An Archaea-specific c-type cytochrome maturation machinery is crucial for methanogenesis in Methanosarcina acetivorans
Figures
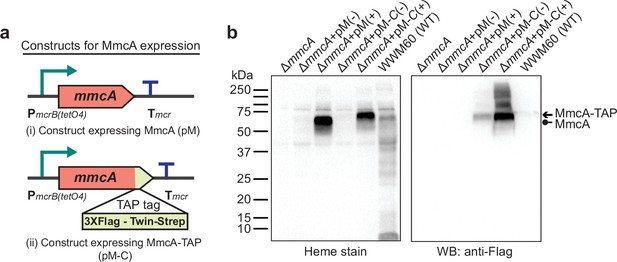
Experimental assays to measure the production and maturation of the diagnostic c-type Cytochrome, MmcA.
(a) The design of diagnostic constructs to use the native heptaheme c-type cytochrome, MmcA, to map the cytochrome c biogenesis pathway in Methanosarcina acetivorans. (i) The control construct (pM) contains an mmcA coding sequence whereas (ii) the test construct (pM-C) contains an mmcA coding sequence with a C-terminal translational fusion of a tandem affinity purification (TAP) tag comprised of a 3× FLAG sequence and a Twin-Strep sequence. In each construct, gene expression is driven by a tetracycline-inducible medium-strength promoter, PmcrB(tetO4), and a transcriptional terminator of the mcr operon from M. acetivorans is provided after the last coding sequence. (b) Assays to measure holo-MmcA formation by covalent heme attachment and detect the tagged MmcA protein in whole cell lysates of M. acetivorans mutants. (Left) A heme peroxidase-based assay is used to detect the presence of proteins with covalently bound heme in whole cells lysates. Using this assay, both untagged (lane 3) and tagged (lane 5) heme-bound holo-MmcA can be detected upon induction of the genes from each plasmid construct described above. The tagged MmcA protein runs at a higher molecular weight due to the presence of a ca. 7.3 kDa TAP tag at the C-terminus compared to the native MmcA protein. (Right) Immunoblotting with commercial anti-FLAG antibody can be used detect MmcA production in the test construct containing a C-terminal TAP tag fused to the mmcA gene (lanes 4 and 5). (-) indicates that no tetracycline was added to the growth medium and (+) indicates that 100 µg/mL tetracycline was added to the growth medium. An equal amount of whole cell lysate protein (80 µg) was loaded in each lane.
-
Figure 1—source data 1
Raw gel and blot images.
- https://cdn.elifesciences.org/articles/76970/elife-76970-fig1-data1-v2.pdf
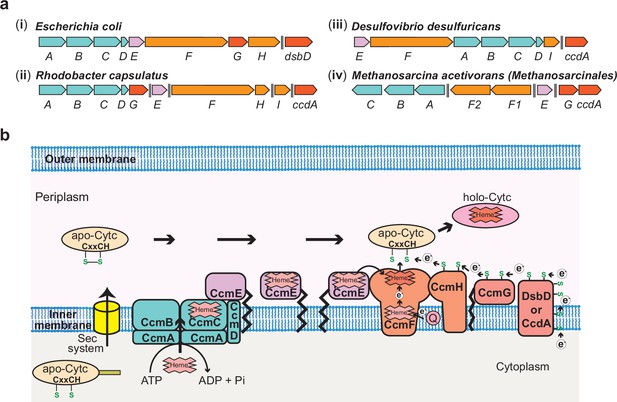
The Ccm Machinery in Bacteria and Archaea.
(a) Chromosomal organization of the System I c-type cytochrome (cyt c) maturation (Ccm) machinery genes in a few representative bacteria and the archaeon, Methanosarcina acetivorans. In bacteria like (i) Escherichia coli, (ii) Rhodobacter capsulatus, and (iii) Desulfovibrio desulfuricans, 9–10 genes (ccmABCDEFGHI and dsbD/ccdA) are involved in the maturation of cyt(s) c, and most of the ccm genes are found in an operon. (iv) The ccm genes in the archaeon M. acetivorans and other methane-metabolizing archaea belonging to the order Methanosarcinales are not found in a single operon and, notably, homologs of three essential genes, ccmD, ccmH, and ccmI, are absent. Double dashed lines indicate a distance >110 kbp on the chromosome. (b) An overview of the System I Ccm machinery from E. coli. The apo-cyt-c is transported across the inner cytoplasmic membrane into the periplasmic space using the Sec system. The CcmABCD membrane-bound complex transports heme b to form heme-bound holo-CcmE in an ATP-dependent manner. Holo-CcmE delivers heme b to the membrane-bound cyt c synthetase complex, CcmFH, that catalyzes the formation of holo-cyt c. CcmG and DsbD/CcdA facilitate the transfer of electrons to reduce the disulfide bond between the cysteine residues in the CXXCH motif of the apo-cyt c prior to the formation of holo-cyt c. Figure adapted from Kranz et al., 2009.
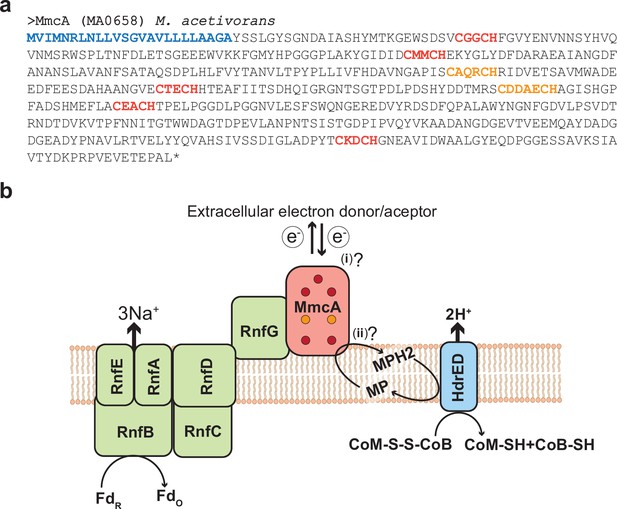
MmcA is a heptaheme c-type cytochrome associated with the Rnf complex in Methanosarcina acetivorans.
(a) Amino acid sequence of the c-type cytochrome (cyt c) MmcA from Methanosarcina acetivorans. The predicted signal peptide is shown in blue, five canonical heme-binding motifs (CXXCH) are shown in red and two non-canonical heme-binding motifs (CXXXCH and CXXXXCH) are shown in orange. Based on these observations, MmcA is a heptaheme cyt c that is present in the pseudo-periplasm of M. acetivorans. (b) MmcA is associated with the Rhodobacter nitrogen fixation (Rnf) bioenergetic complex in methanogens and other alkane-metabolizing archaea. The Rnf complex catalyzes the electron transfer reaction between cytosolic Ferredoxin (Fd) and the membrane-associated electron carrier methanophenazine (MP) coupled to the translocation of Na+ ions across the cytoplasmic membrane. MmcA has been hypothesized to play two roles in the context of the Rnf bioenergetic complex: (i) it is involved in the extracellular transfer of electrons from/to Fd or (ii) it directly interacts with MP to mediate the final step in the intracellular electron transport reactions from Fd to MP. The reduced MPH2 generated by the Rnf complex is regenerated by the membrane-bound heterodisulfide reductase (Hdr) that couples the translocation of protons (H+) to the transfer of electrons from MP to the CoM-S-S-CoB disulfide that acts as the terminal electron acceptor in cytochrome-containing methanogens like M. acetivorans.
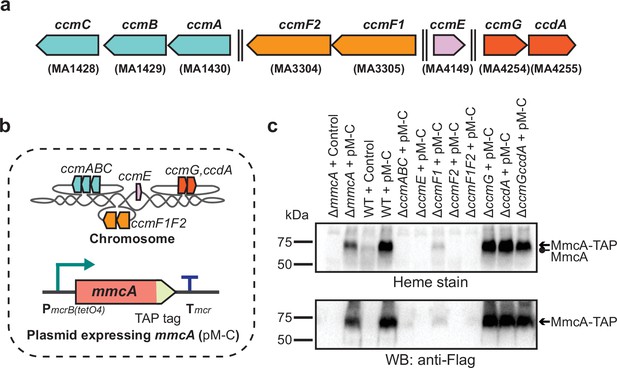
Identifying the role of Ccm genes in c-type cytochrome maturation in Methanosarcina acetivorans.
(a) Chromosomal organization of the System I cytochrome c maturation machinery (Ccm) genes in Methanosarcina acetivorans. Double dashed lines indicate that the genes are located more than 110 kbp away on the chromosome. Genes located at the same chromosomal locus are shown in the same color. (b) A schematic showing the genotype of strains used to assay the role of individual ccm genes in the maturation of c-type cytochromes. In addition to the corresponding ccm-deletion on the chromosome, these strains also contain a self-replicating plasmid (pM-C) where the mmcA coding sequence with a C-terminal tandem affinity purification (TAP) tag comprised of a 3× FLAG sequence and a Twin-Strep sequence is placed under the control of a tetracycline-inducible medium-strength promoter, PmcrB(tetO4). In these strains, the expression of the C-terminal TAP-tagged MmcA can be induced by the addition of tetracycline to the growth medium. (c) A heme peroxidase-based assay to measure the formation of heme-bound holo-MmcA (top) and Western blots (WB) with anti-FLAG antibody to detect the production of C-terminal TAP-tagged MmcA (bottom). Neither holo-MmcA nor tagged protein could be detected in the ∆ccmCBA, ∆ccmE, ∆ccmF2 single mutants, and the ∆ccmF1∆ccmF2 double mutant suggesting that the apo-MmcA undergoes rapid proteolysis in the absence of a functional Ccm machinery. All assays were conducted with whole cell lysates of cultures grown in medium containing 100 µg/mL tetracycline to induce the expression of C-terminal TAP-tagged MmcA. An equal amount of whole cell lysate protein (60 µg) was loaded in each lane. The vector control (i.e. empty vector) used for this experiment is pJK029A as described previously in Guss et al., 2008.
-
Figure 2—source data 1
Raw gel and blot images.
- https://cdn.elifesciences.org/articles/76970/elife-76970-fig2-data1-v2.pdf
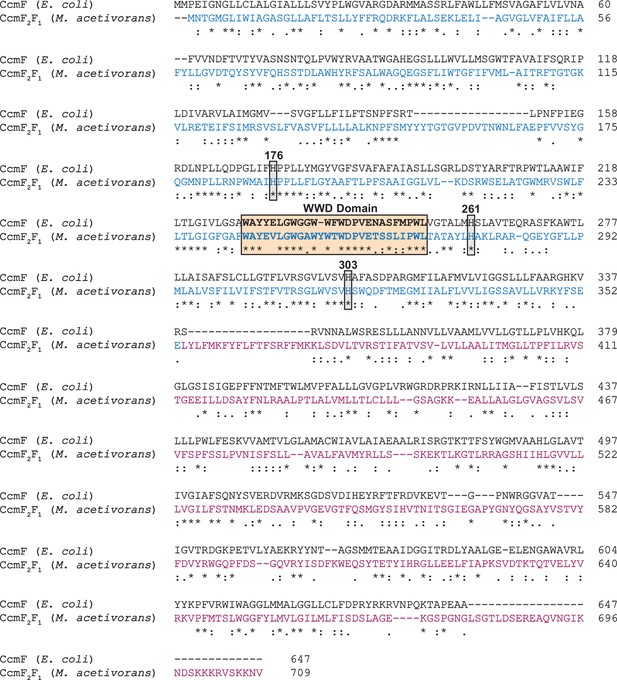
Alignment of the CcmF amino acid sequence from Escherichia coli with the concatenated CcmF2-CcmF1 amino acid sequences from Methanosarcina acetivorans.
Based on this alignment, the CcmF2 sequence (in blue) from M. acetivorans is homologous to N-terminus of CcmF from bacteria and the CcmF1 (in purple) sequence of M. acetivorans is homologous to the C-terminus of CcmF from bacteria. The highly conserved WWD domain, and three conserved histidine residues that serve as axial ligands for heme b in the membrane (His261 in E. coli) and the periplasm (His176 and His303 in E. coli) are all conserved in the CcmF2 sequence of M. acetivorans.
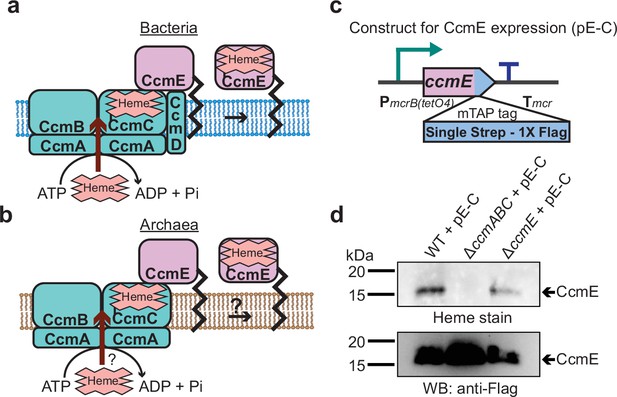
CcmABC is required for the heme transport to form holo-CcmE in Methanosarcina acetivorans.
(a) The formation of heme-bound holo-CcmE in bacteria containing the System I Ccm machinery is facilitated by the CcmABCD complex. The ATP-dependent CcmABC complex translocates heme b from the cytosol to CcmE and CcmD facilitates the dissociation of holo-CcmE from the CcmABCDE adduct. (b) Since archaea lack the ccmD gene, the formation of holo-CcmE is unclear. One hypothesis, shown here, is that the CcmABC complex in archaea mediates the dissociation of holo-ccmE independent of CcmD. (c) The design of a test construct (pE-C) containing the ccmE coding sequence with C-terminal translational fusion of a modified tandem affinity purification (mTAP) tag comprising of a Single-Strep sequence and a 1× FLAG sequence. In this construct, the coding sequence is placed in-between a tetracycline-inducible medium-strength promoter, PmcrB(tetO4) (Guss et al., 2008), and the transcriptional terminator of the mcr operon from Methanosarcina acetivorans. Here, the expression of the C-terminal mTAP-tagged CcmE can be induced by the addition of tetracycline to the growth medium. (d) A heme peroxidase-based assay to measure the formation of holo-CcmE (top) and a Western blot (WB) with anti-FLAG antibody to detect the production of C-terminal mTAP-tagged CcmE (bottom) was conducted. Even though CcmE is produced in the ∆ccmABC mutant, holo-CcmE cannot be formed, consistent with the model shown in (b). All assays were performed with an enriched membrane fraction (i.e. protein eluted after passing the membrane fraction through a Strep-affinity column) of cultures grown in medium containing 100 µg/mL tetracycline to induce the expression of C-terminal mTAP-tagged CcmE. An equal amount of protein (1 µg) was loaded in each lane.
-
Figure 3—source data 1
Raw gel and blot images.
- https://cdn.elifesciences.org/articles/76970/elife-76970-fig3-data1-v2.pdf
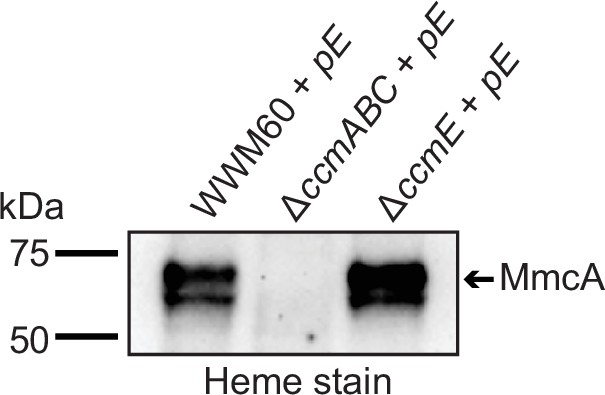
Expression of CcmE with a C-terminal 1× FLAG-1× Strep tag from a plasmid (pE) in the parent strain of Methanosarcina acetivorans (WWM60) and the ∆ccmE background leads to the production of heme-bound holo-MmcA as detected by a heme peroxidase assay of whole cell lysates (lanes 1, 3 of heme-stained gel).
Thus, the C-tagged CcmE is functional and facilitates the maturation of native c-type cytochromes (cyt c) like MmcA. In contrast, expression of C-tagged CcmE in the ∆ccmABC background does not produce any holo-MmcA (lane 2 of heme-stained gel). In the absence of CcmABC, holo-CcmE does not form, which, in turn, prevents the maturation of native cyt c like MmcA. All cultures were grown in medium containing 100 µg/mL tetracycline to induce the expression of C-tagged CcmE from the plasmid pE. An equal amount of protein (~20 µg) was loaded in each lane.
-
Figure 3—figure supplement 1—source data 1
Raw gel and blot images.
- https://cdn.elifesciences.org/articles/76970/elife-76970-fig3-figsupp1-data1-v2.pdf
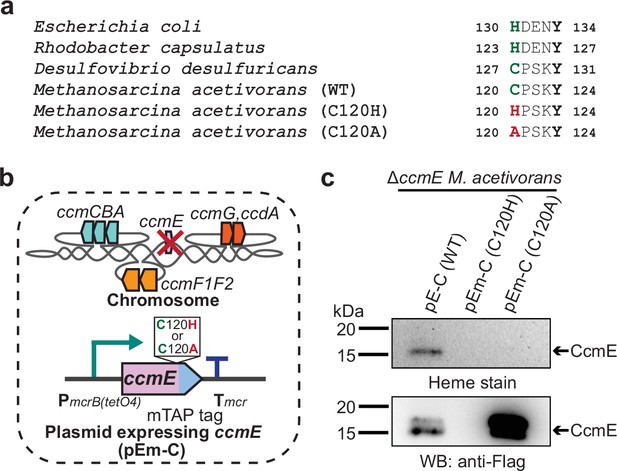
Identifying the heme-binding residue in CcmE from Methanosarcina acetivorans.
(a) Alignment of the heme-binding domain in CcmE sequences derived from representative bacteria and archaea. The heme-binding residue in bacteria can vary: some species like Escherichia coli covalently bind heme using a histidine residue whereas others like Desulfovibrio desulfuricans covalently bind heme using a cysteine residue. Almost all archaeal CcmE sequences, including the sequence derived from Methanosarcina acetivorans, contain a conserved cysteine residue that is likely involved in heme binding. (b) To test the role of the cysteine residue in the CcmE sequence derived from M. acetivorans, we expressed the C120A and C120H point mutants of ccmE with a modified tandem affinity purification (mTAP) tag comprising of a Single-Strep sequence and a 1× FLAG sequence at the C-terminus as described in Figure 3c. (c) A heme peroxidase-based assay was used to measure the formation of holo-CcmE (top) and Western blot (WB) with anti-FLAG antibody was used to detect the production of C-terminal mTAP-tagged CcmE (bottom). Neither the C120A or the C120H mutants of CcmE contain covalently bound heme (top); furthermore, no mTAP-tagged CcmE was detected by WB for the C120H mutant (bottom), possibly indicating that this point mutation destabilizes the protein substantially. All assays were performed with an enriched membrane fraction (i.e. protein eluted after passing the membrane fraction through a Strep-affinity column) of cultures grown in medium containing 100 µg/mL tetracycline to induce the expression of C-terminal mTAP-tagged CcmE. An equal amount of protein (1 µg) was loaded in each lane.
-
Figure 4—source data 1
Raw gel and blot images.
- https://cdn.elifesciences.org/articles/76970/elife-76970-fig4-data1-v2.pdf
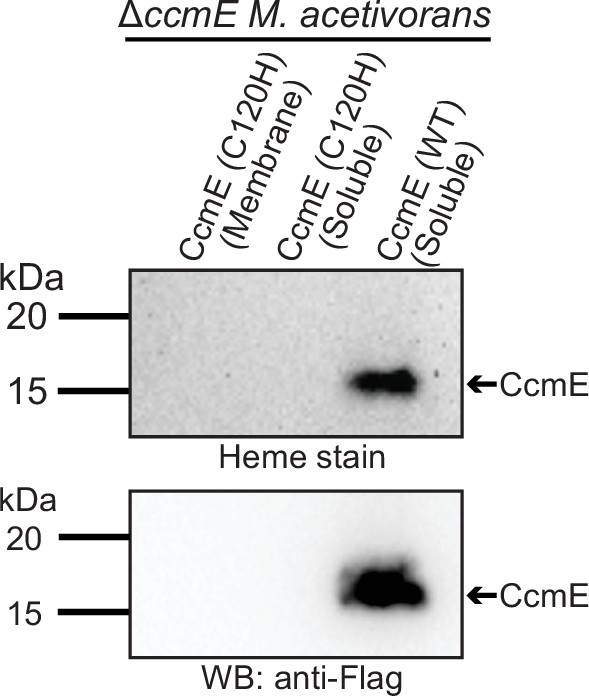
Expression of the C120H mutant of CcmE from Methanosarcina acetivorans with a C-terminal 1× FLAG-1× Strep tag in the ∆ccmE background does not lead to the production of any CcmE protein that can be detected by heme peroxidase assays (top gel) or Western blot using an anti-Flag antibody (bottom gel) in the Strep-enriched membrane fraction (lane 1) or the soluble fraction (lane 2).
In contrast, expression of the wild-type (WT) sequence CcmE from M.acetivorans with a C-terminal 1× FLAG-1× Strep tag in the ∆ccmE background leads to the production of protein that can be detected by heme peroxidase assays (top gel) and Western blot using an anti-Flag antibody (bottom gel) in the Strep-enriched soluble fraction (lane 3) and the membrane fraction (see Figure 4). Strep-enriched membrane or soluble fraction represents protein eluted after passing the membrane or the soluble fraction through a Strep-affinity column. The C120H mutant was sequence-verified prior to protein purification. Based on these data, it is highly likely that the C120H substitution destabilizes the CcmE protein. All cultures were grown in medium containing 100 µg/mL tetracycline to induce the expression of the tagged CcmE protein. An equal amount of protein (~1 µg) was loaded in each lane.
-
Figure 4—figure supplement 1—source data 1
Raw gel and blot images.
- https://cdn.elifesciences.org/articles/76970/elife-76970-fig4-figsupp1-data1-v2.pdf
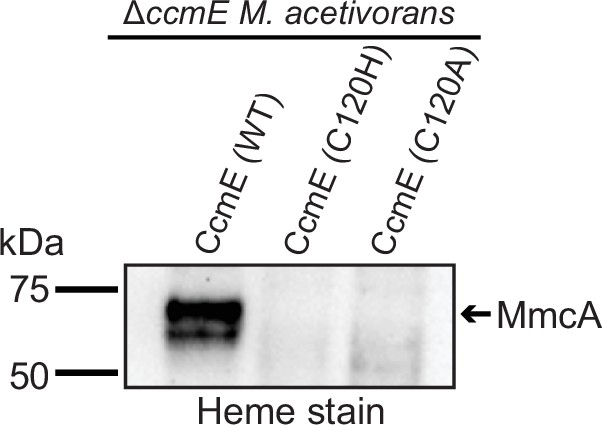
Expression of the wild-type (WT) sequence of CcmE from Methanosarcina acetivorans with a C-terminal 1× FLAG-1× Strep tag (C-tagged CcmE) in the ∆ccmE background leads to the production of heme-bound holo-MmcA as detected by a heme peroxidase assay of whole cell lysates (lane 1 of heme-stained gel).
Thus, the C-tagged WT sequence of CcmE is functional and facilitates the maturation of native c-type cytochromes (cyt c) like MmcA. In contrast, expression of C-tagged C120H or C120A mutants of CcmE in the ∆ccmE background does not produce any holo-MmcA (lanes 2, 3 of heme-stained gel). Thus, the C120H and C120A mutants disrupt the function of CcmE, which, in turn, prevents the maturation of native cyt c like MmcA. All cultures were grown in medium containing 100 µg/mL tetracycline to induce the expression of C-tagged CcmE. An equal amount of protein (~20 µg) was loaded in each lane.
-
Figure 4—figure supplement 2—source data 1
Raw gel and blot images.
- https://cdn.elifesciences.org/articles/76970/elife-76970-fig4-figsupp2-data1-v2.pdf
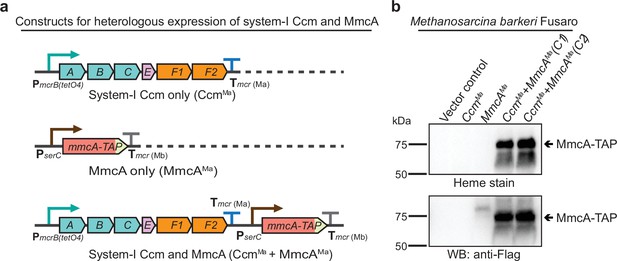
Heterologous expression of the Ccm machinery and the diagnostic c-type cytochrome MmcA in Methanosarcina barkeri Fusaro.
(a) The design of constructs to express a synthetic operon comprised of the ccmABCEF1F2 genes from Methanosarcina acetivorans and/or the mmcA coding sequence from M. acetivorans with a C-terminal tandem affinity purification (TAP) tag comprised of a 3× FLAG sequence and a Twin-Strep sequence. In these constructs, the expression of the ccmABCEF1F2 operon is driven by a tetracycline-inducible medium-strength promoter, PmcrB(tetO4), and a transcriptional terminator of the mcr operon from M. acetivorans Tmcr(Ma) is provided after the last coding sequence. Each gene, apart from ccmA, contains its native Shine-Dalgarno sequence. The C-terminal TAP-tagged MmcA is placed under the control of a constitutive medium-strength promoter (PserC) and the transcriptional terminator of the mcr operon from Methanosarcina barkeri Tmcr(Mb) has been added immediately downstream of the coding sequence. These constructs are integrated at the ØC31 attachment site at a neutral locus on the M. barkeri Fusaro. (b) Heme peroxidase assays to detect the production of heme-bound Holo-MmcA (top) and Western blots (WB) to detect C-terminal TAP-Tagged MmcA protein (bottom) in whole cell lysates of M. barkeri Fusaro cultures grown in medium containing 100 µg/mL tetracycline. A diagnostic c-type cytochrome like MmcA can be produced in a heterologous host lacking a native c-type cytochrome maturation machinery (M. barkeri) by expressing the ccmABCEF1F2 genes from M. acetivorans (lanes 4–5). C1 and C2 refer to two independently colonies of the M. barkeri Fusaro cointegrate expressing the Ccm machinery and MmcA from M. acetivorans. An equal amount of whole cell lysate (60 µg) was loaded in each lane. The vector control (i.e. empty vector) used for this experiment is pJK029A as described elsewhere (Guss et al., 2008).
-
Figure 5—source data 1
Raw gel and blot images.
- https://cdn.elifesciences.org/articles/76970/elife-76970-fig5-data1-v2.pdf
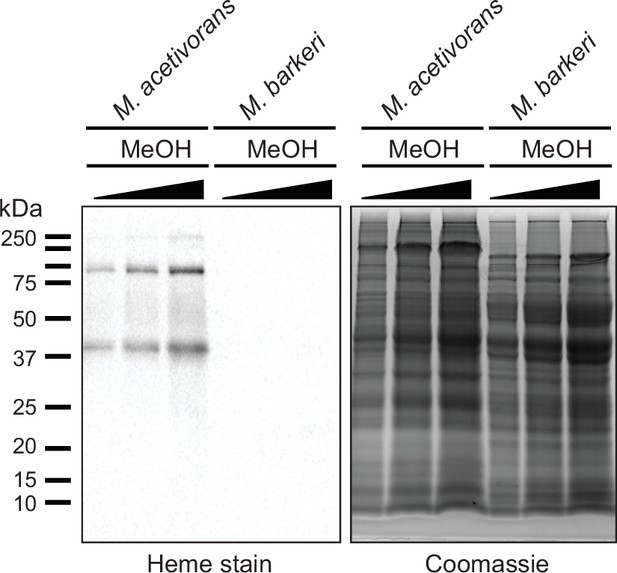
Measuring c-type cytochrome production in cell lysates from Methanosarcina acetivorans and Methanosarcina barkeri.
(Left) Heme peroxidase assays to detect proteins that covalent bind heme (including c-type cytochromes) in whole cell lysates of Methanosarcina acetivorans (lanes 1, 2, 3) and Methanosarcina barkeri (lanes 4, 5, 6) grown in high-salt minimal medium with 125 mM methanol as the sole source of carbon and energy. Three different concentrations (~30, 60, 90 µg) of whole cell lysate were used per strain for these assays as shown in the Coomassie gel control (right).
-
Figure 5—figure supplement 1—source data 1
Raw gel and blot images.
- https://cdn.elifesciences.org/articles/76970/elife-76970-fig5-figsupp1-data1-v2.pdf
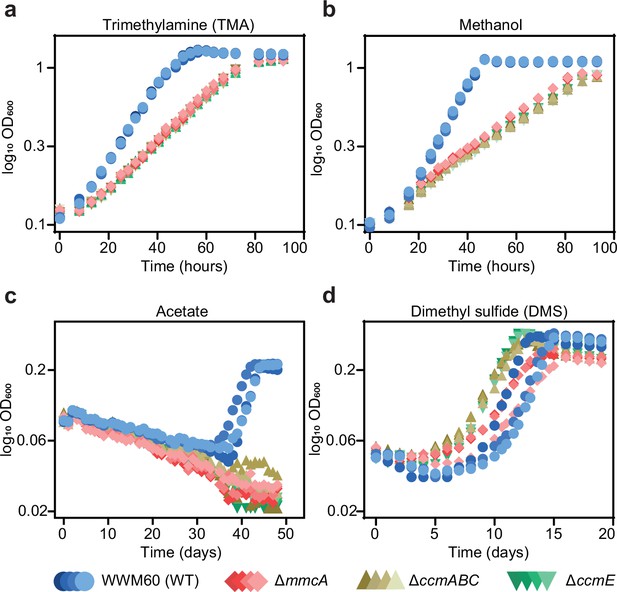
Growth curves of the parent strain (WWM60; referred to as wild-type [WT]) (in blue circles), the ∆mmcA mutant (in red diamonds), the ∆ccmABC mutant (in olive triangles), and the ∆ccmE mutant (in green inverted triangles) in high-salt minimal medium with (a) 50 mM trimethylamine hydrochloride (TMA), (b) 125 mM methanol, (c) 40 mM sodium acetate (acetate), and (d) 20 mM dimethyl sulfide (DMS) as the sole carbon and energy source.
Four replicates were used for growth assays on TMA and methanol and three replicates were used for growth assays on acetate and DMS.
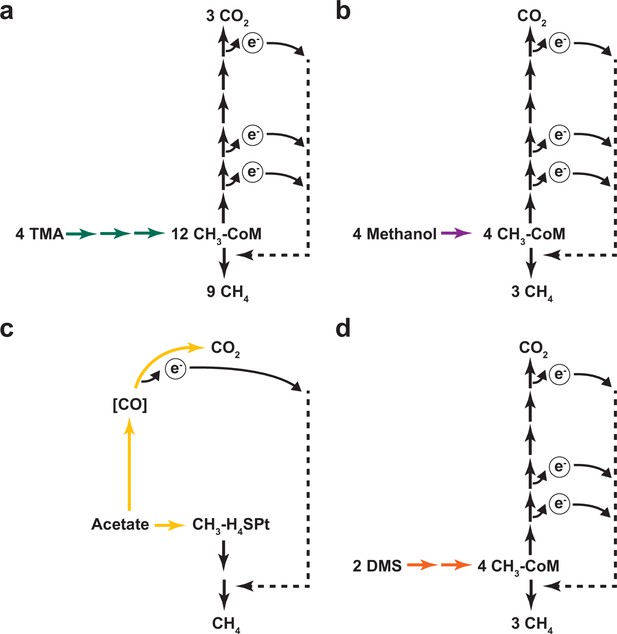
Different modes of methanogenic growth in Methanosarcina acetivorans.
(a) Methanogenic metabolism of trimethylamine (TMA) in Methanosarcina acetivorans. Green arrows represent TMA, dimethylamine (DMA), monomethylamine (MMA) specific methyltransferase reactions that catalyze the transfer of each of the methyl groups in TMA to ultimately form methyl-CoM (CH3-CoM). CH3-CoM undergoes a disproportionation reaction to generate CO2 and CH4 in a 3:1 ratio. (b) Methanogenic metabolism of methanol in M. acetivorans. The purple arrow represents the methanol-specific reaction that catalyzes the transfer of the methyl group to ultimately form methyl-CoM (CH3-CoM). CH3-CoM undergoes a disproportionation reaction to generate CO2 and CH4 in a 3:1 ratio. (c) Methanogenic metabolism of acetate in M. acetivorans. The yellow arrow represents the reaction catalyzed by the acetyl-CoA decarbonylase/synthase (ACDS) enzyme complex that splits acetate into an enzyme-bound carbonyl group and a methyl group that is transferred to the C1 carrier tetrahydrosarcinopterin (H4SPt). Oxidation of the carbonyl group to CO2 produces electrons that can be used to reduce the methyl group to CH4. During acetoclastic methanogenesis, CO2 and CH4 are produced in a 1:1 ratio. (d) Methanogenic metabolism of dimethyl sulfide (DMS) in M. acetivorans. The orange arrows represent DMA and methanethiol (MeSH) specific reactions that catalyzes the transfer of the methyl groups from DMS to ultimately form methyl-CoM (CH3-CoM). CH3-CoM undergoes a disproportionation reaction to generate CO2 and CH4 in a 3:1 ratio.
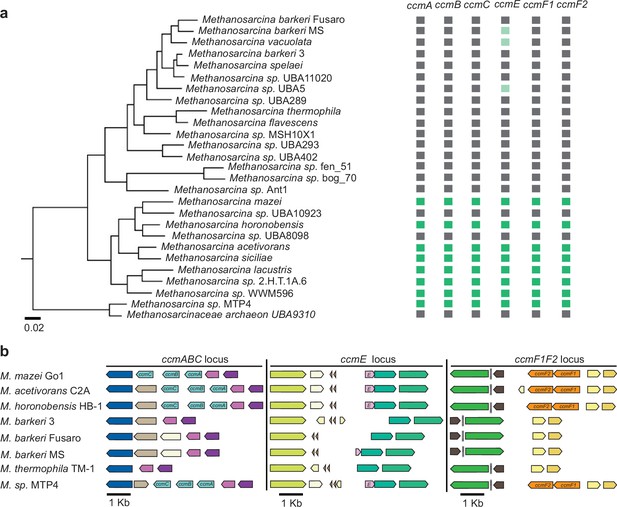
Distribution of the Ccm machinery genes in members of the Methanosarcina Genus.
(a) A phylogenetic tree for strains belonging to the genus Methanosarcina in the Genome Taxonomy Database (GTDB) was obtained from AnnoTree (Mendler et al., 2019). The presence or absence of genes functionally annotated as ccmA, ccmB, ccmC, ccmE, ccmF1, and ccmF2 in the genome of individual strains is shown in dark green (present) or black (absent), respectively. A few strains like M. barkeri MS, M. vacuolata, and M. sp. UBA5 encode a truncated copy of ccmE as indicated in light green. (b) Chromosomal organization of genes surrounding the ccmABC, ccmE, and ccmF1ccmF2 locus in Methanosarcina strains. Genes of the same color (except light yellow) represent members of the same orthologous group. Synteny of the ccmABC, ccmE, and ccmF1ccmF2 chromosomal locus across members of the genus Methanosarcina supports the hypothesis that these genes were present in the last common ancestor and have been lost in several extant lineages.
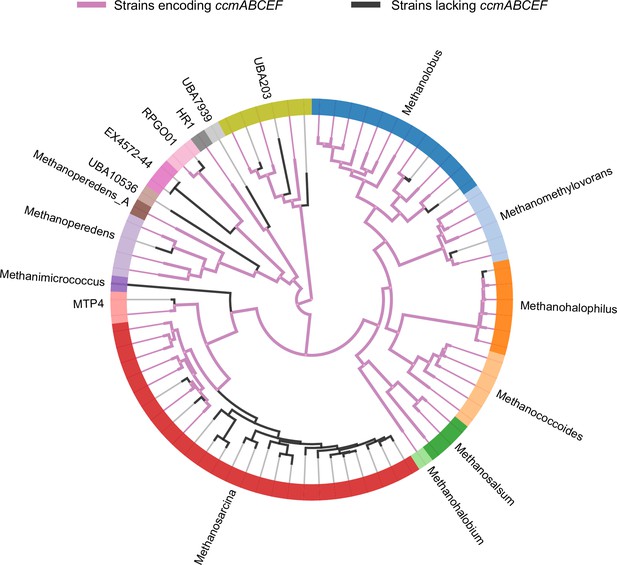
A phylogenomic reconstruction of the relationship between various genera (depicted by different colors along the circumference of the radial tree) within the family Methanosarcinaceae from AnnoTree (Mendler et al., 2019).
Purple lines depict strains that encode homologs of ccmABCEF1F2 in their genome whereas black lines depict strains that lack homologs of ccmABCEF1F2 in their genome. Based on the pattern of distribution of the ccmABCEF1F2 genes, it is likely that these genes were present in the last common ancestor of the Methanosarcinaceae and have been lost in members of various genera including Methanosarcina spp., Methanohalophilus spp., Methanomethylovorans spp., Methanolobus spp., and Methanoperedens spp.
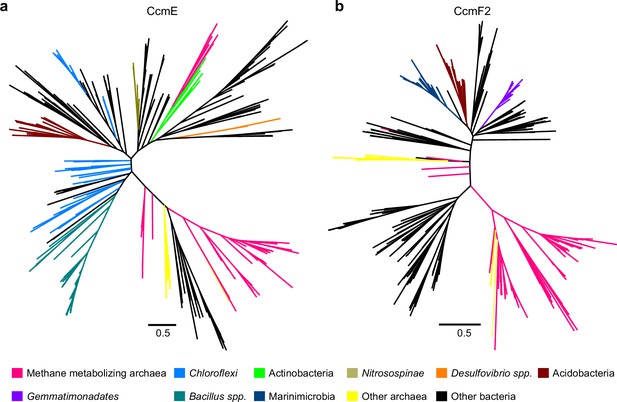
Maximum-likelihood phylogenetic trees of 500 (a) CcmE and (b) CcmF2 sequences obtained from the NCBI non-redundant (nr) protein database using the corresponding sequence from Methanosarcina acetivorans as the search query.
Sequences belonging to certain functional groups or evolutionarily related groups of archaea and bacteria are shown in colors denoted in the legend at the bottom. These gene trees are indicative of multiple horizontal gene transfer (HGT) events between members of the Archaea and Bacteria.
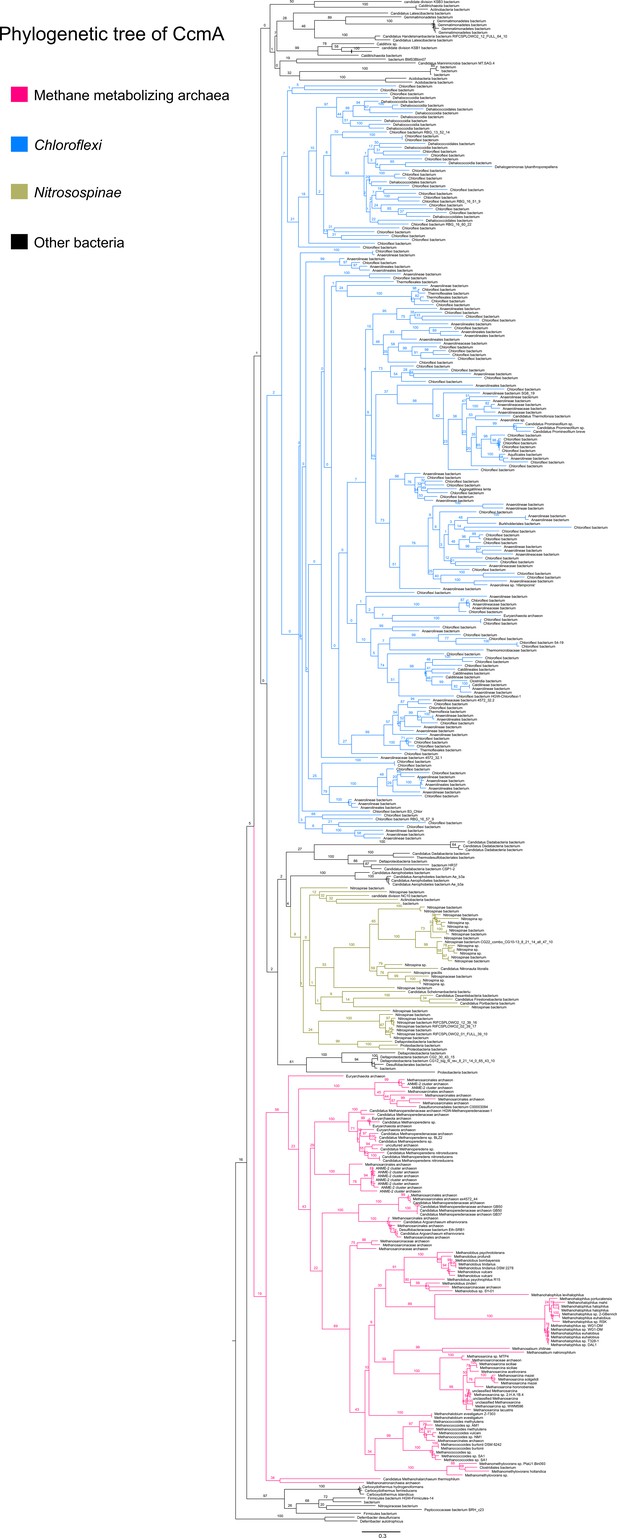
An unrooted maximum-likelihood tree of 500 CcmA sequences retrieved from the NCBI non-redundant protein sequence database using the corresponding sequence from Methanosarcina acetivorans as the search query.
Branch labels indicate support values.
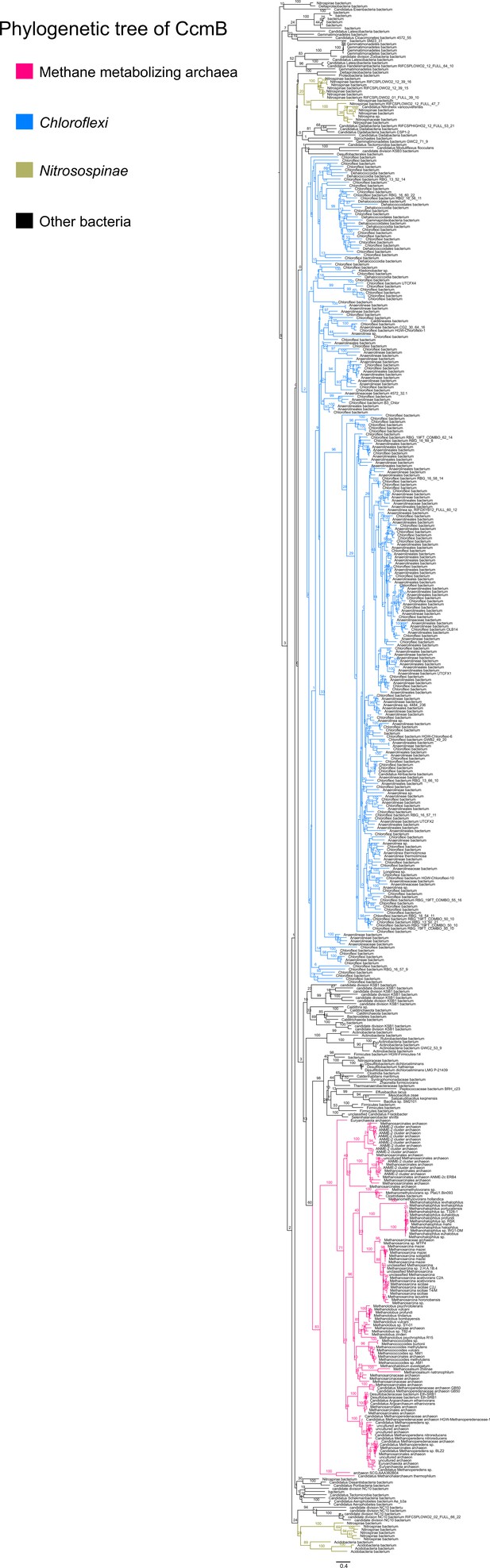
An unrooted maximum-likelihood tree of 500 CcmB sequences retrieved from the NCBI non-redundant protein sequence database using the corresponding sequence from Methanosarcina acetivorans as the search query.
Branch labels indicate support values.
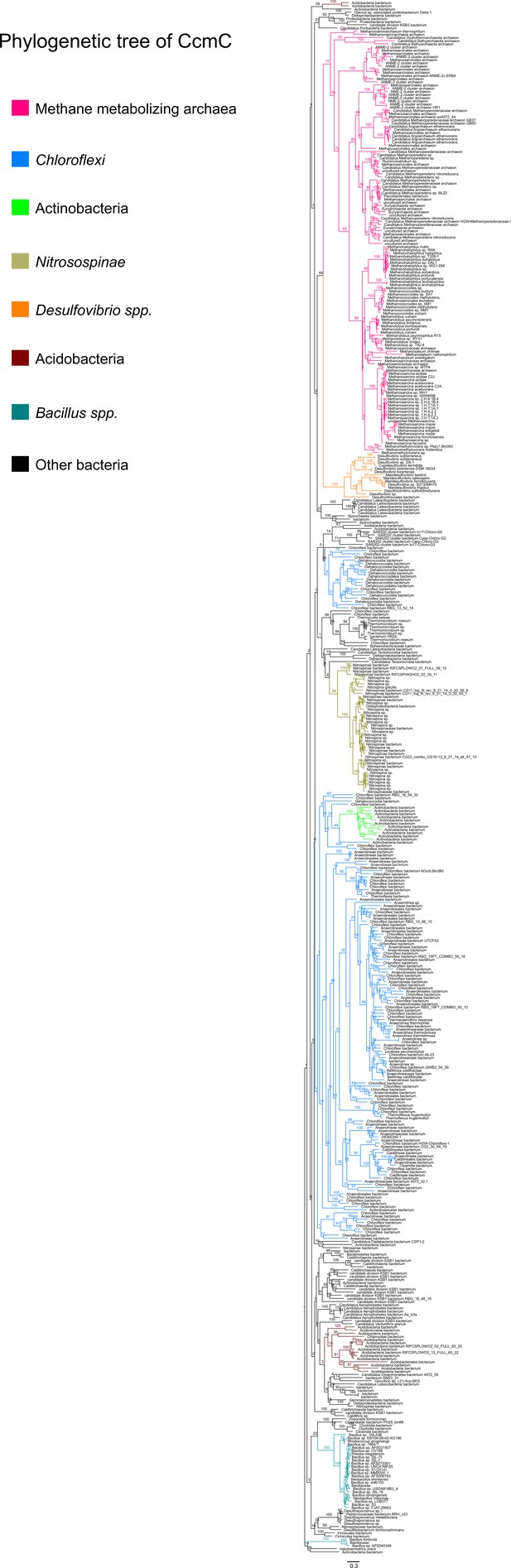
An unrooted maximum-likelihood tree of 500 CcmC sequences retrieved from the NCBI non-redundant protein sequence database using the corresponding sequence from Methanosarcina acetivorans as the search query.
Branch labels indicate support values.
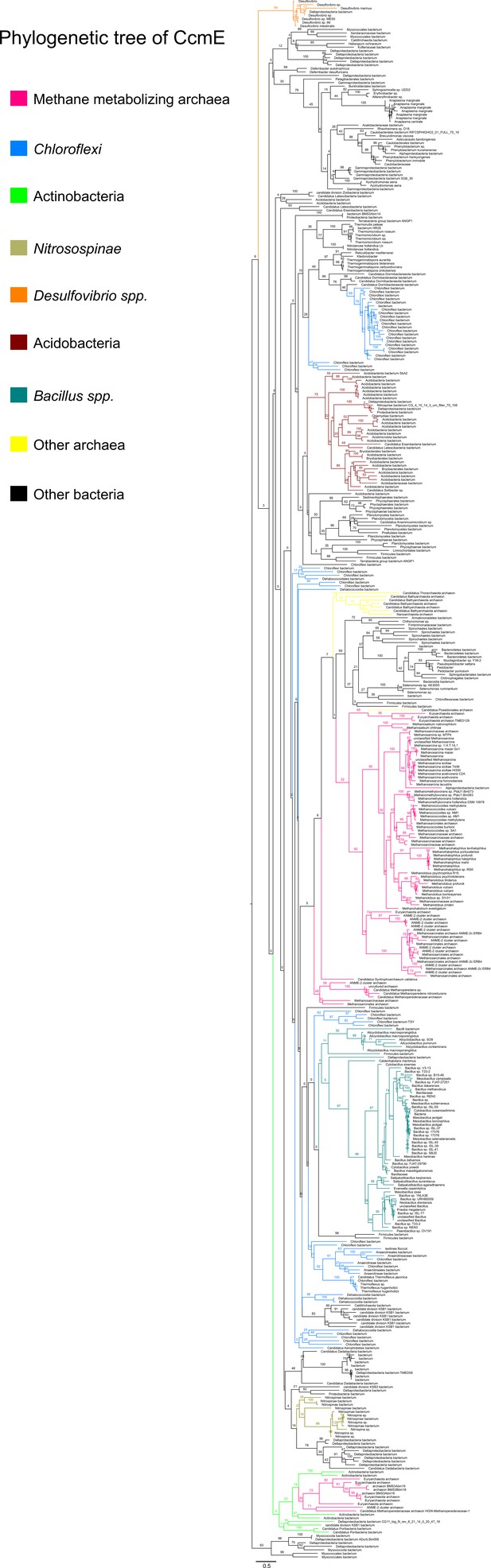
An unrooted maximum-likelihood tree of 500 CcmE sequences retrieved from the NCBI non-redundant protein sequence database using the corresponding sequence from Methanosarcina acetivorans as the search query.
Branch labels indicate support values.
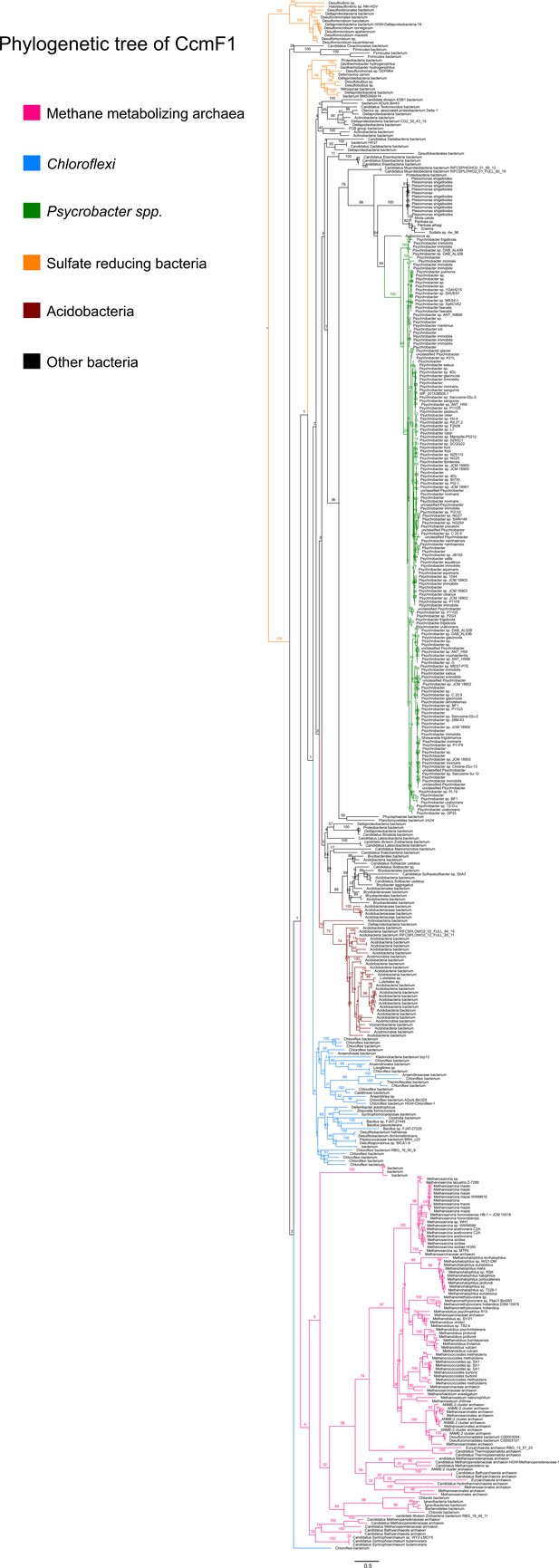
An unrooted maximum-likelihood tree of 500 CcmF1 sequences retrieved from the NCBI non-redundant protein sequence database using the corresponding sequence from Methanosarcina acetivorans as the search query.
Branch labels indicate support values.
Additional files
-
Supplementary file 1
List of mutations in CRISPR-edited mutant strain DDN029 containing a ∆ccmABC in-frame deletion mutation.
- https://cdn.elifesciences.org/articles/76970/elife-76970-supp1-v2.docx
-
Supplementary file 2
Growth data of Methanosarcina acetivorans strains shown in Figure 6.
- https://cdn.elifesciences.org/articles/76970/elife-76970-supp2-v2.docx
-
Supplementary file 3
List of target sequences used in this study.
- https://cdn.elifesciences.org/articles/76970/elife-76970-supp3-v2.docx
-
Supplementary file 4
List of plasmids used in this study.
- https://cdn.elifesciences.org/articles/76970/elife-76970-supp4-v2.docx
-
Supplementary file 5
List of primers used in this study.
- https://cdn.elifesciences.org/articles/76970/elife-76970-supp5-v2.docx
-
Supplementary file 6
List of Methanosarcina strains used in this study.
- https://cdn.elifesciences.org/articles/76970/elife-76970-supp6-v2.docx
-
Transparent reporting form
- https://cdn.elifesciences.org/articles/76970/elife-76970-transrepform1-v2.pdf