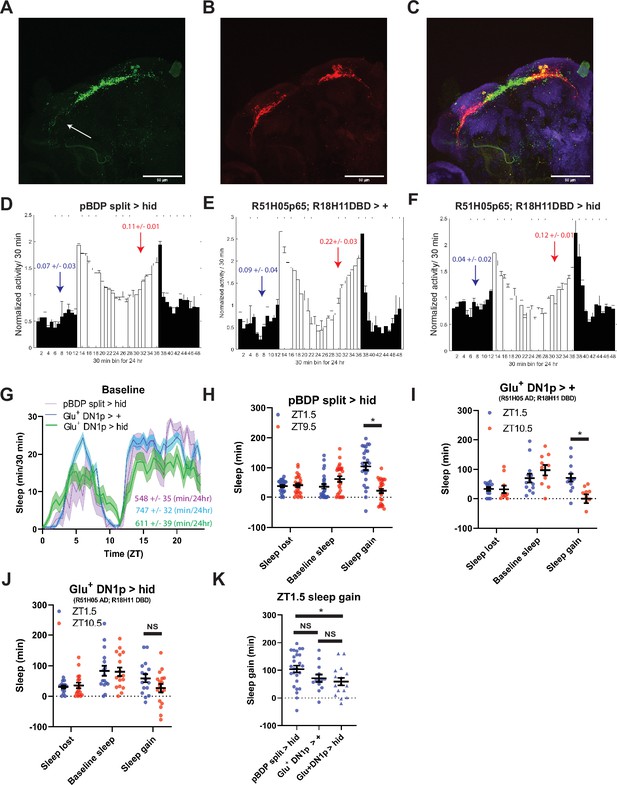
Glutamatergic DN1ps are necessary for morning and evening differences in rebound.
(A-C) 20 x images of split Gal4 line that labeling presynaptic (A), postynaptic (B) and overlay (C) of Glu+ DN1ps (R51H05 AD; R18H11 DBD >SYT GFP; DenMark) co-stained for BRP (blue). (D–F) Averaged activity eductions for female flies during the first 2 days of 12:12 LD. The light-phase is indicated by white bars while the dark-phase is indicated by black bars. Morning and evening anticipation indices are represented in blue and red respectively. (G) Average sleep during the baseline day for Glu+ DN1ps ablated (R51H05 AD; R18H11 DBD >hid) (N=30) (green), Gal4 control (R51H05 AD; R18H11 DBD> +) (N=36) and hid control (pBDP split >hid) (N=26) (purple). Sleep per 24 hr is indicated in the bottom right. (H–J) Comparison of sleep lost, baseline sleep, and sleep gain following deprivation at morning and evening timepoints in Glu+ DN1p ablated flies. Morning times are matched with evening time points with similar baselines. (H) hid control flies with no ablated neurons (pBDP split >hid) (N=26) exhibit greater rebound in the morning compared to matched evening time point (p<0.0001, paired t-test). (I) Gal4 control flies with no ablated neurons (R51H05 AD; R18H11 DBD> +) (N=19) exhibit greater rebound in the morning compared to matched evening time point (p<0.01, paired t-test) (J) Flies with Glu+ DN1ps ablated (R51H05 AD; R18H11 DBD >hid) (N=21) do not exhibit a significant difference in sleep gain between matched morning/evening time points (p>0.09, paired t-test). (K) Comparison of sleep gain at ZT1.5 between flies with Flies with Glu+ DN1ps ablated (R51H05 AD; R18H11 DBD >hid) (N=21) and their controls (pBDP split >hid) (N=26) and (R51H05 AD; R18H11 DBD> +) (N=19). R51H05 AD; R18H11 DBD >hid flies exhibit significantly less rebound at ZT1.5 compared to hid control (p<0.05, ANOVA) and a non-significant decrease compared to Gal4 control (p>0.05, ANOVA). Data are means +/- SEM.
-
Figure 4—source data 1
Glutamatergic DN1ps are necessary for morning and evening differences in rebound.
- https://cdn.elifesciences.org/articles/74327/elife-74327-fig4-data1-v2.xlsx
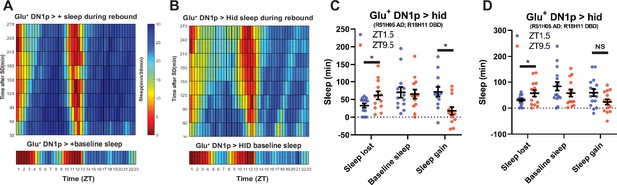
Standardized time points show similar effects to points with matched baseline sleep (A–B) Rebound sleep heatmaps (above) illustrate average sleep as a function of time of day when rebound occurred (ZT) and minutes after SSD episode.
Missing time points are filled using matlab linear interpolation function. Baseline sleep heatmaps (below) illustrate average sleep during 30 min bins. (A) Gal4 controls with no ablated neurons (R51H05 AD; R18H11 DBD> +) (N=19) display low baseline sleep following lights on and preceding lights off. Immediately following SD flies show high sleep except in the hours preceding lights off. Flies tend to sleep less as rebound time proceeds. (B) Glu+ DN1ps ablated (R51H05 AD; R18H11 DBD >hid) (N=14) flies display low baseline sleep following lights on and preceding lights off. Immediately following SD flies show high sleep except in the afternoon and hours preceding lights off. Flies tend to sleep less as rebound time proceeds. (C,D) Comparison of sleep lost, baseline sleep, and sleep gain following deprivation at morning and evening timepoints in clock neuron-ablated flies. Morning times are matched with evening time points with similar baselines. (C) Gal4 control flies with no ablated neurons (R51H05 AD; R18H11 DBD> +) (N=19) exhibit greater rebound in the morning compared to the evening time point (p<0.01, paired t-test) however exhibit greater sleep lost in the evening (p<0.05). (D) Flies with Glu+ DN1ps ablated (R51H05 AD; R18H11 DBD >hid) (N=14) do not exhibit a significant difference in sleep gain between the morning and evening time points (p>0.05, paired t-test) however exhibit greater sleep lost in the evening (p<0.05, paired t-test). Data are means +/- SEM.
-
Figure 4—figure supplement 1—source data 1
Standardized time points show similar effects to points with matched baseline sleep.
- https://cdn.elifesciences.org/articles/74327/elife-74327-fig4-figsupp1-data1-v2.xlsx
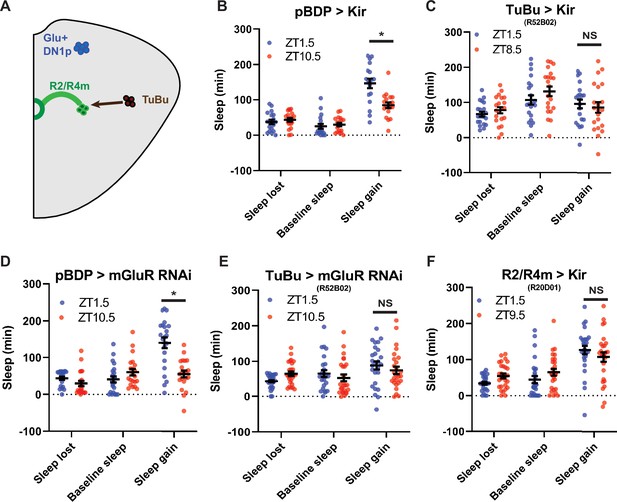
TuBu intermediates convey enhanced morning glutamatergic signal to R2/R4m ellipsoid body neurons (A) Cartoon illustrating proposed link between Glu+ DN1ps and R2/R4m with Tubu intermediates.
(B–F) Comparison of sleep lost, baseline sleep, and sleep gain following deprivation at morning and evening timepoints while modulating neurons linking DN1ps to the EB. Morning times are matched with evening time points with similar baselines. (B) Enhancerless-Gal4 control flies (pBDP >Kir) (N=21) exhibit greater rebound in the morning compared to a matched evening time point (p<0.01, paired t-test). (C) Flies with TuBu neurons silenced (R52B02>Kir) (N=21) do not exhibit a difference in rebound between matched morning/evening time points (p>0.38, paired t-test). (D) Enhancerless-Gal4 driver paired with UAS-GluR-RNAi (pBDP >GluR RNAi) control (N=32) exhibit greater rebound in the morning compared to matched evening time point (p<0.00001, paired t-test). (E) Flies with KD of GluR in TuBu neurons (R52B02>GluR RNAi) do not exhibit a significant difference between matched morning/evening time points (p>0.28, paired t-test). (F) Flies with R2/R4m neurons silenced (R20D01>Kir) (N=32) do not exhibit a significant difference in rebound between matched morning/evening time points (p>0.26, paired t-test). Data are means +/- SEM.
-
Figure 5—source data 1
TuBu intermediates convey enhanced morning glutamatergic signal to R2/R4m ellipsoid body neurons.
- https://cdn.elifesciences.org/articles/74327/elife-74327-fig5-data1-v2.xlsx
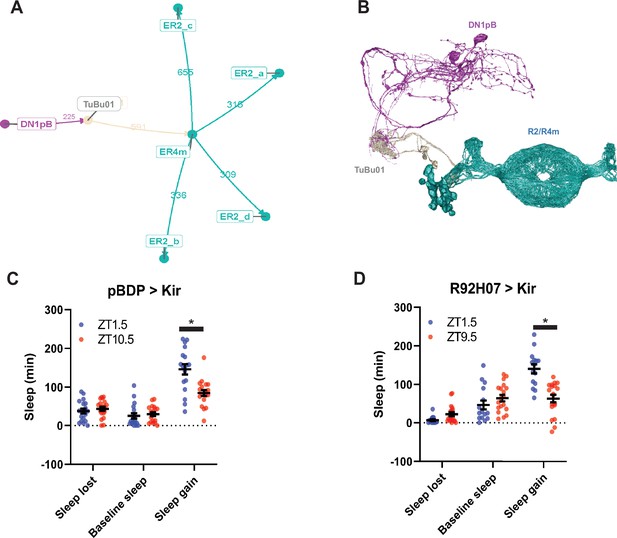
Connectome analysis demonstrates link between anterior projecting DN1ps and R2/R4m ellipsoid body ring neurons.
(A) Node network diagram of pathway from anterior projecting DN1ps to R2/R4m via Tubu intermediates. Arrows indicate directionality of projections and numbers represent average synaptic connections between groups of neurons. (B) Dorsal view of neuronal morphology of pathway from anterior projecting DN1ps to R2/R4m via Tubu intermediates according to Neuprint EM reconstruction. Each cell subtype is color coded to match the model in A. (C–D) Comparison of sleep lost, baseline sleep, and sleep gain following deprivation at morning and evening timepoints while modulating TuBu neurons. Morning times are matched with evening time points with similar baselines. (C) Enhancerless-Gal4 control flies (pBDP >Kir) (N=21) exhibit greater rebound in the morning compared to a matched evening time point (p<0.01, paired t-test). (D) Flies with TuBu neurons silenced (R92H07>Kir) (N=21) exhibit greater rebound in the morning compared to a matched evening time point (p<0.0001, paired t-test).
-
Figure 5—figure supplement 1—source data 1
Connectome analysis demonstrates link between anterior projecting DN1ps and R2/R4m ellipsoid body ring neurons.
- https://cdn.elifesciences.org/articles/74327/elife-74327-fig5-figsupp1-data1-v2.xlsx
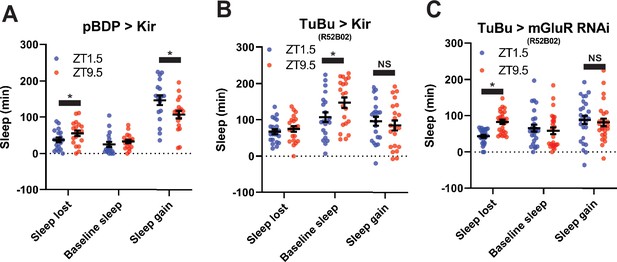
Standardized time points show similar effects to points with matched baseline sleep (A–C) Comparison of sleep lost, baseline sleep, and sleep gain following deprivation at morning and evening timepoints in clock neuron-ablated flies.
Morning times are matched with evening time points with similar baselines. (A) Enhancerless-Gal4 control flies (pBDP >Kir) (N=21) exhibit greater sleep lost for rebound occurring at ZT9.5 (p<0.01, paired t-test) however retain significantly more rebound at ZT 1.5 compared to ZT 9.5 (p<0.05, paired t-test).(B) Flies with TuBu neurons silenced (R52B02>Kir) (N=21) exhibit significantly greater baseline sleep during the rebound period at ZT9.5 (p<0.0001), however do not exhibit a difference in sleep gain between ZT 1.5 and ZT9.5 (p>0.32, paired t-test).(C) Flies with KD of GluR in TuBu neurons (R52B02>GluR RNAi) significantly greater sleep lost at ZT 9.5 (p<0.00001, paired t-test), and exhibit no significant difference in sleep gain between ZT 1.5 and ZT9.5 (p>0.66, paired t-test). Data are means +/- SEM.
-
Figure 5—figure supplement 2—source data 1
Standardized time points show similar effects to points with matched baseline sleep.
- https://cdn.elifesciences.org/articles/74327/elife-74327-fig5-figsupp2-data1-v2.xlsx
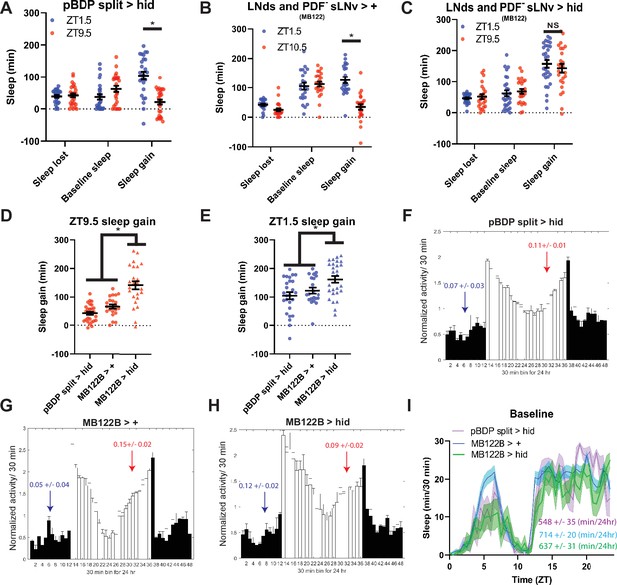
LNds and the PDF- sLNv suppress evening rebound (A–C) Comparison of sleep lost, baseline sleep, and sleep gain following deprivation at morning and evening timepoints in clock neuron-ablated flies.
Morning times are matched with evening time points with similar baselines. (A) hid control flies with no ablated neurons (pBDP split >hid) (N=26) exhibit greater rebound in the morning compared to matched evening time point (p<0.0001, paired t-test). (B) Gal4 control flies with no ablated neurons (MB122B> +) (N=29) exhibit greater rebound in the morning compared to matched evening time point (p<0.01, paired t-test) (C) Flies with 2–3 LNds and the PDF- sLNv ablated (MB122B>hid) (N=30) do not exhibit a significant difference in sleep gain between matched morning/evening time points (p>0.50, paired t-test). (D–F) Averaged activity eductions for female flies during the first 2 days of 12:12 LD. Light phase is indicated by white bars while the dark phase is indicated by black bars. Morning and evening anticipation indices are represented in blue and red respectively. (G) Average sleep during the baseline day for LNds and the PDF- sLNv ablated (MB122B>hid) (N=30) (green), Gal4 control (MB122B > +) (N=29) (blue), and hid control (pBDP split >hid) (N=26) (purple). (H, I) Comparison of sleep gain at ZT1.5 (H) and ZT9.5 (I) between flies with 2–3 LNds and the PDF- sLNv ablated (MB122B>hid) (N=29) and their controls (pBDP split >hid) (N=26)(MB122B> +) (N=29). MB122B>hid flies exhibit greater rebound at morning (H) (p<0.05, ANOVA) and evening (I) (p<0.001, ANOVA). Data are means +/- SEM.
-
Figure 6—source data 1
LNds and the PDF- sLNv suppress evening rebound.
- https://cdn.elifesciences.org/articles/74327/elife-74327-fig6-data1-v2.xlsx
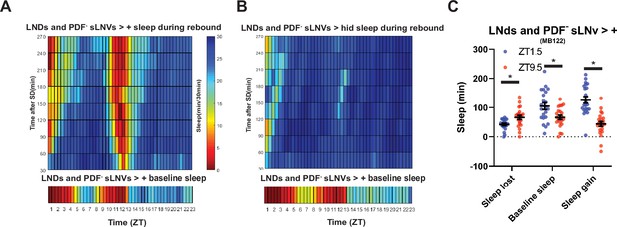
Full SSD demonstrates loss of evening suppression of rebound in LNd ablated flies (A–B) Rebound sleep heatmaps (above) illustrate average sleep as a function of time of day when rebound occurred (ZT) and minutes after SSD episode.
Missing time points are filled using matlab linear interpolation function. Baseline sleep heatmaps (below) illustrate average sleep during 30 min bins. (A) Gal4 controls with no ablated neurons (MB122B> +) (N=29) display low baseline sleep following lights on and preceding lights off. Immediately following SD flies show high sleep except in the hours preceding lights off. Flies tend to sleep less as rebound time proceeds. (B) Flies with 2–3 LNds and the PDF- sLNv ablated (MB122B>hid) (N=30) display low baseline sleep following lights on and preceding lights off. Following SD these flies show high sleep across time points except following lights on. Flies tend to sleep less as rebound time proceeds. (C) Comparison of sleep lost, baseline sleep, and sleep gain following deprivation at morning and evening timepoints in clock neuron-ablated flies. Morning times are matched with evening time points with similar baselines. Gal4 control flies with no ablated neurons (MB122B> +) (N=29) exhibit greater rebound in the morning compared to the evening time point (p<0.01, paired t-test), however, exhibit greater sleep lost in the evening (p<0.01) and greater baseline sleep during the rebound period (p<0.05). Data are means +/- SEM.
-
Figure 6—figure supplement 1—source data 1
Full SSD demonstrates loss of evening suppression of rebound in LNd ablated flies.
- https://cdn.elifesciences.org/articles/74327/elife-74327-fig6-figsupp1-data1-v2.xlsx
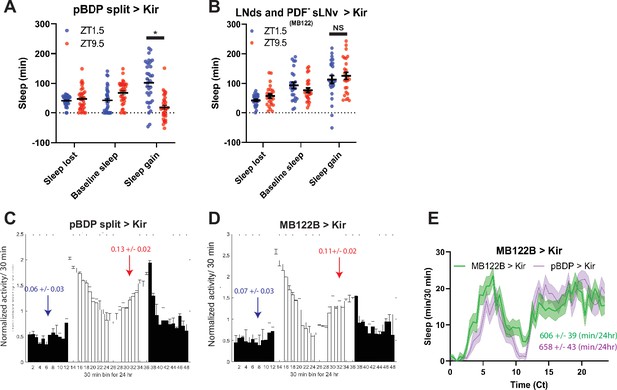
Silencing LNds and the PDF- sLNv suppresses evening rebound (A–B) Comparison of sleep lost, baseline sleep, and sleep gain following deprivation at morning and evening timepoints in clock neuron-ablated flies.
Morning times are matched with evening time points with similar baselines. (A) Control flies with no silenced neurons (pBDP split >Kir) (N=34) exhibit greater rebound in the morning compared to matched evening time point (p<0.0001, paired t-test). (B) Flies with 2–3 LNds and the PDF- sLNv silenced (MB122B>Kir) (N=31) do not exhibit a significant difference in sleep gain between matched morning/evening time points (p>0.45, paired t-test). (C,D) Averaged activity eductions for female flies during the first 2 days of 12:12 LD. Light phase is indicated by white bars while the dark phase is indicated by black bars. Morning and evening anticipation indices are represented in blue and red respectively. (E) Average sleep during the baseline day.(H) 2–3 LNds and the PDF- sLNv silenced (MB122B>Kir) (N=31) (green) and control (pBDP split >Kir) (N=34) (purple). Sleep per 24 hr is indicated in the bottom right. Data are means +/- SEM.
-
Figure 6—figure supplement 2—source data 1
Silencing LNds and the PDF- sLNv suppresses evening rebound.
- https://cdn.elifesciences.org/articles/74327/elife-74327-fig6-figsupp2-data1-v2.xlsx
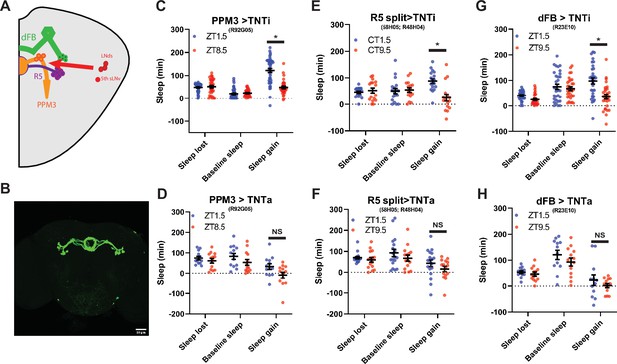
PPM3 convey enhancing homeostatic signal to R5 ellipsoid body neurons (A) Cartoon illustrating link between LNds and 5th sLNv and dFB via with PPM3 and R5 intermediates.
(B) GFP Expression pattern of split Gal4 line that labels Glu+ DN1ps (R58H05 AD; R48H04 DBD >GFP) at 20 x. (C–H) Comparison of sleep lost, baseline sleep, and sleep gain following deprivation at morning and evening timepoints modulating neurons linking LNd activity to the EB. Morning times are matched with evening time points with similar baselines. (C) Flies expressing an inactive form of tetanus toxin in PPM3 neurons (R92G05>TNTi)(N=45) exhibit greater rebound in the morning than at a matched evening time point (p<0.0001, paired t-test). (D) Silencing PPM3 neurons with an active form of tetanus toxin (R92G05>TNTa)(N=27) resulted in no significant difference between matched morning/evening time points (p>0.10, paired t-test). (E) Flies expressing an inactive form of tetanus toxin in R5 neurons (R58H05 AD; R48H04 DBD >TNTi) (N=21) exhibit greater rebound in the morning than at a matched evening time point (p<0.01, paired t-test). (F) Silencing R5 neurons with tetanus toxin (R58H05 AD; R48H04 DBD >TNTa) (N=16) resulted in no significant difference in sleep gain for matched morning and evening time points (p>0.70, paired t-test). (G) Flies expressing an inactive form of tetanus toxin in the dFB (R23E10>TNTi) (N=30) exhibit greater rebound in the morning than at a matched evening time point (p<0.0001, paired t-test). (H) Silencing dFB neurons with tetanus toxin (R23E10>TNTa) (N=12) resulted in no significant difference between morning and evening time points (p>0.45, paired t-test).
-
Figure 7—source data 1
PPM3 convey enhancing homeostatic signal to R5 ellipsoid body neurons.
- https://cdn.elifesciences.org/articles/74327/elife-74327-fig7-data1-v2.xlsx
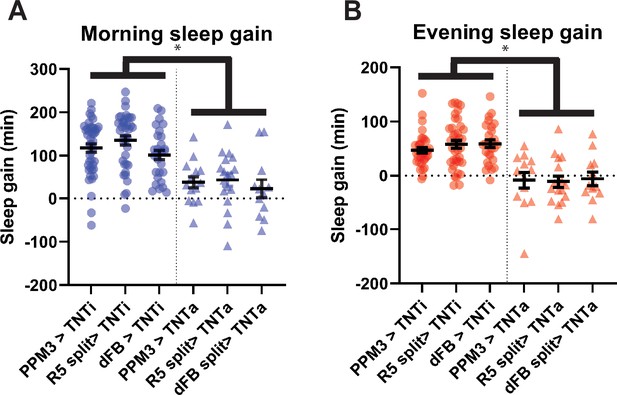
Silencing of PPM3, dFB and R5 reduces rebound in the morning and evening (A, B) Comparison of sleep gain at morning (A) and evening (B) timepoints between flies with silenced neurons (TNTa) and their controls (TNTi).
Flies expressing an inactive form of tetanus toxin in PPM3 neurons (R92G05>TNTi)(N=45) exhibit greater rebound than flies with silenced PPM3 neurons (R92G05>TNTa)(N=27) in the morning (A) (p<0.0001, t-test) and evening (B) (p<0.0001, t-test). Flies expressing an inactive form of tetanus toxin in R5 neurons (R58H05 AD; R48H04 DBD >TNTi) (N=21) exhibit greater rebound than flies with R5 neurons silenced (R58H05 AD; R48H04 DBD >TNTa) (N=16) in both the morning (A) (p<0.0001, t-test) and evening (B) (p<0.0001, t-test). Flies expressing an inactive form of tetanus toxin in the dFB (R23E10>TNTi) (N=30) than flies with dFB neurons silenced (R23E10>TNTa)(N=12) in the morning (A) (p<0.0001, t-test) and evening (B) (p<0.0001, t-test). Data are means +/- SEM.
-
Figure 7—figure supplement 1—source data 1
Silencing of PPM3, dFB, and R5 reduces rebound in the morning and evening.
- https://cdn.elifesciences.org/articles/74327/elife-74327-fig7-figsupp1-data1-v2.xlsx
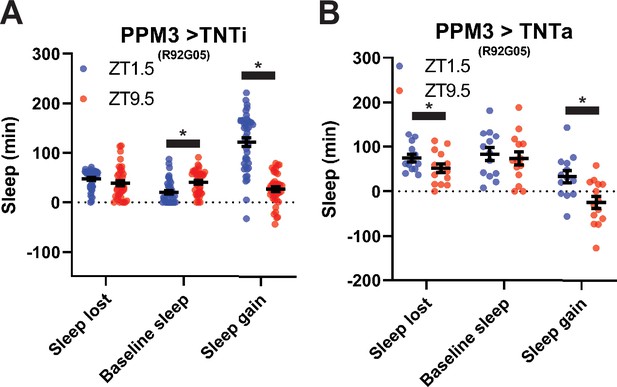
Standardized time points show similar effects to points with matched baseline sleep (A–C) FD sleep during two baseline time periods (sleep lost and baseline sleep) and sleep gain for rebound occurring at ZT1.5 and ZT 9.5.
(A) Flies expressing an inactive form of tetanus toxin in PPM3 neurons (R92G05>TNTi)(N=45) exhibit greater baseline sleep during the rebound period at ZT9.5 (p<0.001 paired t-test) and exhibit significantly greater rebound at ZT 1.5 compared to ZT9.5 (p<0.0001, paired t-test).(B) Flies with PPM3 neurons silenced with the active form of tetanus toxin (R92G05>TNTa)(N=27) exhibit significantly less sleep lost (p<0.05, paired t-test) and sleep gain (p<0.0001, paired t-test) at ZT 9.5 compared to ZT1.5. Data are means +/- SEM.
-
Figure 7—figure supplement 2—source data 1
Standardized time points show similar effects to points with matched baseline sleep.
- https://cdn.elifesciences.org/articles/74327/elife-74327-fig7-figsupp2-data1-v2.xlsx
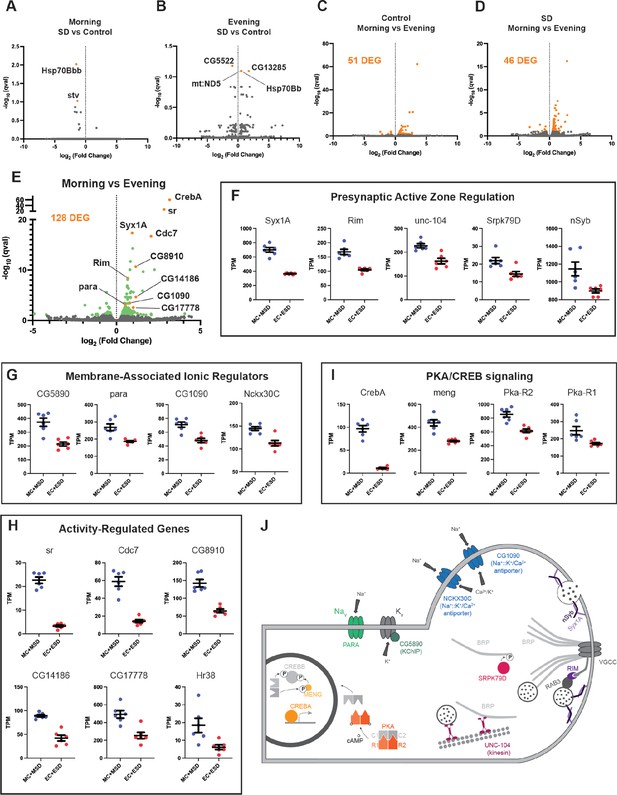
RNA sequencing of FAC-sorted R5 neurons suggests elevated activity in the morning (A) Volcano plot (fold change versus qval) of Morning SD (MSD) vs Morning Control (MC) gene expression.
Significantly differentially expressed genes shown in orange. (B) Volcano plot of Evening SD (ESD) vs Evening Control (MC) gene expression. Significantly differentially expressed genes shown in orange. (C) Volcano plot of MC vs EC gene expression. 51 significantly differentially expressed genes (DEG) were identified and are shown in orange. (D) Volcano plot of MSD vs ESD gene expression. 46 significantly differentially expressed genes (DEG) were identified and are shown in orange. (E) Volcano plot of MC +MSD vs EC +ESD gene expression. Differentially expressed genes are shown in green with a few genes highlighted in orange and labeled. (F–I) Scatter plots for several differentially expressed genes. Transcripts Per Kilobase Million (TPM) is shown for each sample. All morning samples are grouped, and all evening samples are grouped. Graphs are grouped by similar functions: (F) active zone components/regulators, (G) membrane-associated ionic regulators, (H) activity-regulated genes, (I) PKA/CREB signaling. (J) Schematic of select morning upregulated genes. Upregulated genes are shown in color while other interacting components are depicted in gray. PARA and CG5890 are both involved in the generation and propagation of action potentials. Multiple active zone components/regulators (NSYB, SYX1A, RIM, SRPK79D, UNC-104) interact with BRP and voltage-gated calcium channels (VGCCs) to support neuronal output and intracellular calcium influx. Elevated levels of intracellular calcium are regulated by the antiporters NCKX30C and CG1090. Second messenger cAMP interacts with regulatory subunits of PKA (R1/R2) and releases the catalytic subunits (C1/C2) to phosphorylate CREBB and MENG, stabilizing CREBB. CREBA acts as a transcriptional activator independent of PKA activity.
-
Figure 8—source data 1
RNA sequencing of FAC-sorted R5 neurons suggests elevated activity in the morning.
- https://cdn.elifesciences.org/articles/74327/elife-74327-fig8-data1-v2.xlsx
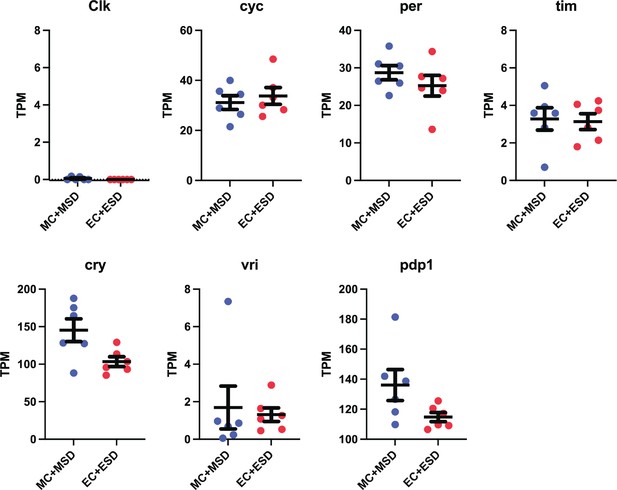
Clock genes are not different between morning and evening in R5 neurons Scatter plots for core clock genes.
Transcripts Per Kilobase Million (TPM) is shown for each sample. All morning samples are grouped, and all evening samples are grouped.
-
Figure 8—figure supplement 1—source data 1
Clock genes are not different between morning and evening in R5 neurons.
- https://cdn.elifesciences.org/articles/74327/elife-74327-fig8-figsupp1-data1-v2.pdf
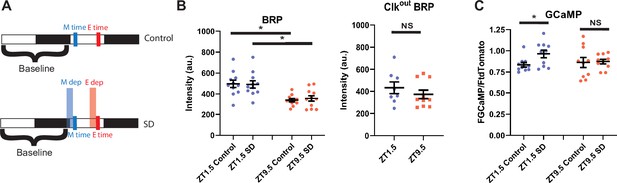
R5 neurons exhibit time dependent changes in BRP and calcium response to SD (A) Schematic illustrating deprivation and dissection timing for morning (M) and evening (E) with (lower) and without (upper) SD.
(B) Fluorescence of BRP-STaR in R5 projections as a function of time of day and SD in WT (left)and Clkout mutant (right) backgrounds. Intensity of BRP staining is decreased at ZT9.5 compared to ZT1.5 in both control (N=11, 11)(p<0.001, independent t-test) and SD (N=11, 11) (p<0.01, independent t-test) groups. Intensity of BRP staining is not affected by SD in the morning (N=11,11) (p>0.90, independent t-test) or evening (N=11,11) (p>0.58, independent t-test). Intensity of BRP staining in Clkout mutants is not significantly different at ZT1.5 (N=8) compared to ZT9.5 (N=10) (p>0.36, independent t-test).(D) GCaMP expression in R5 projections (R69F08>GCamP6 s) at ZT1.5 and ZT9.5 with and without SD. GCaMP fluorescence was normalized to the tdTomato fluorescence signal intensity. There is no difference in normalized GCaMP6s signaling between baseline morning (N=10) and evening (N=10) time points. SD in the morning (N=10) increases GCaMP6s intensity (p<0.05, independent t-test) but not in the evening (N=10) (p>0.87 independent t-test), independent t-test. Data are means +/- SEM.
-
Figure 9—source data 1
R5 neurons exhibit time dependent changes in BRP and calcium response to SD.
- https://cdn.elifesciences.org/articles/74327/elife-74327-fig9-data1-v2.xlsx
Videos
Flies exhibit sleep following 2.5 hr SD terminating at ZT1.5 Sped up video recording of 4.5 hr of rebound of 36 WT flies following SD from ZT23-ZT1.5.
Hours post SD are indicated in red in the bottom right corner. Flies exhibit little movement throughout the 4.5 hr following SD indicating sleep.
Flies are active following 2.5 hr SD terminating at ZT9.5 Sped up video recording of 4.5 hr of rebound of 36 WT flies following SD from ZT7-ZT9.5.
Hours post SD are indicated in red in the bottom right corner. After a brief period of immobility flies exhibit high activity (low sleep) preceding lights on.
Tables
Summary of male morning and evening anticipation.
Genotype | Region/Cells targeted | LD morning anticipation | LD evening anticipation | N |
---|---|---|---|---|
+>hid | Clock Gal4 control | 0.14+/-0.04 | 0.37+/-0.03 | 17 |
pBDP split >hid | Split control (HID) | 0.13+/-0.02 | 0.24+/-0.03 | 26 |
pBDP split >kir | Split control (Kir) | 0.10+/-0.02 | 0.33+/-0.04 | 12 |
cry39>hid | broad clock | 0.05+/-0.02 ** | 0.12+/-0.04 *** | 30 |
pdf >hid | –0.07+/-0.02 *** | 0.24+/-0.03* | 38 | |
cry39; pdf-gal80 >hid | LNd and Dn1 | 0.05+/-0.01 * | 0.06+/-0.04 *** | 14 |
R51H05 AD; R18H11 DBD >hid | Glu +DN1 p | 0.06+/-0.02 * | 0.25+/-0.02 | 22 |
MB122 >hid | 3–4 LNds PDF-sLNv | 0.12+/-0.02 | 0.25+/-0.02 | 35 |
MB122 >kir | 3–4 LNds PDF-sLNv | 0.07+/-0.02 | 0.22+/-0.02 | 26 |
-
Data are means +/- SEM (*p<0.05, **p<0.01, ***p<0.001).