Metabolic clogging of mannose triggers dNTP loss and genomic instability in human cancer cells
Figures
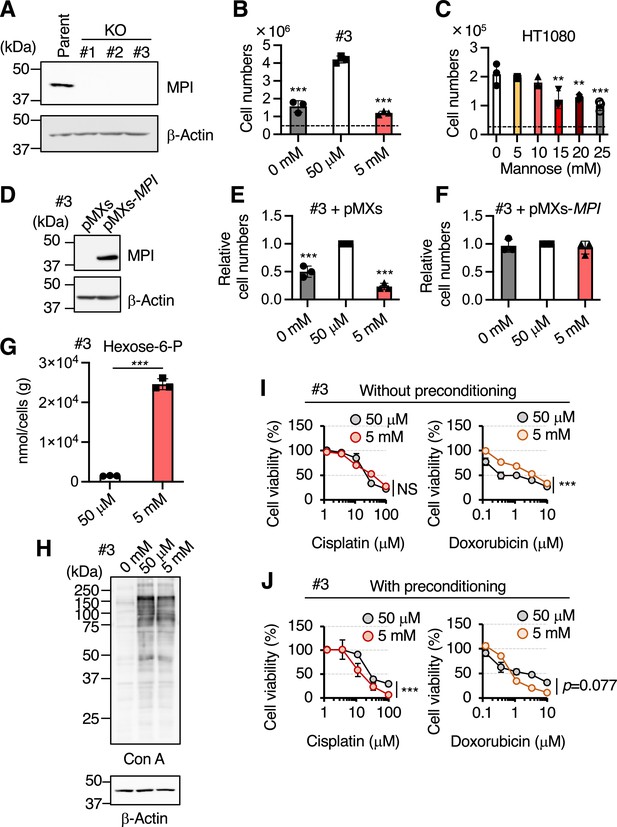
The induction of honeybee syndrome suppresses cell proliferation and increases chemosensitivity.
(A) Western blot analysis of HT1080 (parent) and mannose phosphate isomerase knockout (MPI-KO) HT1080 (KO, clone #1–3) cells. The blots have been vertically flipped for presentation purpose. (B, C) The cell numbers of MPI-KO HT1080 (#3, B) and the parental HT1080 (C) cells after 48 hr incubation in culture medium supplemented with mannose at the indicated concentrations. A dashed line indicates the cell numbers at seeding. (D) Western blot analysis of MPI-KO HT1080 (#3) cells retrovirally transduced with empty vector (pMXs) or human MPI gene (pMXs-MPI). (E, F) The relative cell numbers of MPI-KO HT1080 (#3) cells retrovirally transduced with empty vector (pMXs, E) or human MPI gene (pMXs-MPI, F) after 48 hr incubation under mannose-starved (0 mM), mannose-unchallenged (50 μM), or mannose-challenged (5 mM) conditions. (G) The quantification of hexose-6-P in MPI-KO HT1080 (#3) cells cultured in the presence of 50 μM or 5 mM mannose. (H) Lectin blot and western blot analyses of MPI-KO HT1080 (#3) cells cultured as in (B). Con A, concanavalin A lectin. (I) Cell viability assay in MPI-KO HT1080 (#3) cells co-treated with mannose (50 μM or 5 mM) and DNA replication inhibitors (cisplatin or doxorubicin) for 24 hr (I, without preconditioning), or preconditioned with mannose (50 μM or 5 mM) for 24 hr, followed by incubation with the DNA replication inhibitors for an additional 24 hr in the presence of the same concentrations of mannose used for preconditioning (J, with preconditioning). Data represent the mean ± SD; n = 3 independent experiments. *p<0.05, **p<0.01, and ***p<0.001, NS, not significant, one-way ANOVA with post hoc Dunnett’s test (B, C, E, F) Welch’s t-test (G), or two-way ANOVA with post hoc Bonferroni’s test (I, J).
-
Figure 1—source data 1
Original blot images depicting cropped regions for Figure 1A.
- https://cdn.elifesciences.org/articles/83870/elife-83870-fig1-data1-v1.zip
-
Figure 1—source data 2
Original blot images depicting cropped regions for Figure 1D.
- https://cdn.elifesciences.org/articles/83870/elife-83870-fig1-data2-v1.zip
-
Figure 1—source data 3
Original blot images depicting cropped regions for Figure 1H.
- https://cdn.elifesciences.org/articles/83870/elife-83870-fig1-data3-v1.zip
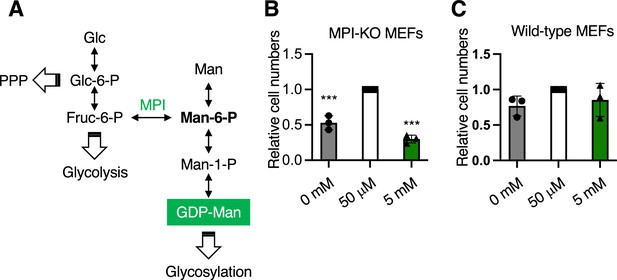
Mannose auxotrophy and sensitivity in mannose phosphate isomerase knockout (MPI-KO) mouse embryonic fibroblasts (MEFs) and wild-type MEFs.
(A) Metabolic pathways for glucose and mannose. PPP, the pentose phosphate pathway; Glc, glucose; Glc-6-P, glucose-6-phosphate; Fruc-6-P, fructose-6-phosphate; Man-6-P, mannose-6-phosphate; Man-1-P, mannose-1-phosphate; Man, mannose; GDP-Man, guanosine diphosphate-mannose. (B, C) The relative numbers of MPI-KO MEFs (B) and wild-type MEFs (C) after 48 hr incubation in culture medium supplemented with mannose at the indicated concentrations. Data represent the mean ± SD; n = 3 independent experiments. ***p<0.001, one-way ANOVA with post hoc Dunnett’s test (B, C).
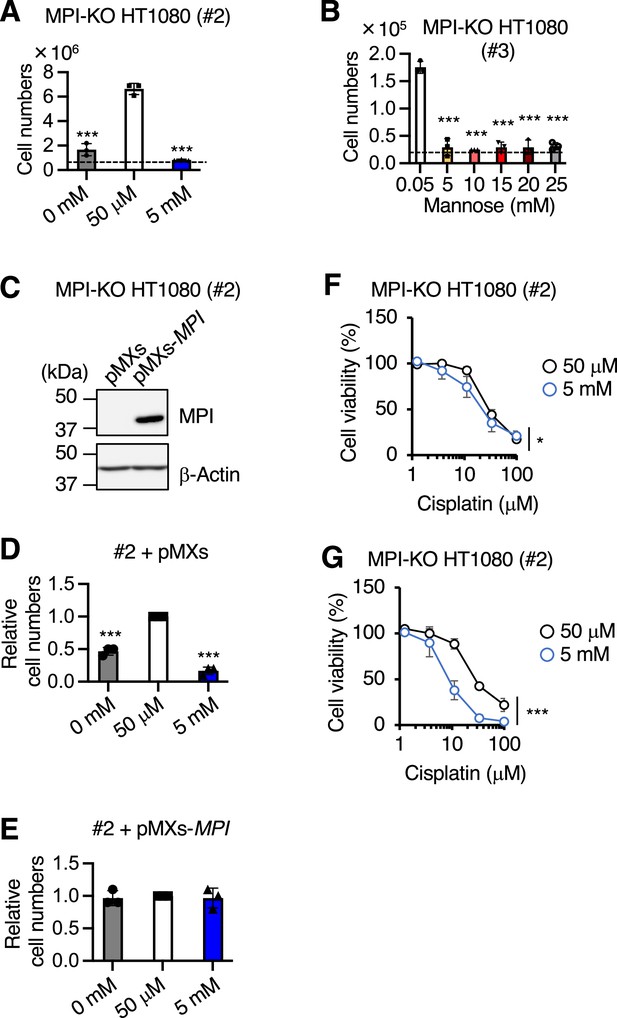
Establishment of mannose phosphate isomerase knockout (MPI-KO) HT1080 cells.
(A, B) The numbers of MPI-KO HT1080 (#2 [A] or #3 [B]) cells after 48 hr incubation in culture medium under the indicated conditions. A dashed line indicates the cell numbers at seeding. (C) Western blot analysis of MPI-KO HT1080 (#2) cells retrovirally transduced with empty vector (pMXs) or human MPI gene (pMXs-MPI). (D, E) The relative numbers of MPI-KO HT1080 (#2) cells retrovirally transduced with empty vector (pMXs, D) or human MPI gene (pMXs-MPI, E) after 48 hr incubation in culture medium supplemented with mannose at the indicated concentrations. (F, G) Cell viability assay in MPI-KO HT1080 (#2) cells co-treated with mannose (50 μM or 5 mM) and cisplatin for 24 hr (F), or preconditioned with mannose (50 μM or 5 mM) for 24 hr, followed by incubation with cisplatin for an additional 24 hr in the presence of the same concentrations of mannose used for preconditioning (G). Data represent the mean ± SD; n = 3 independent experiments. *p<0.05 and ***p<0.001, one-way ANOVA with post hoc Dunnett’s test (A, B, D, E) or two-way ANOVA with post hoc Bonferroni’s test (F, G).
-
Figure 1—figure supplement 2—source data 1
Original blot images depicting cropped regions for Figure 1—figure supplement 2C.
- https://cdn.elifesciences.org/articles/83870/elife-83870-fig1-figsupp2-data1-v1.zip
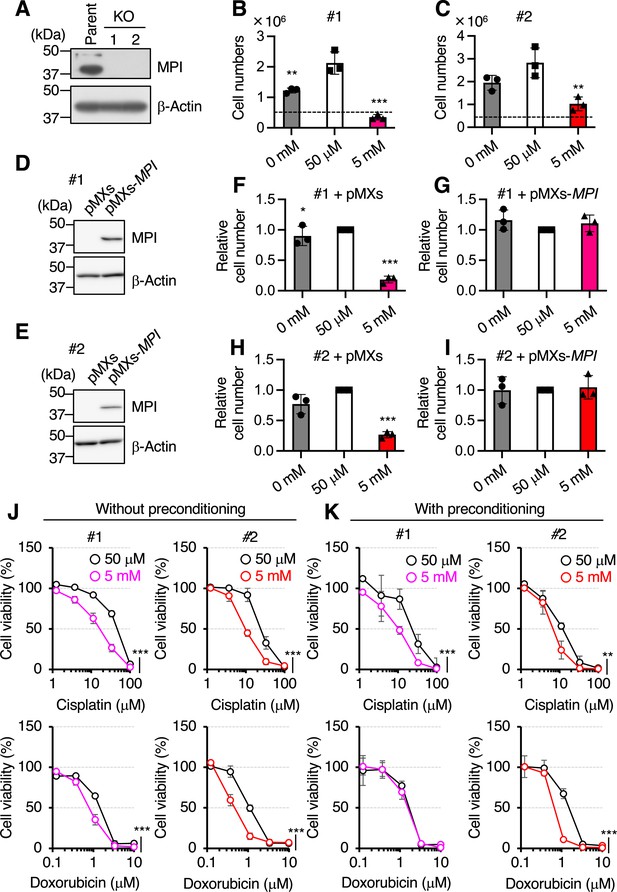
Establishment of mannose phosphate isomerase knockout (MPI-KO) HeLa cells.
(A) Western blot analysis of HeLa (parent) cells and MPI-KO HeLa (KO, clones #1 and #2) cells. (B, C) The numbers of MPI-KO HeLa (#1 [B] and #2 [C]) cells after 48 hr incubation under the indicated conditions. (D, E) Western blot analysis of MPI-KO HeLa (#1 [D] and #2 [E]) cells retrovirally transduced with empty vector (pMXs) or human MPI gene (pMXs-MPI). (F–I) The relative numbers of MPI-KO HeLa (#1 [F, G]; and #2 [H, I]) cells retrovirally transduced with empty vector (pMXs, F and H) or human MPI gene (pMXs-MPI, G and I) after 48 hr incubation under the indicated conditions. (J, K) Cell viability assay in MPI-KO HeLa (#1 and #2) cells co-treated with mannose (50 μM or 5 mM) and DNA replication inhibitors (cisplatin or doxorubicin) for 24 hr (without preconditioning, J), or preconditioned with mannose (50 μM or 5 mM) for 24 hr, followed by incubation with the DNA replication inhibitors for an additional 24 hr in the presence of the same concentrations of mannose used for preconditioning (with preconditioning, K). Data represent the mean ± SD; n = 3 independent experiments. *p<0.05, **p<0.01, and ***p<0.001, one-way ANOVA with post hoc Dunnett’s test (B, C, F–I) or two-way ANOVA (J, K).
-
Figure 1—figure supplement 3—source data 1
Original blot images depicting cropped regions for Figure 1—figure supplement 3A.
- https://cdn.elifesciences.org/articles/83870/elife-83870-fig1-figsupp3-data1-v1.zip
-
Figure 1—figure supplement 3—source data 2
Original blot images depicting cropped regions for Figure 1—figure supplement 3D.
- https://cdn.elifesciences.org/articles/83870/elife-83870-fig1-figsupp3-data2-v1.zip
-
Figure 1—figure supplement 3—source data 3
Original blot images depicting cropped regions for Figure 1—figure supplement 3E.
- https://cdn.elifesciences.org/articles/83870/elife-83870-fig1-figsupp3-data3-v1.zip
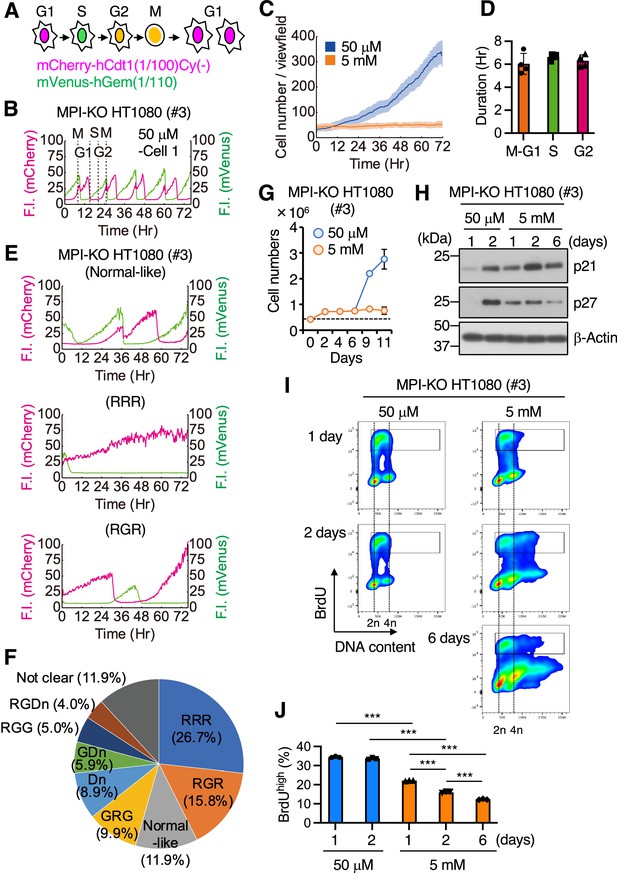
Mannose challenge generates slow-cycling cells.
(A) Schematic representation of cell cycle progression (G1, S, G2, and M phases) visualized by Fucci(CA). Magenta, green, and yellow indicate the expression of mCherry-hCdt1(1/100)Cy(−), mVenus-hGem(1/110), and both, respectively. (B) A representative Fucci(CA) signal profile in mannose phosphate isomerase knockout (MPI-KO) HT1080 cells under the mannose-unchallenged conditions (50 μM). Fluorescent intensity (F.I.) for mCherry (left y-axis) and mVenus (right y-axis) is shown by magenta and green, respectively. Individual phases of the cell cycle (G1, S, G2, and M) are demarcated by dashed lines. (C) The number of MPI-KO HT1080 cells during the time-lapse imaging under mannose-unchallenged (50 μM, blue) and mannose-challenged (5 mM, orange) culture conditions. (D) The durations of M–G1 (the sum of both phases), S, and G2 phases (n=1109 cells) in MPI-KO HT1080 cells under mannose-unchallenged conditions (50 μM). (E) Representative Fucci(CA) signal profiles of MPI-KO HT1080 cells cultured under mannose-challenged conditions for 76 hr. The order of expression of mCherry-hCdt1(1/100)Cy(−) (denoted by R) and mVenus- hGem(1/110) (denoted by G) was used to classify the Fucci profiles and the classification is indicated in parentheses. See also Figure 2—figure supplement 1. (F) The proportion of Fucci(CA) signal profiles of the mannose-challenged MPI-KO HT1080 cells. R, mCherry-hCdt1(1/100)Cy(−) positive; G, mVenus-hGem(1/100) positive; Dn, double negative for Fucci indicators. One hundred cells were visually inspected for the classification and the cells that could not be classified were categorized as ‘not clear.’ See also Figure 2—figure supplement 1. (G) The number of MPI-KO HT1080 (#3) cells. The cells were cultured in the presence of 5 mM mannose for 6 d, and they were further cultured in the presence of 50 μM or 5 mM mannose for 5 d. A dashed line indicates the cell numbers at seeding. (H) Western blot analysis of MPI-KO HT1080 (#3) cells cultured in the presence of 50 μM or 5 mM mannose for the indicated time. (I) Flow cytometry for BrdU and DNA content (Hoechst33342) in MPI-KO HT1080 (#3) cells cultured in the presence of 50 μM or 5 mM mannose for the indicated time. The cells were labeled with 10 μM BrdU for 1 hr before harvest. The highly replicating cells (BrdUhigh) were annotated with black boxes. (J) The percentage of BrdUhigh cells in (I) (in the gated populations). Total numbers of single cells were set to 100%. Data represent the mean ± SD; n = 4 independent fields (C) and n = 3 independent experiments (G, J). ***p<0.001, one-way ANOVA with post hoc Tukey’s test (J).
-
Figure 2—source data 1
Original blot images depicting cropped regions for Figure 2H.
- https://cdn.elifesciences.org/articles/83870/elife-83870-fig2-data1-v1.zip
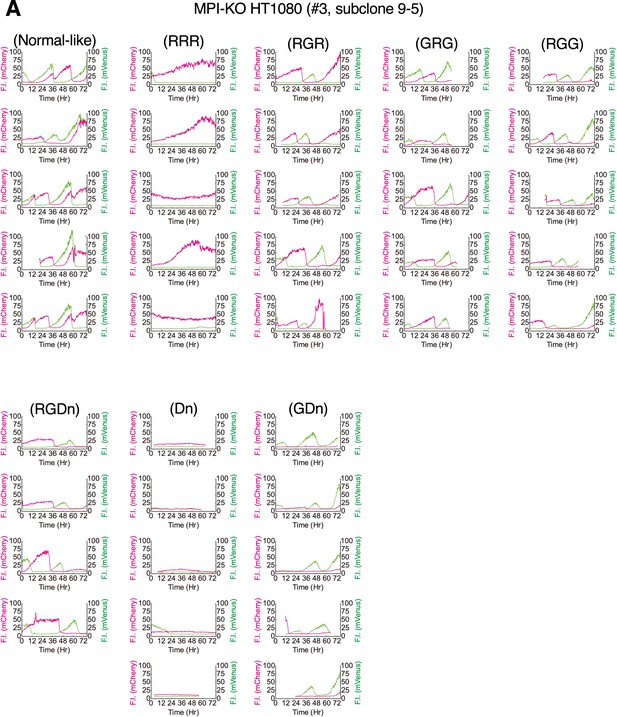
The classification of Fucci profiles in mannose phosphate isomerase knockout (MPI-KO) HT1080 cells cultured under mannose-challenged conditions.
(A) Fluorescent intensity (F.I.) for mCherry (left y-axis) and mVenus (right y-axis) is shown in magenta and green, respectively. The order of expression of mCherry-hCdt1(1/100)Cy(–) (denoted by R) and mVenus-hGem(1/110) (denoted by G) was used to classify the Fucci profiles and the classification is indicated in parentheses. Dn, double negative for Fucci indicators. 100 cells were visually inspected for the classification and five examples are shown for each category, except for RGDn (n = 4 in total). Cells that could not be classified visually were categorized as ‘not clear’.
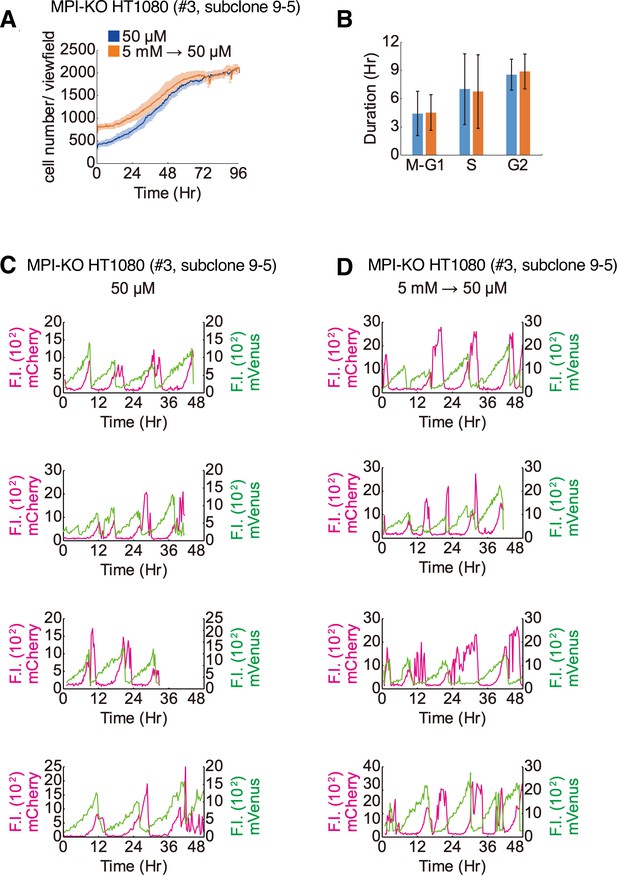
Time-lapse imaging of mannose phosphate isomerase knockout (MPI-KO) HT1080 cells.
(A) The number of MPI-KO HT1080 cells during the time-lapse imaging under mannose-unchallenged culture conditions (50 μM, blue). The same time-lapse imaging analysis was performed on cells that had been allowed to recover from mannose challenge (5 mM → 50 μM, orange) as in Figure 2G. (B) The durations of M–G1 (the sum of both phases), S, and G2 phases (n = 512 ± 176 cells) in MPI-KO HT1080 cells cultured in (A). (C, D) Representative Fucci(CA) signal profiles of MPI-KO HT1080 cells cultured in (A). Fluorescent intensity (F.I.) for mCherry (left y-axis) and mVenus (right y-axis) is shown in magenta and green, respectively.
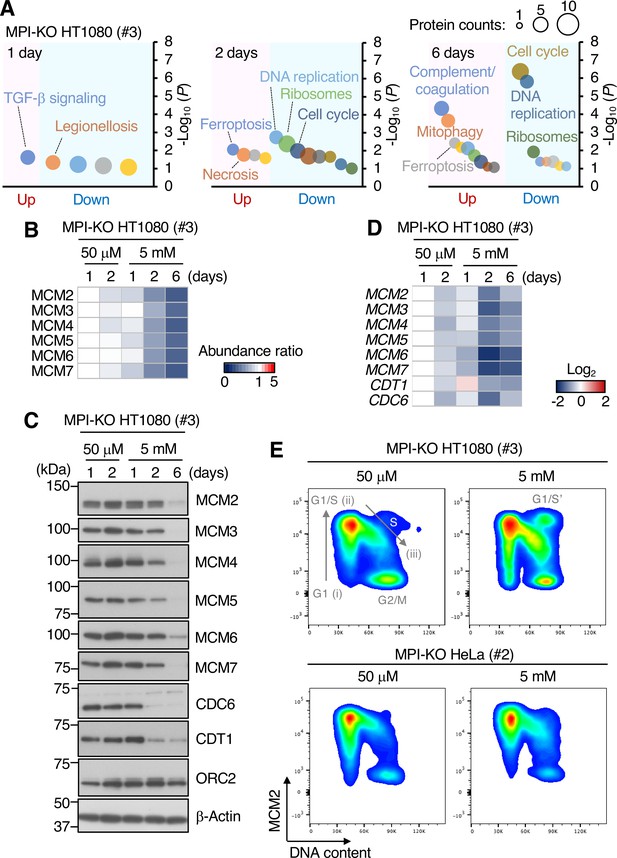
Mannose challenge limits DNA synthesis at ongoing replication forks.
(A) Functional annotation analysis of the proteomic data in mannose phosphate isomerase knockout (MPI-KO) HT1080 (#3) cells cultured in the presence of 50 μM or 5 mM mannose for the indicated time. (B) Heatmap representation of the relative amounts of MCM2-7 proteins in MPI-KO HT1080 (#3) cells cultured in the presence of 50 μM or 5 mM mannose for the indicated time. (C) Western blot analysis of whole-cell lysates of MPI-KO HT1080 (#3) cells cultured in the presence of 50 μM or 5 mM mannose for the indicated time. (D) Heatmap representation of the relative expression levels of MCM2, MCM3, MCM4, MCM5, MCM6, MCM7, CDT1, and CDC6 genes in MPI-KO HT1080 (#3) cells cultured in the presence of 50 μM or 5 mM mannose for the indicated time. (E) Chromatin flow cytometry for MCM2 and DNA content (Hoechst33342) in MPI-KO HT1080 (#3) or MPI-KO HeLa (#2) cells cultured in the presence of 50 μM or 5 mM mannose for 24 hr. The binding of MCM2 to chromatins (origin licensing) is detected in G1 phase (step i) and it peaks at G1/S boundary (step ii). As S phase progresses, MCM2 is dissociated from chromatins (step iii). G1/S’ denotes the G1/S boundary of cell populations that underwent endoreplication.
-
Figure 3—source data 1
Original blot images depicting cropped regions for Figure 3C.
- https://cdn.elifesciences.org/articles/83870/elife-83870-fig3-data1-v1.zip
-
Figure 3—source data 2
Original blot images depicting cropped regions for Figure 3C.
- https://cdn.elifesciences.org/articles/83870/elife-83870-fig3-data2-v1.zip
-
Figure 3—source data 3
Original blot images depicting cropped regions for Figure 3C.
- https://cdn.elifesciences.org/articles/83870/elife-83870-fig3-data3-v1.zip
-
Figure 3—source data 4
Original blot images depicting cropped regions for Figure 3C.
- https://cdn.elifesciences.org/articles/83870/elife-83870-fig3-data4-v1.zip
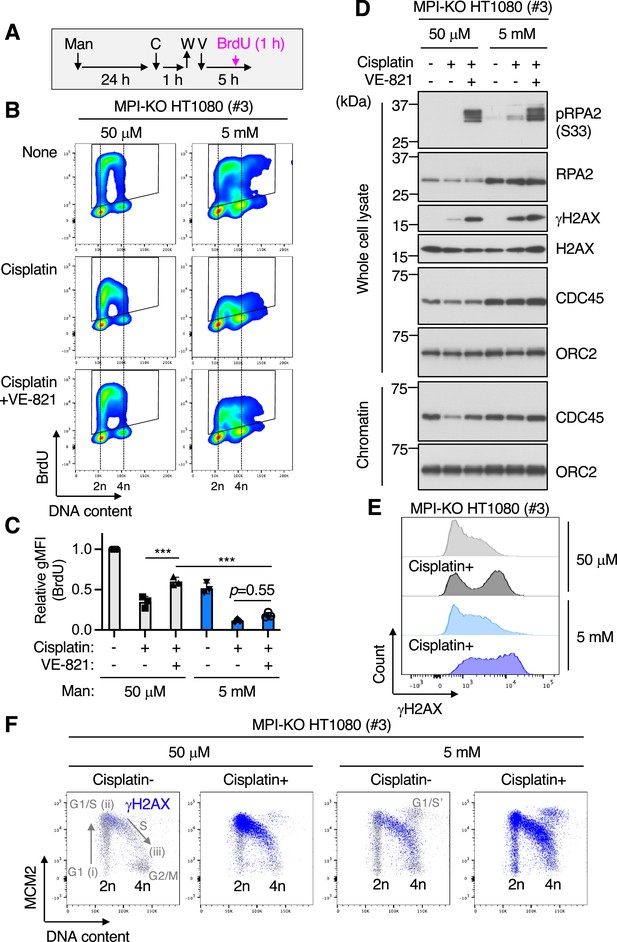
Mannose challenge disengages dormant origins from DNA synthesis during replication stress.
(A) Schematic representation of drug treatments. Man, 50 μM or 5 mM mannose; C, 100 μM cisplatin; W, wash; V, 1 μM VE-821; BrdU, 10 μM 5-bromo-2′-deoxyuridine. (B) Flow cytometry for BrdU and DNA content (Hoechst33342) in mannose phosphate isomerase knockout (MPI-KO) HT1080 (#3) cells treated as indicated in (A). The BrdU-positive cells were gated in black boxes for quantification of the geometric mean fluorescent intensity. (C) Relative geometric mean fluorescent intensity (gMFI) of BrdU in (B) (in the gated populations). The gMFI of BrdU in cells treated with 50 μM mannose in the absence of cisplatin (None) was set to 1.0. (D) Western blot analysis of whole-cell lysates and chromatin fractions of MPI-KO HT1080 (#3) cells treated as indicated in (A), except that BrdU labeling was omitted. (E, F) Chromatin flow cytometry for γH2AX, MCM2, and DNA content (Hoechst33342) in MPI-KO HT1080 (#3) cells treated as in (A), except that VE-821 treatment and BrdU labeling were omitted. The binding of MCM2 to chromatins (origin licensing) is detected in G1 phase (step i) and it peaks at G1/S boundary (step ii). As S phase progresses, MCM2 is dissociated from chromatins (step iii). G1/S’ denotes the G1/S boundary of cell populations that underwent endoreplication. Data represent the mean ± SD; n = 3 independent experiments. ***p<0.001, one-way ANOVA with post hoc Tukey’s test (C).
-
Figure 4—source data 1
Original blot images depicting cropped regions for Figure 4D.
- https://cdn.elifesciences.org/articles/83870/elife-83870-fig4-data1-v1.zip
-
Figure 4—source data 2
Original blot images depicting cropped regions for Figure 4D.
- https://cdn.elifesciences.org/articles/83870/elife-83870-fig4-data2-v1.zip
-
Figure 4—source data 3
Original blot images depicting cropped regions for Figure 4D.
- https://cdn.elifesciences.org/articles/83870/elife-83870-fig4-data3-v1.zip
-
Figure 4—source data 4
Original blot images depicting cropped regions for Figure 4D.
- https://cdn.elifesciences.org/articles/83870/elife-83870-fig4-data4-v1.zip
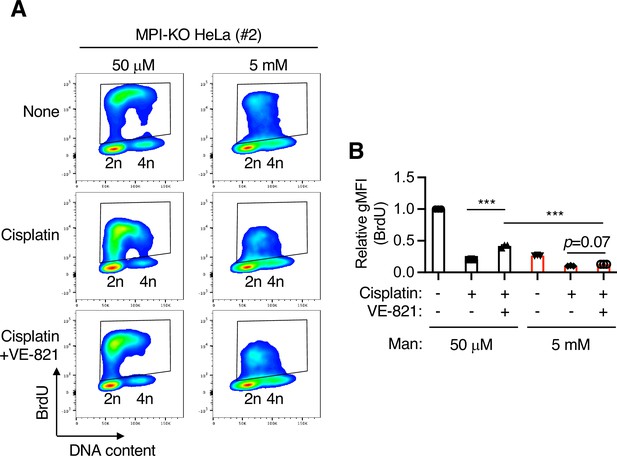
Mannose challenge disengages dormant origins from DNA synthesis during replication stress in mannose phosphate isomerase knockout (MPI-KO) HeLa cells.
(A) Flow cytometry for BrdU and DNA content in MPI-KO HeLa (#2) cells treated as in Figure 4A. The BrdU-positive cells were gated in black boxes for quantification of the geometric mean fluorescent intensity. (B) Relative geometric mean fluorescent intensity (gMFI) of BrdU in (A) (in the gated populations). The gMFI of BrdU in cells treated with 50 μM mannose in the absence of cisplatin (None) was set to 1.0. Data represent the mean ± SD; n = 3 independent experiments. ***p<0.001, one-way ANOVA with post hoc Tukey’s test (B).
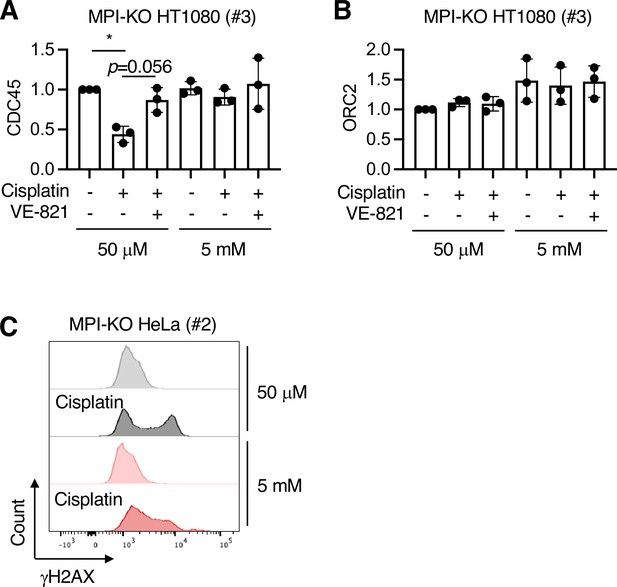
Mannose challenge impairs loading/unloading dynamics of CDC45 and enhances cisplatin-induced genomic instability.
(A, B) Relative amounts of the chromatin-bound CDC45 (A) and ORC2 (B) in mannose phosphate isomerase knockout (MPI-KO) HT1080 (#3) cells in Figure 4D. (C) Chromatin flow cytometry for γH2AX in MPI-KO HeLa (#2) cells treated as in Figure 4A, except that VE-821 treatment and BrdU labeling were omitted. Data represent the mean ± SD; n = 3 independent experiments. *p<0.05, one-way ANOVA with post hoc Tukey’s test (A, B).
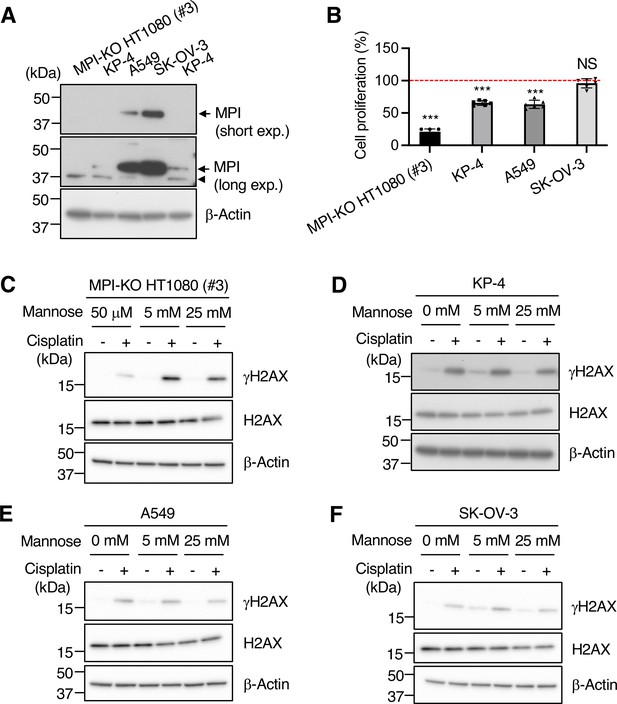
The effects of mannose on cell proliferation and genomic instability in human cancer cells with varying levels of mannose phosphate isomerase (MPI).
(A) Western blot analysis for MPI in MPI-KO HT1080 (#3), KP-4, A549, and SK-OV-3 cells (250 μg of wet cells). short exp., short exposure; long exp., long exposure. Arrows and arrowheads indicate MPI and bands cross-reacted with anti-MPI antibody, respectively. (B) Cell proliferation of the indicated cell lines after 24-h mannose treatment (5 mM for MPI-KO HT1080 [#3] and 25 mM for KP-4, A549, and SK-OV-3 cells). ATP levels in each cell line that was cultured in 50 μM (MPI-KO HT1080 cells) or 0 mM (KP-4, A549 and SK-OV-3 cells) mannose were set to 100% (red dashed line). (C–F) Western blot analysis forγH2AX, H2AX, and β-actin in MPI-KO HT1080 (#3) cells (C), KP-4 cells (D), A549 cells (E), and SK-OV-3 cells (F) that were treated as in Figure 4A, except that VE-821 and BrdU treatments were omitted. Data represent the mean ± SD; n = 6 independent experiments. ***p<0.001, NS, not significant, Welch’s t-test (B).
-
Figure 4—figure supplement 3—source data 1
Original blot images depicting cropped regions for Figure 4—figure supplement 3A.
- https://cdn.elifesciences.org/articles/83870/elife-83870-fig4-figsupp3-data1-v1.zip
-
Figure 4—figure supplement 3—source data 2
Original blot images depicting cropped regions for Figure 4—figure supplement 3C.
- https://cdn.elifesciences.org/articles/83870/elife-83870-fig4-figsupp3-data2-v1.zip
-
Figure 4—figure supplement 3—source data 3
Original blot images depicting cropped regions for Figure 4—figure supplement 3D.
- https://cdn.elifesciences.org/articles/83870/elife-83870-fig4-figsupp3-data3-v1.zip
-
Figure 4—figure supplement 3—source data 4
Original blot images depicting cropped regions for Figure 4—figure supplement 3E.
- https://cdn.elifesciences.org/articles/83870/elife-83870-fig4-figsupp3-data4-v1.zip
-
Figure 4—figure supplement 3—source data 5
Original blot images depicting cropped regions for Figure 4—figure supplement 3F.
- https://cdn.elifesciences.org/articles/83870/elife-83870-fig4-figsupp3-data5-v1.zip
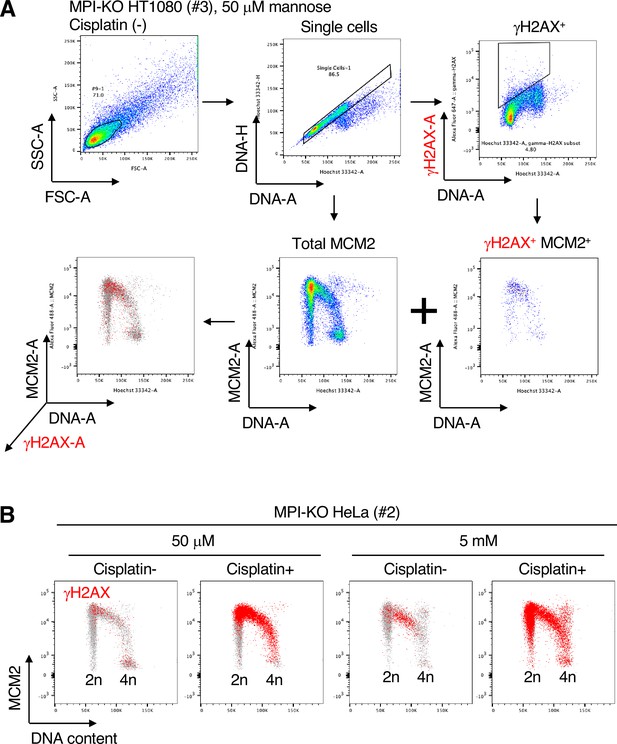
Identification of γH2AX-positive cells by chromatin flow cytometry at cell cycle levels.
(A) Strategy for gating the chromatin-bound γH2AX and MCM2 in Figure 4F. The chromatins were extracted from mannose phosphate isomerase knockout (MPI-KO) HT1080 (#3) cells and stained with anti-MCM2 and γH2AX antibodies and Hoechst33342 for DNA. Total single cells were used to plot total chromatin-bound MCM2 profile against DNA content (Total MCM), while γH2AX-positive populations (γH2AX+) were gated from single cells and used to plot the chromatin-bound MCM2 profile against DNA content (γH2AX+MCM2+). These two plots were overlaid to visualize γH2AX-positive cells in cell cycle progression. (B) Chromatin flow cytometry for γH2AX, MCM2 and DNA content (Hoechst33342) in MPI-KO HeLa (#2) cells treated as in Figure 4A, except that VE-821 treatment and BrdU labeling were omitted.
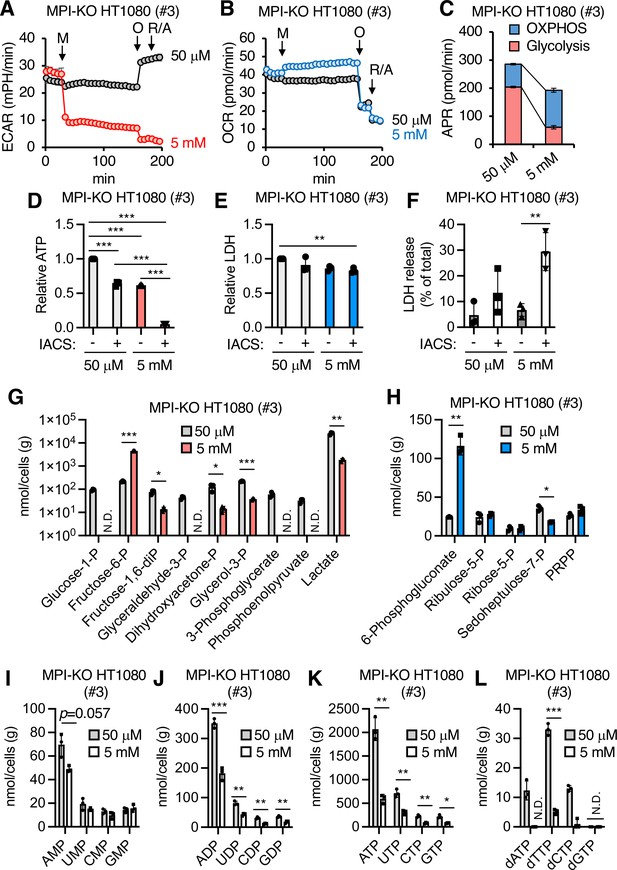
Mannose challenge impairs bioenergetic balance and generates a distinct metabolic landscape.
(A, B) Extracellular acidification rates (ECAR, A) and oxygen consumption rates (OCR, B) in mannose phosphate isomerase knockout (MPI-KO) HT1080 (#3) cells. The cells were first cultured in 50 μM mannose, and then the medium alone or mannose (5 mM final, M) was added to the cells. They were incubated for 120 min and further treated with 1.5 μM oligomycin A (O) for 18 min, followed by 0.5 μM each of rotenone and antimycin A (R/A) for 18 min. (C) ATP production rates (APR) estimated from the data in (A, B). See also the ‘Materials and methods.’ (D) Relative ATP levels in MPI-KO HT1080 (#3) cells treated for 6 hr with or without 30 nM IACS-010759 (IACS) in the presence of 50 μM or 5 mM mannose. (E) Relative lactate dehydrogenase (LDH) levels in MPI-KO HT1080 (#3) cells treated as in (D). (F) LDH release from MPI-KO HT1080 (#3) cells treated for 24 hr with or without 30 nM IACS-010759 (IACS) in the presence of 50 μM or 5 mM mannose. (G–L) Metabolomic profiling of glycolysis (G), the pentose phosphate pathway (H), ribonucleotides (I–K), and deoxyribonucleoside triphosphates (L) in MPI-KO HT1080 (#3) cells cultured for 24 hr in the presence of 50 μM or 5 mM mannose. P, phosphate; PRPP, 5-phosphoribosyl-1-pyrophosphate. Data represent the mean ± SD; n = 3 independent experiments. *p<0.05, **p<0.01, and ***p<0.001, one-way ANOVA with post hoc Tukey’s test (D–F) or Welch’s t-test (G–L).
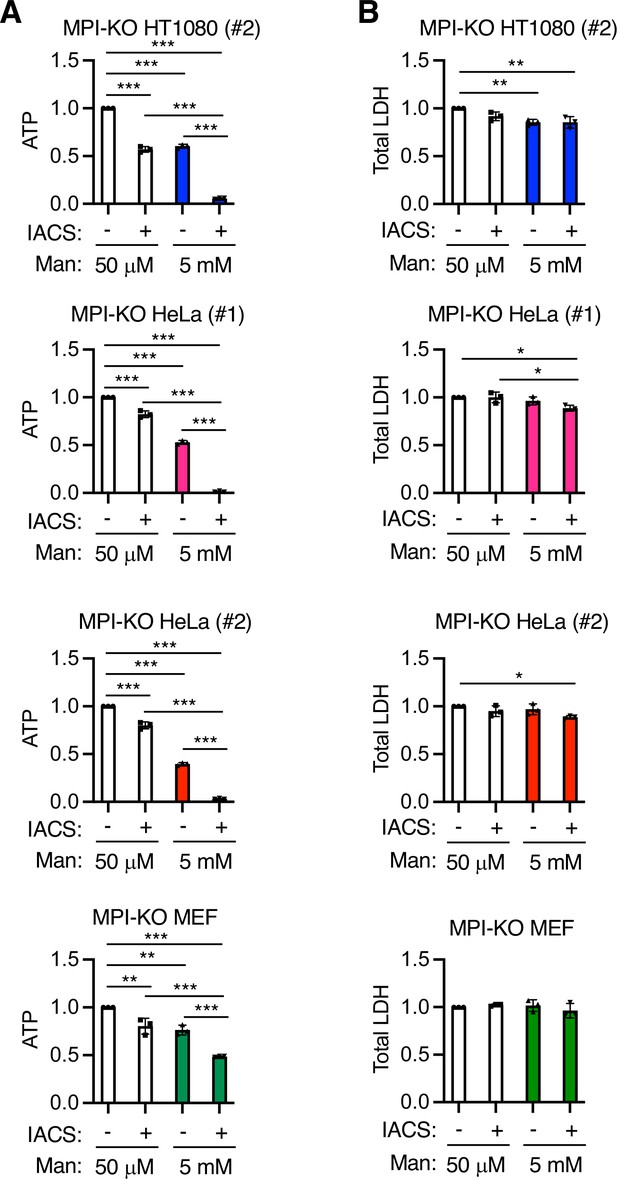
Mannose-challenged mannose phosphate isomerase knockout (MPI-KO) cells highly depend on oxidative phosphorylation (OXPHOS) for ATP production.
Relative ATP levels (A) and lactate dehydrogenase (LDH) activity (B) in MPI-KO HT1080 (#2) cells, MPI-KO HeLa (#1 and #2) cells, and MPI-KO MEFs co-treated with mannose (50 μM or 5 mM) and 30 nM IACS-010759 (IACS) or vehicle alone for 6 hr. Data represent the mean ± SD; n = 3 independent experiments. *p<0.05, **p<0.01, and ***p<0.001, one-way ANOVA with post hoc Tukey’s test.
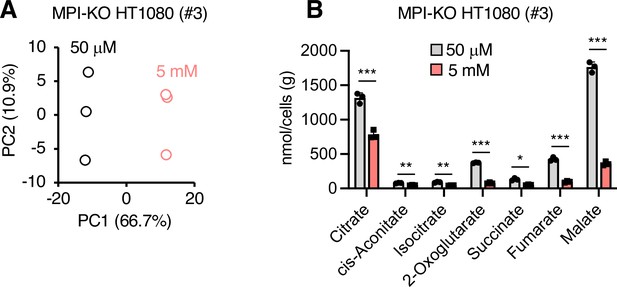
Metabolic changes in mannose-challenged and -unchallenged mannose phosphate isomerase knockout (MPI-KO) HT1080 cells.
(A) Principal component analysis of metabolites in MPI-KO HT1080 (#3) cells cultured in the presence of 50 μM or 5 mM mannose for 24 hr. (B) Quantification of metabolites in the tricarboxylic acid cycle. Data represent the mean ± SD; n = 3 independent experiments. *p<0.05, **p<0.01, ***p<0.001, NS, not significant, Welch’s t-test.
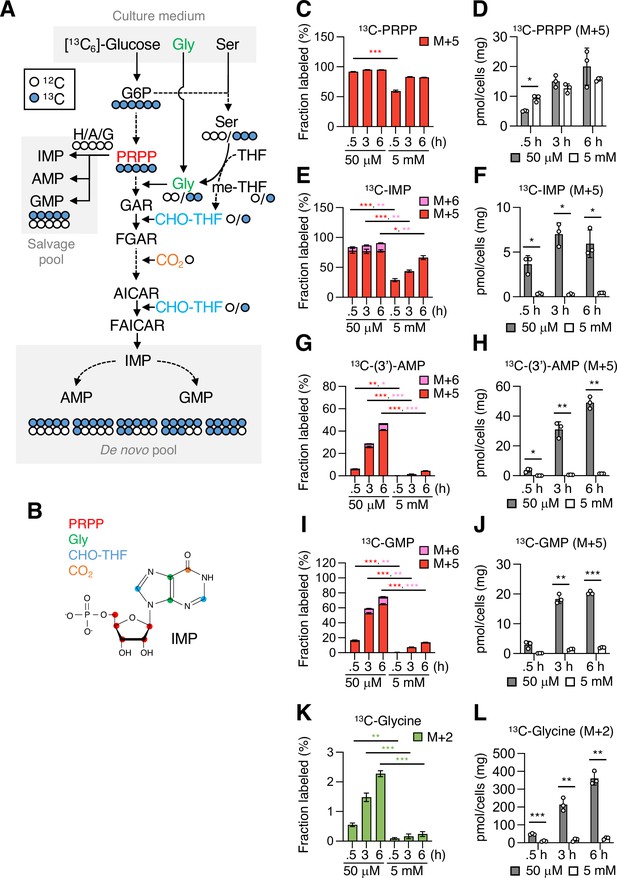
Mannose challenge severely impairs purine metabolism.
(A) A schematic representation of [13C6]-glucose tracing in purine metabolism. Blue and white circles denote 13C and 12C, respectively. Phosphoribosyl pyrophosphate (PRPP) is produced from glucose 6-phosphate (G6P) through glycolysis and the pentose phosphate pathway, while serine (Ser), glycine (Gly), 5,10-methylenetetrahydrofolate (me-THF), and 10-fomyltetrahydrofolate (CHO-THF) are produced through the glycolysis-serine biosynthesis-folate cycle axis. In salvage pathway of purine metabolism, PRPP is conjugated with hypoxanthine (X), adenine (A), or guanine (G) to form inosine 5’-monophosphate (IMP), adenosine 5’-monophosphate (AMP), or guanosine 5’-monophosphate (GMP), respectively. In the de novo pathway, IMP is formed from PRPP via multiple steps and used to produce AMP and GMP. Possible labeling patterns with 12C and 13C were indicated. GAR, 5'-phosphoribosylglycinamide; FGAR, 5'-phosphoribosyl-N-formylglycinamide; AICAR, 5'-phosphoribosyl-5-amino-4-imidazolecarboxamide; FAICAR, 5'-phosphoribosyl-5-formamido-4-imidazolecarboxamide. (B) A molecular structure of IMP. Red, green, blue and orange circles indicate carbon atoms originating from PRPP, Gly, CHO-THF and CO2, respectively. (C−L) Fractional enrichment (fraction labeled; C, E, G, I, K) and pool size (D, F, H, J, L) of the indicated metabolites. The mannose phosphate isomerase knockout (MPI-KO) HT1080 (#3) cells were preconditioned in mannose-challenged (5 mM) or -unchallenged (50 μM) culture medium for 24 hr and then labeled with [13C6]-glucose for the indicated periods before harvest. The amounts of AMP were estimated as a mixture with 3’-AMP [(3’)-AMP] due to their insufficient separation. Data represent the mean ± SD; n = 3 independent experiments. *p<0.05, **p<0.01, ***p<0.001, Welch’s t-test.
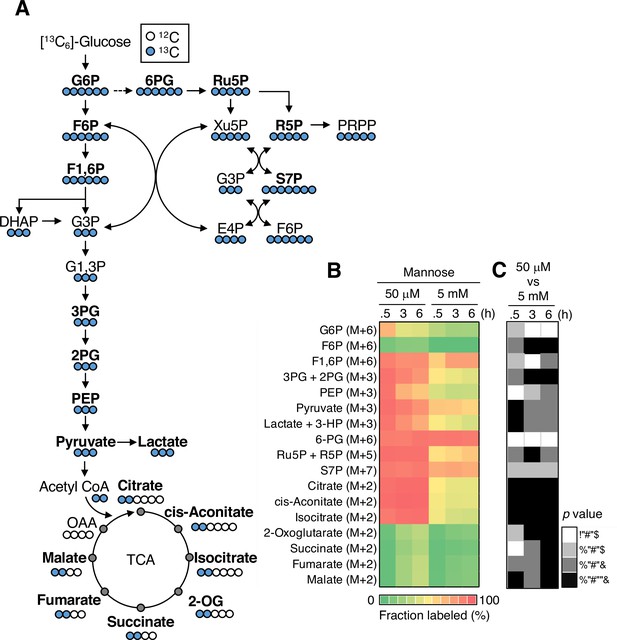
[13C6]-Glucose-tracing analysis for metabolites in glycolysis, the pentose phosphate pathway (PPP), and the tricarboxylic acid (TCA) cycle.
(A) A schematic representation of [13C6]-glucose tracing in glycolysis, the PPP, and the TCA cycle. Blue and white circles denote 13C and 12C, respectively. G6P, glucose-6-phosphate; F6P, fructose-6-phosphate; F1,6P, fructose-1,6-bisphosphate; DHAP, dihydroxyacetone; G3P, glyceraldehyde-3-phosphate; G1,3P, 1,3-bisphosphoglycerate; 3PG, 3-phospoglycerate; 2PG, 2-phosphoglycerate; PEP, phosphoenolpyruvate; 6PG, 6-phosphogluconate; Ru5P, ribulose-5-phosphate; R5P, ribose-5-phosphate; Xu5P, xylulose-5-phopshate; S7P, sedoheptulose-7-phosphate; E4P, erythrose-4-phosphate; 2-OG, 2-oxoglutarate; OAA, oxaloacetate. (B) Heatmap representation of fractional enrichment of the indicated metabolites. The mannose phosphate isomerase knockout (MPI-KO) HT1080 (#3) cells were preconditioned in mannose-challenged (5 mM) or -unchallenged (50 μM) culture medium for 24 hr and then labeled with [13C6]-glucose for the indicated periods before harvest. The fractional enrichment of 3PG and 2PG, lactate and 3-HP, and Ru5P and R5P were estimated as a mixture due to their insufficient separation. (C) Statistical analysis of data in (B). Data represent the mean; n = 3 independent experiments. p>0.05 (white squares), p<0.05 (light gray squares), p<0.01 (dark gray squares), p<0.001 (black squares), Welch’s t-test.
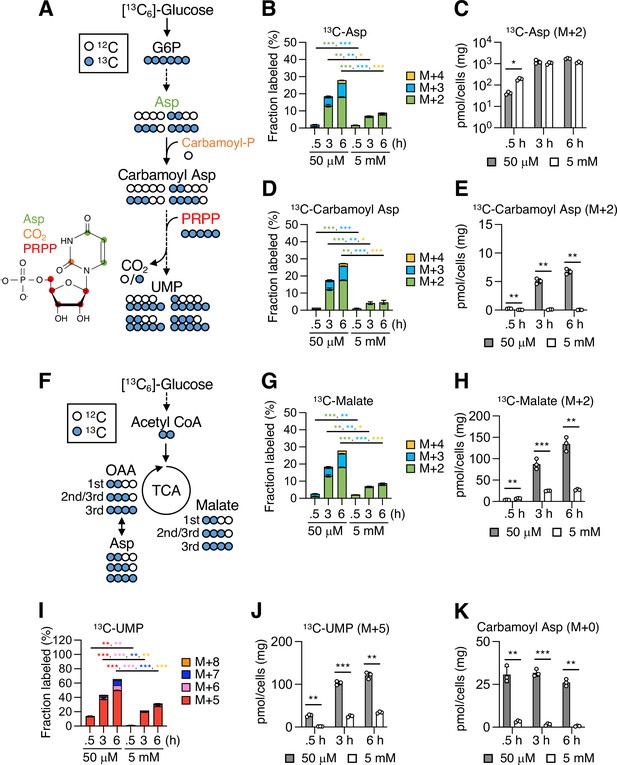
[13C6]-Glucose-tracing analysis for metabolites in pyrimidine metabolism.
(A) A schematic representation of [13C6]-glucose tracing in pyrimidine metabolism. Blue and white circles denote 13C and 12C, respectively. Aspartate (Asp) is produced from glucose 6-phosphate (G6P) through glycolysis and the tricarboxylic acid (TCA) cycle. In pyrimidine metabolism, Asp is conjugated with carbamoyl phosphate (carbamoyl-P) to form carbamoyl Asp, which is further conjugated with phosphoribosyl pyrophosphate (PRPP) to form ortidine-5’-monophosphate that is decarboxylated to give uridine-5’-monophosphate (UMP). The possible labeling patterns with 12C and 13C were indicated. A molecular structure of UMP is shown. Red, green, and orange circles indicate carbon atoms originating from PRPP, Asp, and CO2, respectively. (B–E) Fractional enrichment (fraction labeled; B, D) and pool size (C, E) of 13C-Asp and 13C-carbamoyl Asp. The mannose phosphate isomerase knockout (MPI-KO) HT1080 (#3) cells were preconditioned in mannose-challenged (5 mM) or -unchallenged (50 μM) culture medium for 24 hr and then labeled with [13C6]-glucose for the indicated periods before harvest. (F) A schematic representation of [13C6]-glucose tracing in the first, second, and third rounds of the TCA cycle. Blue and white circles denote 13C and 12C, respectively. The possible labeling patterns with 12C and 13C were indicated. OAA, oxaloacetate. (G, H) Fractional enrichment (fraction labeled; G) and pool size (H) of 13C-malate. (I, J) Fractional enrichment (fraction labeled; I) and pool size (J) of 13C-UMP. (K) Pool size of unlabeled carbamoyl Asp. Data represent the mean ± SD; n = 3 independent experiments. *p<0.05, **p<0.01, ***p<0.001, Welch’s t-test.
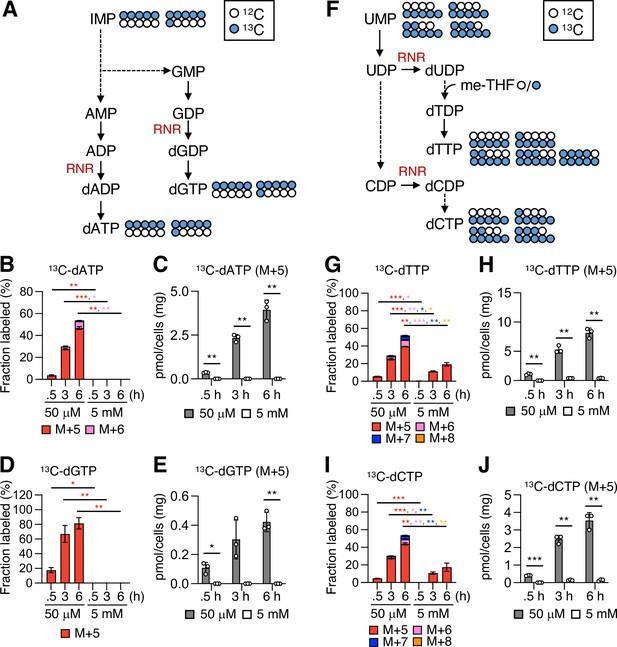
[13C6]-Glucose-tracing analysis for deoxyribonucleoside triphosphates (dNTPs).
(A) A schematic representation of [13C6]-glucose tracing in purine metabolism. Blue and white circles denote 13C and 12C, respectively. AMP, adenosine-5’-monophosphate; ADP, adenosine-5’-diphosphate; dADP, deoxyadenosine-5’-diphosphate; dATP, deoxyadenosine-5’-triphosphate; GMP, guanosine-5’-monophosphate; GDP, guanosine-5’-diphosphate; dGDP, deoxyguanosine-5’-diphosphate; dGTP, deoxyguanosine-5’-triphosphate; RNR, ribonucleotide reductase. The possible labeling patterns with 12C and 13C were indicated. (B−E) Fractional enrichment (fraction labeled; B, D) and pool size (C, E) of 13C-dATP and 13C-dGTP. The mannose phosphate isomerase knockout (MPI-KO) HT1080 (#3) cells were preconditioned in mannose-challenged (5 mM) or -unchallenged (50 μM) culture medium for 24 hr and then labeled with [13C6]-glucose for the indicated periods before harvest. (F) A schematic representation of [13C6]-glucose tracing in pyrimidine metabolism. Blue and white circles denote 13C and 12C, respectively. UMP, uridine-5’-monophosphate; UDP, uridine-5’-diphosphate; dUDP, deoxyuridine-5’-diphosphate; dTDP, deoxythymidine-5’-diphosphate; dTTP, deoxythymidine-5’-triphosphate; CDP, cytidine-5’-diphosphate; dCDP, deoxycytidine-5’-diphosphate; dCTP, deoxycytidine-5’-triphosphate; RNR, ribonucleotide reductase. The possible labeling patterns with 12C and 13C were indicated. (G−J) Fractional enrichment (fraction labeled; G, I) and pool size (H, J) of 13C-dTTP and 13C-dCTP. Data represent the mean ± SD; n = 3 independent experiments. *p<0.05, **p<0.01, ***p<0.001, Welch’s t-test.
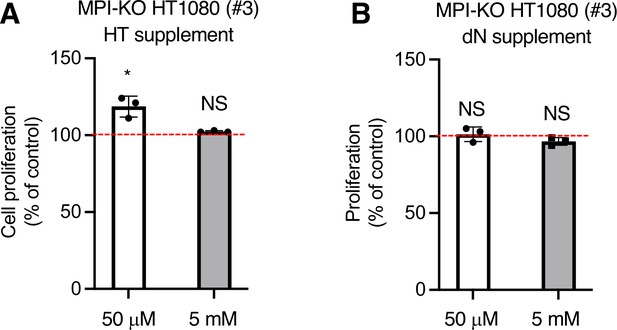
Supplementation of mannose-challenged mannose phosphate isomerase knockout (MPI-KO) HT1080 cells with hypoxanthine and thymidine or with deoxyribonucleoside mixture does not improve cell proliferation.
(A, B) Cell proliferation assay in MPI-KO HT1080 (#3) cells co-treated with mannose (50 μM or 5 mM) and a mixture of 100 μM hypoxanthine and 16 μM thymidine (HT supplement) or a mixture of 10 μM each of deoxyadenosine, thymidine, deoxycytidine, and deoxyguanosine (dN supplement). ATP levels in MPI-KO HT1080 (#3) cells that were cultured in 50 μM or 5 mM mannose without HT supplement and dN supplement were set to 100% (red dashed line). Data represent the mean ± SD; n = 3 independent experiments. *p< 0.05, NS, not significant, Welch’s t-test.
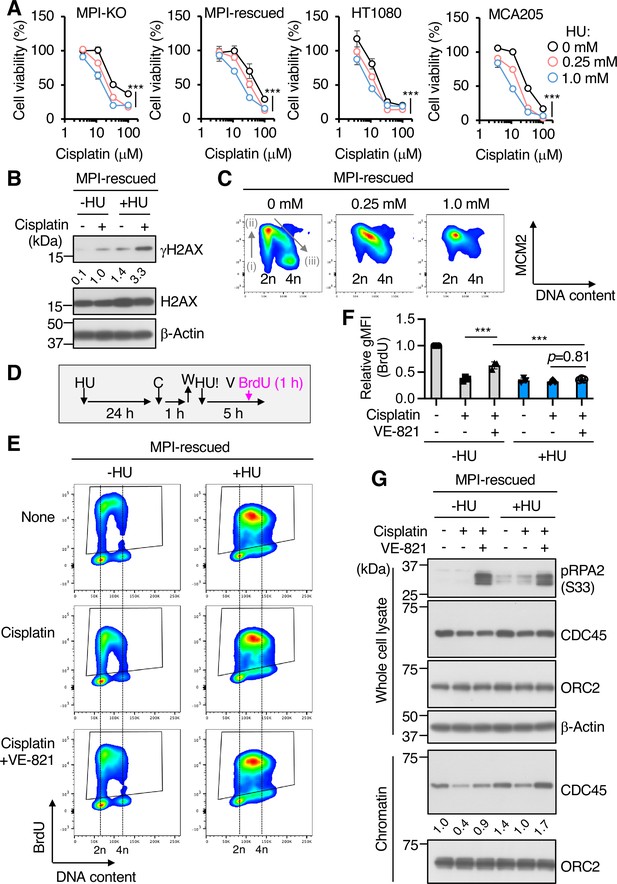
Pharmacological inhibition of de novo deoxyribonucleoside triphosphate (dNTP) biosynthesis retards cell cycle progression, increases chemosensitivity, and inhibits DNA synthesis from dormant origins.
(A) Cell viability assay in mannose phosphate isomerase knockout (MPI-KO) HT1080 (#3), the MPI-rescued cells (MPI-rescued), the parental HT1080, and mouse fibrosarcoma MCA205 cells that were preconditioned with hydroxyurea (HU) for 24 hr, followed by incubation with cisplatin for an additional 24 hr in the presence of the same concentrations of HU used for preconditioning. (B) Western blot analysis of whole-cell lysates from the MPI-rescued MPI-KO HT1080 (#3) cells preconditioned with 0.25 mM HU for 24 hr and pulsed with 100 μM cisplatin for 1 hr, followed by a 5 hr chase. Numbers indicate the relative amounts of γH2AX (the average of two independent experiments each from the MPI-rescued MPI-KO HT1080 cells and the parental HT1080 cells). (C) Chromatin flow cytometry for MCM2 and DNA content (Hoechst33342) in the MPI-rescued MPI-KO HT1080 (#3) cells treated with or without HU for 24 hr at the indicated concentrations. The binding of MCM2 to chromatin is detected in G1 phase (step i) and it peaks at G1/S boundary (step ii). As S phase progresses, MCM2 is dissociated from chromatin (step iii). (D) Schematic representation of drug treatment. HU, 0.25 mM hydroxyurea; C, 100 μM cisplatin; W, wash; V, 1 μM VE-821; 10 μM BrdU, 5-bromo-2′-deoxyuridine. (E) Flow cytometry for BrdU and DNA content (Hoechst33342) in the MPI-rescued MPI-KO HT1080 (#3) cells that were treated as indicated in (D). The BrdU-positive cells were gated in black boxes for quantification of the geometric mean fluorescent intensity. (F) Relative geometric mean fluorescent intensity (gMFI) of BrdU in (E) (in the gated populations). The gMFI of BrdU in cells treated without HU (None) was set to 1.0. (G) Western blot analysis of whole-cell lysates and chromatin fractions of the MPI-rescued MPI-KO HT1080 (#3) cells treated as indicated in (D), except that BrdU labeling was omitted. Numbers indicate the relative amounts of chromatin-bound CDC45 (the average of three independent experiments). Data represent the mean ± SD; n = 3 independent experiments. *p<0.05, **p<0.01, and ***p<0.001, two-way ANOVA with post hoc Bonferroni’s test (A) or one-way ANOVA (F) with post hoc Tukey’s test.
-
Figure 7—source data 1
Original blot images depicting cropped regions for Figure 7B.
- https://cdn.elifesciences.org/articles/83870/elife-83870-fig7-data1-v1.zip
-
Figure 7—source data 2
Original blot images depicting cropped regions for Figure 7G.
- https://cdn.elifesciences.org/articles/83870/elife-83870-fig7-data2-v1.zip
-
Figure 7—source data 3
Original blot images depicting cropped regions for Figure 7G.
- https://cdn.elifesciences.org/articles/83870/elife-83870-fig7-data3-v1.zip
-
Figure 7—source data 4
Original blot images depicting cropped regions for Figure 7G.
- https://cdn.elifesciences.org/articles/83870/elife-83870-fig7-data4-v1.zip
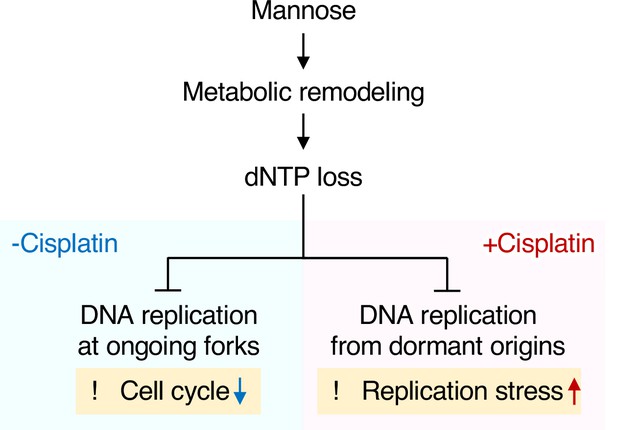
Proposed model for the mechanism underlying anticancer activity of mannose in mannose phosphate isomerase knockout (MPI-KO) human cancer cells.
A large influx of mannose into MPI-KO human cancer cells induces metabolic remodeling, leading to the loss of deoxyribonucleoside triphosphates (dNTPs). This metabolic deficiency impairs DNA replication at ongoing replication forks and slows cell cycle progression in the absence of cisplatin (-Cisplatin). On the other hand, mannose-caused dNTP loss disengages dormant origins from DNA replication in the presence of cisplatin, thereby exacerbating replication stress (+Cisplatin).
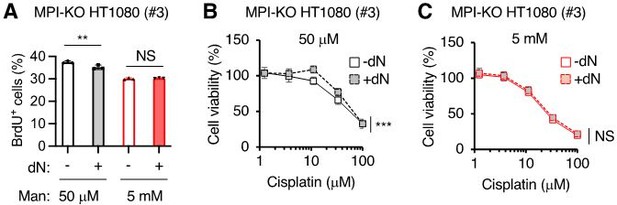
The effects of deoxyribonucleoside supplementation on cell proliferation, DNA synthesis and chemosensitivity in MPI-KO HT1080 cells.
(A) The percentage of MPI-KO HT1080 (#3) cells that were actively incorporating 2-bromo-5-deoxyuridine (BrdU+). Before 1-h pulse labelling with BrdU, the cells were cultured for 24 h in culture medium supplemented with (+) or without (-) a mixture of dNs in the presence of mannose (Man) at the indicated concentrations. (B and C) Cell viability in the MPI-KO HT1080 (#3) cells that were precondition for 24 h with (+) or without (-) dN mixture in the presence of mannose at 50 µM (B) or 5 mM (C), followed by the incubation with cisplatin for additional 24 h under the same culture conditions. Data represent the mean ± SD, n=3 independent experiments. **p<0.01, and ***p<0.001, NS, not significant, Welch’s t-test (A), two-way ANOVA with post hoc Bonferroni’s (B and C).
Videos
Time-lapse imaging of cell cycles in mannose phosphate isomerase knockout (MPI-KO) HT1080 cells.
MPI-KO HT1080 cells that expressed Fucci(CA) reporters were observed for 76 hr under the mannose-unchallenged (left) or the mannose-challenged (right) culture conditions. Red, mCherry-hCdt1(1/100)Cy(-); green, mVenus-hGem(1/110). Images were acquired every 15 min. Image size: 253.44 μm × 190.48 μm for each panel.
Abnormal cytokinesis during M phase in the mannose-challenged mannose phosphate isomerase knockout (MPI-KO) HT1080 cells with normal-like Fucci(CA) signal profiles.
Time-lapse movies of three representative Fucci(CA)-expressing MPI-KO HT1080 cells that were cultured under the mannose challenge conditions. Red, mCherry-hCdt1(1/100)Cy(-); green, mVenus-hGem(1/110). Note that the cells in G2-M phase (yellow) show abnormal cytokinesis. Images were acquired every 15 min. Image size: 52.8 μm × 52.8 μm for each panel.
Tables
Reagent type (species) or resource | Designation | Source or reference | Identifiers | Additional information |
---|---|---|---|---|
Gene (Homo sapiens) | MPI | GenBank | Gene ID: 4351 | |
Genetic reagent (H. sapiens) | MPI-KO HT1080 | This paper | Clone #2, #3, which can be obtained from the Department of Glyco-Oncology and Medical Biochemistry, Osaka International Cancer Institute | |
Genetic reagent (H. sapiens) | Fucci MPI-KO HT1080 | This paper | Clone #3 subclone (9-5), which can be obtained from the Department of Glyco-Oncology and Medical Biochemistry, Osaka International Cancer Institute | |
Genetic reagent (H. sapiens) | MPI-KO HeLa | This paper | Clone #1, #2, which can be obtained from the Department of Glyco-Oncology and Medical Biochemistry, Osaka International Cancer Institute | |
Genetic reagent (Mus musculus) | Wild-type mouse embryonic fibroblasts | DeRossi et al., 2006 | ||
Genetic reagent (M. musculus) | MPI-KO mouse embryonic fibroblasts | DeRossi et al., 2006 | ||
Cell line (H. sapiens) | HT1080 | Our laboratory stock | Validated by short tandem repeat profiling | |
Cell line (H. sapiens) | HeLa | RIKEN BioResource Research Center | RCB0007 | |
Cell line (H. sapiens) | KP-4 | RIKEN BioResource Research Center | RCB1005 | |
Cell line (H. sapiens) | SK-OV-3 | American Type Culture Collection (ATCC) | HTB-77 | |
Cell line (H. sapiens) | A549 | ATCC | CRM-CCL-185 | |
Cell line (M. musculus) | MCA205 | A gift from Rosenberg lab | ||
Cell line (H. sapiens) | The Plat-A retroviral packaging cell line | Cell Biolabs | RV-102 | |
Antibody | Anti-MPI (rabbit polyclonal) | GeneTex | GTX103682 | WB (1:5000) |
Antibody | Anti-β-actin (mouse monoclonal) | FUJIFILM Wako | 010-27841 | WB (1:10,000) |
Antibody | Anti-H2AX (mouse monoclonal) | Santa Cruz | 938CT5.1.1 | WB (1:2000) |
Antibody | Anti-phospho-H2AX (Ser139) (mouse monoclonal) | Millipore | JBW301 | WB (1:5000) Chromatin FACS (1:200) |
Antibody | Anti-RPA2 (mouse monoclonal) | Santa Cruz | 9H8 | WB (1:5000) |
Antibody | Anti-Phospho-RPA2 (S33) (rabbit polyclonal) | Bethyl | A300-246A | WB (1:10,000) |
Antibody | Anti-MCM2 (rabbit monoclonal) | Cell Signaling Technology | D7G11 | WB (1:10,000) Chromatin FACS (1:200) |
Antibody | Anti-MCM3 (mouse monoclonal) | Santa Cruz | E-8 | WB (1:10,000) |
Antibody | Anti-MCM4 (rabbit polyclonal) | GeneTex | GTX109740 | WB (1:5000) |
Antibody | Anti-MCM5 (rabbit polyclonal) | GeneTex | GTX33310 | WB (1:5000) |
Antibody | Anti-MCM6 (mousemonoclonal) | Santa Cruz | H-8 | WB (1:20,000) |
Antibody | Anti-MCM7 (mouse monoclonal) | Santa Cruz | 141.2 | WB (1:5000) |
Antibody | Anti-CDT1 (rabbit monoclonal) | Abcam | ab202067 | WB (1:10,000) |
Antibody | Anti-CDC6 (rabbit monoclonal) | Abcam | ab109315 | WB (1:1000) |
Antibody | Anti-ORC2 (rat monoclonal) | Santa Cruz | 3G6 | WB (1:10,000) |
Antibody | Anti-CDC45 (rabbit monoclonal) | Cell Signaling Technology | D7G6 | WB (1:10,000) |
Antibody | Alexa Fluor 488-conjugated anti-BrdU antibody (mouse monoclonal) | BioLegend | 3D4 | FACS (1:100) |
Antibody | Anti-rabbit IgG-Alexa Fluor 488 (donkey polyclonal) | Thermo Fisher Scientific | R37118 | Chromatin FACS (1:1000) |
Antibody | Anti-mouse IgG-Alexa Fluor 647 (donkey polyclonal) | Thermo Fisher Scientific | A-31571 | Chromatin FACS (1:1000) |
Recombinant DNA reagent | pENTR-hMPI | This paper | This reagent can be obtained from the Department of Glyco-Oncology and Medical Biochemistry, Osaka International Cancer Institute | |
Recombinant DNA reagent | pMXs-Neo-hMPI | This paper | This reagent can be obtained from the Department of Cancer Drug Discovery and Development, Osaka International Cancer Institute | |
Recombinant DNA reagent | mCherry-hCdt1(1/100)Cy(−)/pcDNA3 | RIKEN BioResource Research Center | RDB15459 | |
Recombinant DNA reagent | pMXs-Neo to yield pMXs-Neo-mCherry-hCdt1(1/100)Cy(−) | This paper | This reagent can be obtained from the Department of Cancer Drug Discovery and Development, Osaka International Cancer Institute | |
Recombinant DNA reagent | pMMLV-mVenus-hGem(1/110):IRES:Bsd | Vector Builder | ||
Recombinant DNA reagent | Edit-R SMART Cas9_mCMV_(PuroR) expression plasmid | Dharmacon | U-005200-120 | |
Recombinant DNA reagent | pENTR/D-TOPO vector | Thermo Fisher Scientific | K240020 | |
Recombinant DNA reagent | pMXs-Neo retroviral expression vector | Cell Biolabs | RTV-011 | |
Sequence-based reagent | Synthetic CRISPR RNAs for human MPI | Dharmacon | CM-011729-01 CM-011729-03 | |
Sequence-based reagent | trans-activating CRISPR RNA | Dharmacon | U-002005-05 | |
Commercial assay or kit | VECTASTAIN ABC-HRP kit | Vector Laboratories | PK-4000 | |
Commercial assay or kit | CellTiter-Glo 2.0 | Promega | G924B | |
Commercial assay or kit | Cytotoxicity LDH Assay Kit-WST | Dojindo | CK12 | |
Commercial assay or kit | PAGE Clean Up Kit | Nacalai | 06441-50 | |
Commercial assay or kit | In-Fusion HD Cloning Kit | Clontech | 639648 | |
Commercial assay or kit | RNeasy Mini Kit | QIAGEN | 74106 | |
Commercial assay or kit | NucleoSpin Gel and PCR Clean-Up Kit | Clontech | 740609 | |
Commercial assay or kit | THUNDERBIRD Next SYBR qPCR Mix | TOYOBO | QPX-201 | |
Chemical compound, drug | Cellstain Hoechst 33342 solution | Dojindo | H342 | FACS (1:100) Chromatin FACS (1:200) |
Chemical compound, drug | 5-Bromo-2’-deoxyuridine | Tokyo Chemical Industry | B1575 | |
Chemical compound, drug | 2’-Deoxyadenosine | Wako | 046-18693 | |
Chemical compound, drug | Thymidine | Wako | 203-19423 | |
Chemical compound, drug | 2’-Deoxyguanosine | Tokyo Chemical Industry | D0052 | |
Chemical compound, drug | 2’-Deoxycytidine | Tokyo Chemical Industry | D3583 | |
Chemical compound, drug | Dialyzed FBS | Cytiva | SH30079.02 | |
Chemical compound, drug | Cisplatin | Tokyo Chemical Industry | D3371 | |
Chemical compound, drug | Doxorubicin | FUJIFILM Wako | 040-21521 | |
Chemical compound, drug | IACS-010759 | Selleckchem | S8731 | |
Chemical compound, drug | VE-821 | Sigma-Aldrich | SML1415 | |
Chemical compound, drug | Hydroxyurea | FUJIFILM Wako | 085-06653 | |
Chemical compound, drug | [13C6]-Glucose | Cambridge Isotope Laboratories | CLM-1396-1 | |
Chemical compound, drug | cOmplete Protease Inhibitor Cocktail | Sigma-Aldrich | 11836170001 | |
Chemical compound, drug | PhosSTOP | Sigma-Aldrich | 4906845001 | |
Chemical compound, drug | Biotinylated concanavalin A | Cosmo Bio | J203 | Lectin blot (1:10,000) |
Chemical compound, drug | TRIzol Reagent | Thermo Fisher Scientific | 15596026 | |
Chemical compound, drug | Lipofectamine 3000 | Thermo Fisher Scientific | L3000015 | |
Chemical compound, drug | Lipofectamine 2000 | Thermo Fisher Scientific | 11668027 | |
Software, algorithm | MASCOT 2.8 | Matrix Science | ||
Software, algorithm | Proteome Discoverer 2.4 | Thermo Fisher Scientific | ||
Software, algorithm | ImageJ | Schneider et al., 2012 | ||
Software, algorithm | Trackmate | Tinevez et al., 2017 | ||
Software, algorithm | MATLAB | MathWorks |
Additional files
-
Supplementary file 1
List of primers used in this study.
- https://cdn.elifesciences.org/articles/83870/elife-83870-supp1-v1.docx
-
Supplementary file 2
List of plasmids used in this study.
- https://cdn.elifesciences.org/articles/83870/elife-83870-supp2-v1.docx
-
MDAR checklist
- https://cdn.elifesciences.org/articles/83870/elife-83870-mdarchecklist1-v1.docx
-
Source data 1
Proteomic data.
- https://cdn.elifesciences.org/articles/83870/elife-83870-data1-v1.xlsx
-
Source data 2
Functional annotation analysis of proteomic data.
- https://cdn.elifesciences.org/articles/83870/elife-83870-data2-v1.xlsx
-
Source data 3
Metabolomic data.
- https://cdn.elifesciences.org/articles/83870/elife-83870-data3-v1.xlsx
-
Source data 4
Metabolomic data for stable isotope labeling experiments.
- https://cdn.elifesciences.org/articles/83870/elife-83870-data4-v1.xlsx