On the flexibility of the cellular amination network in E coli
Figures
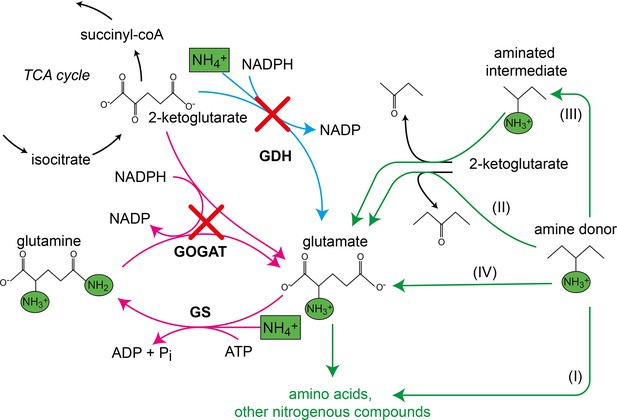
Canonical ammonium assimilation via glutamate dehydrogenase (GDH) (blue arrow) or glutamine synthetase (GS) and glutamine 2-ketoglutarate aminotransferase (GOGAT) (pink arrows).
One pathway for ammonium (green boxes) assimilation is the amination of the tricarboxylic acid (TCA) cycle intermediate 2-ketoglutarate by GDH to form glutamate (blue arrow). A second pathway requires joint action of GS and GOGAT, which first aminate glutamate to form glutamine (GS), which donates one amine to 2-ketoglutarate (GOGAT) to form two glutamate molecules, which further provide amines (green circles) for biosynthesis of amino acids and other nitrogenous compounds (pink arrows). Growth of the glutamate auxotrophic (glut-aux) strain deleted in GDH and GOGAT (red crosses) by a supplemented amine source is possible via the following mechanisms. The amine donor either (I) replaces glutamate as an amine source for the production of amino acids and nitrogenous compounds, (II) donates an amine to 2-ketoglutarate to form glutamate, (III) is converted to an intermediate donating an amine to 2-ketoglutarate, or (IV) is metabolically converted into glutamate. Green arrows indicate these cases.
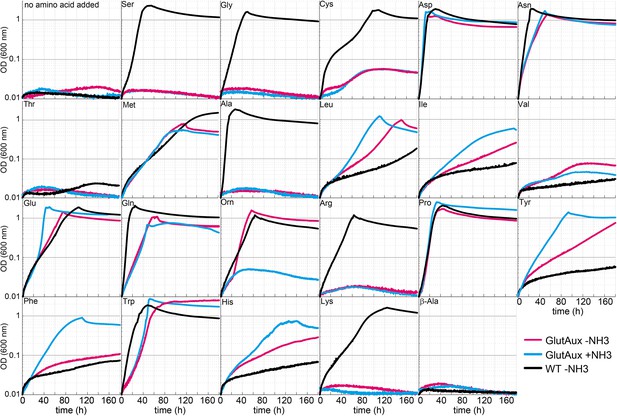
Identification of amino acids that rescue growth of the glutamate auxotrophic (glut-aux) strain.
The glut-aux strain was grown in M9 medium with (blue line) or without ammonium (magenta line) and 20 mM glycerol as carbon source. E. coli wildtype (WT) was grown in M9 medium without ammonium (black line) and 20 mM glycerol as carbon source. 5 mM of the indicated amino acids or no amino acid as negative control were supplemented to test whether they can serve as an amine source (glut-aux strain) or an ammonium source (WT). Data shows representative growth as observed from triplicate repeats with errors <5%.
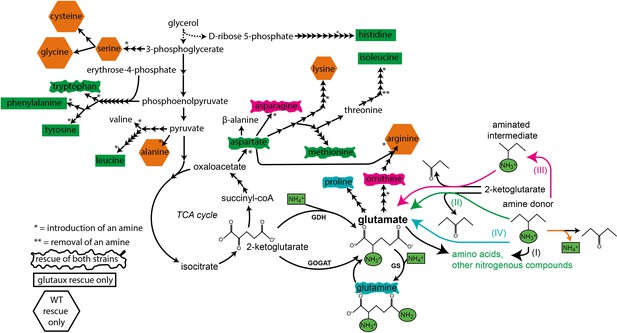
Summary of growth rescue experiment (Figure 2) with wildtype (WT) and glutamate auxotrophic (glut-aux) strain.
Black arrows indicate amino acid biosynthesis (number of steps indicated) from the fed carbon source glycerol. Amine introduction or removal is presented by * or **, respectively. Shapes around the amino acids represent whether both strains (rectangle with wavy borders), only glut-aux (rectangle), or only WT (hexagon) were rescued. Shape color indicates suggested rescue mechanism (indicated on the right; I–IV for glut-aux rescue, and deamination [orange] for WT rescue) based on literature search and findings in this work. Biosynthesis precursors and strain rescue are not correlated. The majority of amino acids expected to serve for direct transamination (II, green) rescued only the glut-aux.
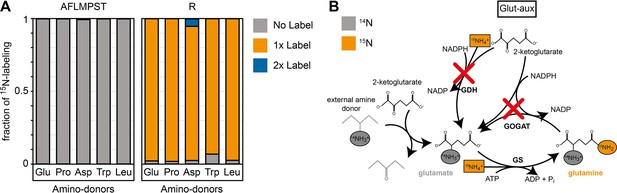
15N labeling confirms amine assimilation from the supplied amino acid in the glutamate auxotrophic (glut-aux) strain.
(A) The glut-aux strain was incubated in 15N-NH4Cl M9 medium with 20 mM glycerol as carbon source. 15N labeling pattern in analyzed proteinogenic amino acids (single letter code) upon feeding with 5 mM of unlabeled amino acids glutamate, proline, aspartate, tryptophan, or leucine as amine donors (three-letter code). The labeling patterns of the amino acids A, F, L, M, P, S, and T were identical to the supplied amino acids and hence only a representative dataset is shown. Data represents means of triplicate measurements with errors <5%. (B) Schematic presentation of expected ammonium incorporation in glut-aux grown on M9 with 15N-NH4Cl with 20 mM glycerol as carbon source and an unlabeled amino acid as ammonium source. Since the genes encoding glutamate dehydrogenase (GDH) and glutamine 2-ketoglutarate aminotransferase (GOGAT) are deleted in the glut-aux (red crosses), the glut-aux thus relies on the provided amino acid for biosynthesis of unlabeled (gray) glutamate. During glutamine biosynthesis, the glut-aux assimilates free ammonium (boxes) supplied as 115N-NH4Cl to form once-labeled glutamine that is used for carbamoyl-phosphate biosynthesis and thus results in once-labeled arginine.
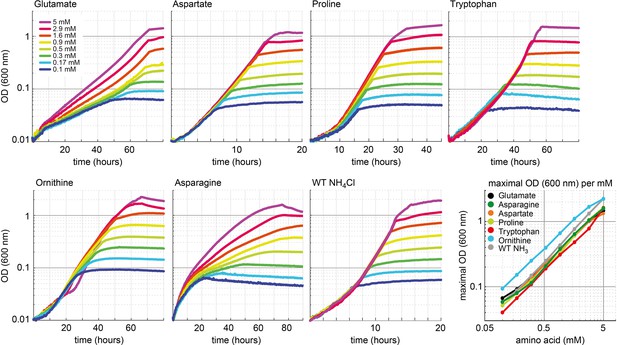
Growth dependency of the glutamate auxotrophic (glut-aux) strain on amino acid concentration.
Cells were grown in ammonium-free M9 medium with 20 mM glycerol and the indicated concentrations of the amino acids glutamate, aspartate, proline, tryptophan, ornithine, and asparagine. As a comparison, wildtype (WT) was grown in ammonium-free M9 medium with 20 mM glycerol but with NH4Cl concentrations similar to the amino acid concentrations used. Data shows representative growth as observed from triplicate repeats with errors <5%.
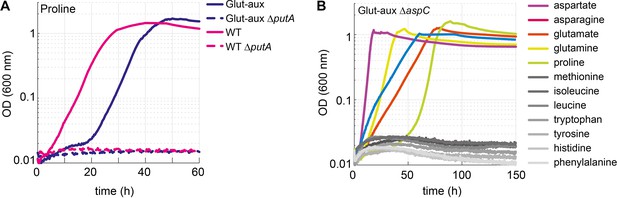
Proline is converted to glutamate to serve as an amine source.
Aspartate transaminase (AspC) is responsible for the utilization of several amino acids. (A) Deletion of putA abolished growth with proline as an amine source in the glutamate auxotrophic (glut-aux) strain and as a nitrogen source in the wildtype (WT). (B) Deletion of aspC eliminates growth of the glut-aux with methionine, leucine, isoleucine, histidine, tyrosine, tryptophan, and phenylalanine as amine donors (gray lines). Experiments were carried out in M9 with ammonium containing 20 mM glycerol and 5 mM of the indicated amine sources. Data represents means of triplicates with <5% variation.
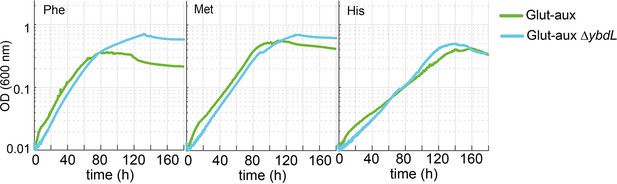
Deletion of ybdL does not alter growth of the glutamate auxotrophic (glut-aux) strain with phenylalanine, methionine, and histidine as amine sources.
Experiments were carried out in M9 with ammonium containing 20 mM glycerol and 5 mM of the indicated amine sources. Data represents means of triplicates with <5% variation.
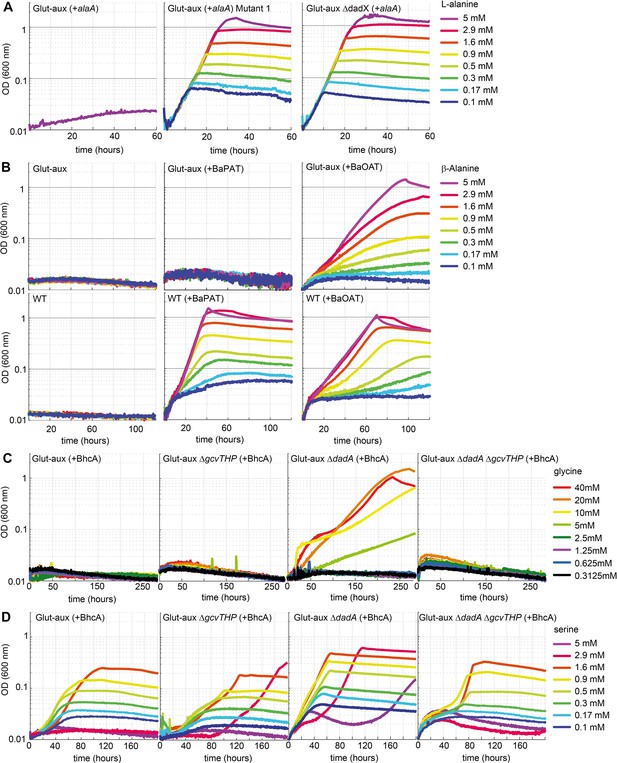
Glutamate auxotrophic (glut-aux) strains engineered to use alanine, β-alanine, glycine, and serine as an amine source.
(A) DadX mutation or deletion and alanine transaminase overexpression allows alanine utilization as an amine source. Growth of the glut-aux strains on M9 medium with ammonium and 20 mM glycerol with 5 mM alanine as an amine donor. (B) Overexpression of β-alanine-2-ketoglutarate aminotransferase (BaOAT) enables amine donation from β-alanine in the glut-aux strain. Glut-aux strain and wildtype (WT) overexpressing β-alanine-pyruvate aminotransferase (BaPAT) or β-alanine-2-ketoglutarate aminotransferase (BaOAT) where grown in media containing no N-source (WT) or ammonium chloride (glut-aux), 20 mM glycerol, and the indicated β-alanine concentrations. (C) DadA deletion and BhcA overexpression allow glycine utilization as an amine donor. Growth of the glut-aux strains + BhcA on M9 with ammonium and 20 mM glycerol with indicated glycine concentrations. Data represents triplicate measurements with <5% variation. (D) BhcA overexpression allows use of serine as an amine source, which is improved by additional deletion of dadA. Growth of the glut-aux strains + BhcA on M9 with ammonium and 20 mM glycerol with indicated serine concentrations. All data represents triplicate measurements with <5% variation.
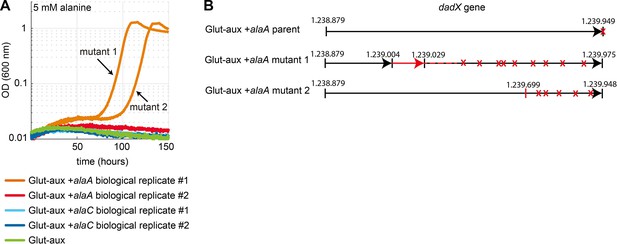
Mutations in the dadX locus allow transaminase-based use of alanine as an amine source.
(A) Growth of two biological replicates of glutamate auxotrophic (glut-aux) + alaA and glut-aux + alaC as well as glut-aux on M9 with ammonium and 20 mM glycerol as carbon source and 5 mM alanine. This experiment was carried out in technical duplicates (all shown) in different wells, of which both from glut-aux + alaA biological replicate 1 evolved towards growth on alanine. Isolated cultures of mutant 1 and 2 were analyzed by whole-genome sequencing. (B) Schematic presentation of 26 bp duplication (red) found in the dadX gene of the glut-aux + alaA mutant 1 and 1 bp deletion in the dadX gene glut-aux + AlaA mutant 2 in comparison to the glut-aux + alaA parent. Both mutations cause stop-codons (red crosses) within the dadX gene reading frame.
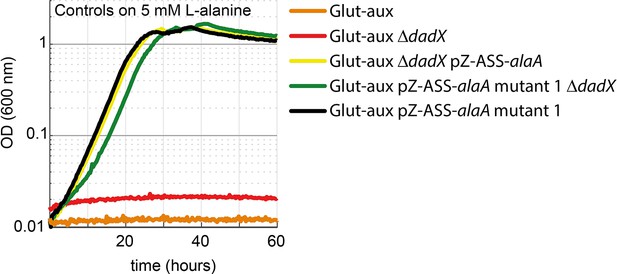
DadX mutation or deletion and alanine transaminase overexpression allows alanine utilization as an amine source.
Growth of the glutamate auxotrophic (glut-aux) strains on M9 with ammonium and 20 mM glycerol with 5 mM alanine as an amine donor. Strains not overexpressing alanine transaminase cannot utilize alanine as an amine source. All data represents triplicate measurements with <5% variation.
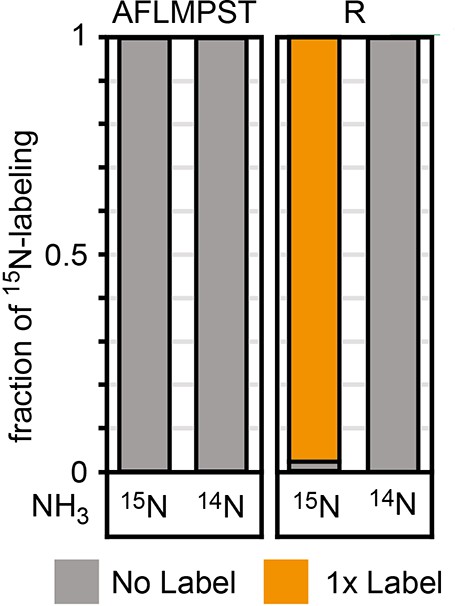
15N labeling experiments confirm incorporation of the amino group of β-alanine into proteinogenic amino acids (single-letter code).
The glutamate auxotrophic (glut-aux) + β-alanine-2-ketoglutarate transaminase (BaOAT) strain was grown in medium containing 20 mM glycerol with 5 mM β-alanine and either 14NH4 or 15NH4. Data represents means of three independent experiments.
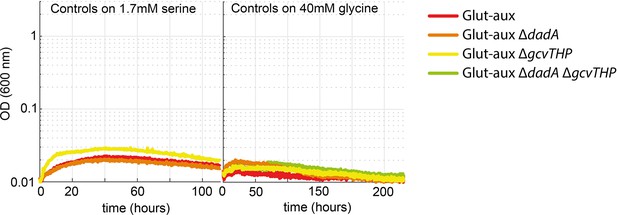
Transaminase overexpression is essential for the use of serine or glycine as an amine donor in the glutamate auxotrophic (glut-aux) strain.
Glut-aux strains not overexpressing BhcA were incubated in M9 with ammonium and 20 mM glycerol and 1.7 mM serine or 40 mM glycine as an amine donor. No growth was obtained, indicating the need for BhcA overexpression for the utilization of glycine or serine as amine sources. All data represents triplicate measurements with <5% variation.
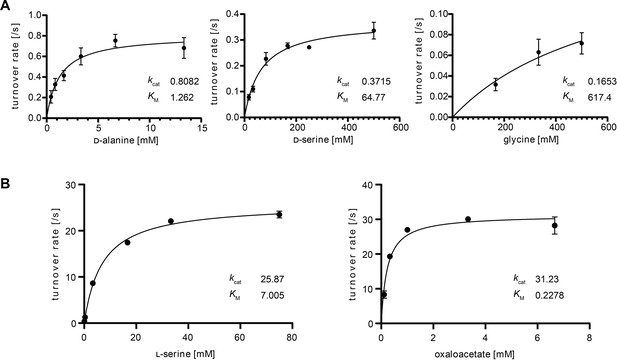
Michaelis–Menten kinetics of DadA and BhcA for the selected substrates.
(A) DadA turnover rate with d-alanine (left), d-serine (middle), and glycine (right) measured in a dichlorophenolindophenole (DCPIP)-coupled assay. (B) BhcA turnover rate with serine (left) and oxaloacetate (right). To saturating concentrations of oxaloacetate (left) or serine (right), different concentrations of the respective other substrate were added in an assay coupling BhcA-mediated hydroxypyruvate formation to NADPH-dependent hydroxypyruvate reduction catalyzed by GhrA. Data are shown from n = 3 independent experiments at different substrate concentrations. Kinetic parameters are listed in Supplementary file 3 (DadA) and Supplementary file 4 (BhcA).
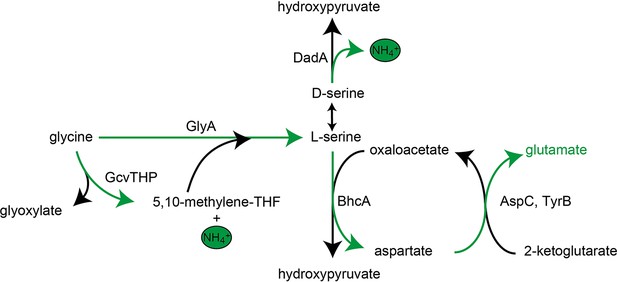
Schematic presentation of glycine and serine utilization by the glutamate auxotrophic (glut-aux) strain ΔdadA expressing BhcA.
Glycine is converted to serine by combined activity of GlyA and GcvTHP, which is then substrate for BhcA in a transamination reaction with oxaloacetate. The formed aspartate allows glutamate formation via native transaminases. DadA promiscuously deaminating serine to form hydroxypyruvate might be an intracellular serine sink and needed to be deleted for optimal growth of the glut-aux + BhcA with glycine or serine as an amine source. Notably, the BhcA substrate l-serine would first need to be converted to d-serine, for example, by promiscuously acting alanine racemase Alr, to be substrate for DadA. Green arrows indicate the transfer of ammonium.
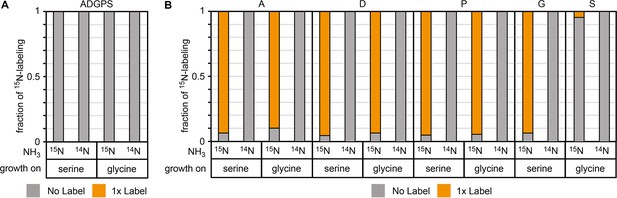
15N labeling confirms amine donation from serine or glycine in glutamate auxotrophic (glut-aux) ΔdadA + BhcA.
(A) Glut-aux ΔdadA + BhcA grown on 15N-NH3Cl M9 medium or 14N-NH3Cl M9 medium with 20 mM glycerol as carbon source. (B) Wildtype (WT) grown on 15N-NH3Cl M9 medium or 14N-NH3Cl M9 medium with 20 mM glycerol as carbon source. For both strains, 15N labeling in amino acids (single-letter code) was analyzed upon feeding with 1.7 mM of unlabeled serine or 20 mM of unlabeled glycine. Data represents means of triplicate measurements with errors <5%.
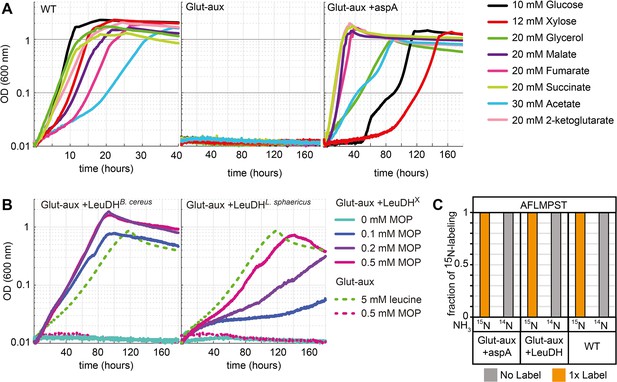
Aspartate ammonia lyase or leucine dehydrogenase (LeuDH) can replace glutamate-based ammonia assimilation to fix all ammonium for cell growth.
(A) Growth of wildtype (WT), glutamate auxotrophic strain (glut-aux), and glut-aux + aspA on M9 with ammonium and indicated carbon sources. Overexpression of aspartate ammonia lyase allows the glut-aux to assimilate free ammonium via fumarate amination. (B) Growth of glut-aux and glut-aux + LeuDH from Bacillus cereus or Lysinibacillus sphaericus on M9 with ammonium, 20 mM glycerol as carbon source and the indicated additional substrates. Overexpression of LeuDH allows the glut-aux to assimilate free ammonium from the medium in presence of 4-methyl-2-oxopentanoate (MOP). (C) Representative dataset for nitrogen labeling results from glut-aux + AspA, glut-aux + LeuDH, and WT strains. Cells were grown either with 15NH4 or 14NH4 and 20 mM succinate (glut-aux + AspA), 20 mM glycerol + 0.5 mM MOP (glut-aux + LeuDH, from B. cereus or L. sphaericus), or 20 mM glycerol (WT). All strains were grown in M9 medium with ammonium. Data represents triplicate experiments with <5% variation.
Tables
Strains and plasmids used in this study.
Name | Description/deletions | References |
---|---|---|
E. coli strains | ||
DH5α | Cloning of overexpression constructs | |
SIJ488 | WT, integrated λ-red recombinase and flippase | Jensen et al., 2015 |
Glut-aux | ΔgdhA ΔgltBD | This study |
Glut-aux ΔybdL | ΔgdhA ΔgltBD ΔybdL | This study |
Glut-aux ΔputA | ΔgdhA ΔgltBD ΔputA | This study |
ΔputA | ΔputA | This study |
Glut-aux ΔaspC | ΔgdhA ΔgltBD ΔaspC | This study |
Glut-aux ΔdadX | ΔgdhA ΔgltBD ΔdadX | This study |
Glut-aux ΔdadA | ΔgdhA ΔgltBD ΔdadA | This study |
Glut-aux Δgcv | ΔgdhA ΔgltBD ΔgcvTHP | This study |
Glut-aux Δgcv ΔdadA | ΔgdhA ΔgltBD ΔgcvTHP ΔdadA | This study |
JW0999 | KEIO ΔputA | Baba et al., 2006 |
Plasmids | ||
ASS | Overexpression plasmid with p15A origin, streptomycin resistance, constitutive strong promoter | Wenk et al., 2018 |
ASS-aspA | ASS backbone for overexpression of aspA from E. coli | This study |
ASS-alaA | ASS backbone for overexpression of alaA from E. coli | This study |
ASS-alaC | ASS backbone for overexpression of alaC from E. coli | This study |
ASS-leuDHB. cereus | ASS backbone for overexpression of leucine dehydrogenase from Bacillus cereus | This study |
ASS-leuDHL. sphaericus | ASS backbone for overexpression of leucine dehydrogenase from Lysinibacillus sphaericus | This study |
ASS-BAPAT | ASS backbone for overexpression of β-alanine-pyruvate-aminotransferase from Pseudomonas aeruginosa | This study |
ASS-BAOAT | ASS backbone for overexpression of β-alanine-2-ketoglutarate-aminotransferase from Saccharomyces kluyveri | This study |
ASS-BhcA | ASS backbone for overexpression of glycine-oxaloacetate aminotransferase from Paracoccus denitrificans | This study |
Additional files
-
Supplementary file 1
Doubling time of glutamate auxotrophic (glut-aux) and wildtype on tested amino acids.
Strains were grown over 170 hr in minimal medium with ammonium (glut-aux) and without ammonium (glut-aux, wildtype). 20 mM glycerol was used as the carbon source and 5 mM amino acid as nitrogen source. Maximal doubling time in a window of 6 hr was calculated using MATLAB and is indicated with standard error. An OD600 of 0.1 was the threshold for defining growth (above OD600 of 0.1) or no growth.
- https://cdn.elifesciences.org/articles/77492/elife-77492-supp1-v2.docx
-
Supplementary file 2
Mutations found in glutamate auxotrophic (glut-aux) + alaA mutants 1 and 2.
After sequencing the genomes of two independently isolated glut-aux + alaA mutants and the glut-aux + alaA parent, the results were mapped against the E. coli MG1655 reference genome (GenBank accession no. U00096.3) using Breseq. Mutations occurring in the mutants and not the parent are listed.
- https://cdn.elifesciences.org/articles/77492/elife-77492-supp2-v2.docx
-
Supplementary file 3
Kinetics of DadA with d-alanine and glycine.
Enzyme activity was determined in a dichlorophenolindophenole (DCPIP)-coupled assay with various substrate concentrations and 60 µg protein for measurements with d-alanine and 280 µg protein for measurements with glycine. Data are mean ± SE.
- https://cdn.elifesciences.org/articles/77492/elife-77492-supp3-v2.docx
-
Supplementary file 4
Kinetics of BhcA with l-serine and oxaloacetate.
Enzyme activity was determined in an assay coupling BhcA-dependent l-serine transamination to NADPH-dependent hydroxypyruvate reduction by GhrA with varying concentrations of l-serine or oxaloacetate for the respective activity measurements. Data are mean ± SE.
- https://cdn.elifesciences.org/articles/77492/elife-77492-supp4-v2.docx
-
Supplementary file 5
Oligonucleotide primers used.
‘KO’ primers were used to amplify the knockout kanamycin (Km) cassette from pKD4 with 50 bp gene-specific upstream and downstream sequences. ‘KO-Ver’ primers (knockout verification) were used to verify gene replacement by Km resistance cassette and cassette removal by flippase. External and internal primers were used to verify successful removal of the gene from the genome.
- https://cdn.elifesciences.org/articles/77492/elife-77492-supp5-v2.docx
-
Transparent reporting form
- https://cdn.elifesciences.org/articles/77492/elife-77492-transrepform1-v2.pdf