Hippo signaling impairs alveolar epithelial regeneration in pulmonary fibrosis
Abstract
Idiopathic pulmonary fibrosis (IPF) consists of fibrotic alveolar remodeling and progressive loss of pulmonary function. Genetic and experimental evidence indicates that chronic alveolar injury and failure to properly repair the respiratory epithelium are intrinsic to IPF pathogenesis. Loss of alveolar type 2 (AT2) stem cells or mutations that either impair their self-renewal and/or impair their differentiation into AT1 cells can serve as a trigger of pulmonary fibrosis. Recent reports indicate increased YAP activity in respiratory epithelial cells in IPF lungs. Individual IPF epithelial cells with aberrant YAP activation in bronchiolized regions frequently co-express AT1, AT2, conducting airway selective markers and even mesenchymal or EMT markers, demonstrating ‘indeterminate’ states of differentiation and suggesting that aberrant YAP signaling might promote pulmonary fibrosis. Yet, Yap and Taz have recently also been shown to be important for AT1 cell maintenance and alveolar epithelial regeneration after Streptococcus pneumoniae-induced injury. To investigate how epithelial Yap/Taz might promote pulmonary fibrosis or drive alveolar epithelial regeneration, we inactivated the Hippo pathway in AT2 stem cells resulting in increased nuclear Yap/Taz, and found that this promotes their alveolar regenerative capacity and reduces pulmonary fibrosis following bleomycin injury by pushing them along the AT1 cell lineage. Vice versa, inactivation of both Yap1 and Wwtr1 (encoding Taz) or Wwtr1 alone in AT2 cell stem cells impaired alveolar epithelial regeneration and resulted in increased pulmonary fibrosis upon bleomycin injury. Interestingly, the inactivation of only Yap1 in AT2 stem cells promoted alveolar epithelial regeneration and reduced pulmonary fibrosis. Together, these data suggest that epithelial Yap promotes, and epithelial Taz reduces pulmonary fibrosis suggesting that targeting Yap but not Taz-mediated transcription might help promote AT1 cell regeneration and treat pulmonary fibrosis.
Editor's evaluation
This is an interesting and potentially significant study that adds important new information to our understanding of the mechanisms of lung epithelial repair after tissue injury. The authors have delineated a novel and non-redundant role for the hippo pathway and the downstream regulators Yap/Taz in regulating the repair of lung injury. These studies will inform future investigations into the mechanisms of the repair of lung injury.
https://doi.org/10.7554/eLife.85092.sa0Introduction
Idiopathic pulmonary fibrosis (IPF) pathogenesis encompasses alveolar and fibrotic remodeling, inflammation, and eventual loss of lung architecture Barkauskas and Noble, 2014 resulting in progressive loss of pulmonary function, respiratory failure, and death, often within 5 years of diagnosis King et al., 2011; Steele and Schwartz, 2013. It is now largely accepted that key mechanisms of IPF initiation and progression include chronic alveolar injury and failure to properly repair the respiratory epithelium by AT2 cells Camelo et al., 2014; Thannickal et al., 2004; Yang et al., 2013. Histologically, IPF is typified by respiratory epithelial cells in the lung parenchyma that express atypical proximal airway epithelial cell type markers including those expressed by goblet cells and BCs that are normally restricted to conducting airways and indeterminate cell type markers Plantier et al., 2011; Seibold et al., 2013. These atypical epithelial cells form bronchiolized or honeycomb structures that replace normal alveolar structures. Dramatic changes in ciliated, basal, and goblet-cell gene signatures correspond with the loss of genes associated with normal alveolar epithelial cells, reflecting profound changes in epithelial cells in both the airways and alveoli in IPF lungs Seibold et al., 2013; Kropski et al., 2015; Xu et al., 2016.
Yap/Taz signaling are important in alveolar epithelial regeneration by AT2 cells after Streptococcus pneumoniae-induced injury LaCanna et al., 2019. In this model, simultaneous inactivation of Yap1 and Wwtr1 (Taz) resulted in impaired alveolar epithelial regeneration, whereas single Yap1 or Wwtr1 mutant mice had minor or no phenotypes LaCanna et al., 2019. Interestingly, loss of Yap1/Wwtr1 in AT1 cells leads to spontaneous reprogramming into the AT2 cell lineage. However, while overexpression of a dominant active Yap1S112A in AT2 cells induced Hopx expression, these cells did not exhibit the classic flattened and elongated morphology of mature AT1 cells, and most continued to express Sftpc Penkala et al., 2021. Yap1S112A overexpression often does not recapitulate the effects of Hippo pathway inactivation as Yap1S112A induces a feedback activation of the Hippo pathway, resulting in increased degradation of endogenous Yap and Taz Chen et al., 2015; Moroishi et al., 2015. In addition, the inactivation of Yap1 alone in AT1 cells has no effect on AT1 cell marker expression. These data suggest that nuclear Yap can drive the expression of some AT1 cell genes but may not be sufficient to fully reprogram AT2 cells into AT1 cells. However, the inactivation of Hippo kinases Stk3/4 (Mst2/1, respectively), resulting in increased nuclear Yap and Taz, promotes the proliferation of AT2s and their differentiation into AT1s Gokey et al., 2021; Little et al., 2021. Interestingly, the inactivation of Wwtr1 in adult mouse AT2 cells has been shown to result in reduced AT1 cell differentiation in organoids and bleomycin-induced lung injury Sun et al., 2019. Together these findings again suggest an important role for Taz in AT1 cell differentiation. Yet, in a pneumonectomy model inactivation of Yap1 in AT2 cells (SftpcCreER; Yap1f/-) decreased their proliferation and differentiation into AT1 cells compared to AT2 cells heterozygous for Yap1 (SftpcCreER; Yap1f/+) Liu et al., 2016, though comparison to wildtype (SftpcCreER; Yap1+/+) AT2 cells was not performed.
Clearly, the Hippo pathway plays a very important role in lung development, homeostasis, and repair after injury. Although most of the available literature investigating the role of the Hippo pathway in the lung considers Yap and Taz as functionally redundant, some of the conflicting findings described above can only be explained by the fact that Yap and Taz play distinctive or even contrasting roles in different contexts and have dose-dependent as well as nuclear and cytoplasmic roles. Yap and Taz have common and distinctive structural features, reflecting only partially overlapping regulatory mechanisms and control nonidentical transcriptional programs. The issue of their divergent roles is currently underexplored in lung biology but holds fundamental implications for mechanistic and translational studies.
Many labs have found that AT2 to AT1 cell differentiation is impaired in IPF and that cells get stuck in a transitional state Jiang et al., 2020; Kobayashi et al., 2020. After lung injury, damage-associated transient progenitors (DATPs) emerge, representing a transitional state between AT2 stem cells and newly regenerated AT1 cells (aka pre-alveolar type-1 transitional cell state (PATS)) Kobayashi et al., 2020; Choi et al., 2020. Interestingly, DATP or PATS express profibrotic genes and show an enrichment of TP53, YAP, TGFβ, DNA-damage-response signaling, and cellular senescence, all of which are known to be involved in fibrosis in multiple organs, including the lung. However, the molecular pathways that induce and/or maintain DATPs are not completely understood nor is it clear whether promoting AT1 cell differentiation is sufficient to drive the resolution of pulmonary fibrosis. Here, by using murine lineage tracing coupled with injury-repair models and spatial transcriptomics studies, we uncovered a method to treat lung fibrosis by promoting AT1 cell differentiation through inhibition of Yap1. Mechanistically, the use of loss of function and spatial transcriptomics revealed an inhibition of Wwtr1 and AT1 cell differentiation by Yap. Importantly, these transitional states correlate with abnormal epithelial cells associated with defective fibrotic foci in human lungs with progressive pulmonary fibrosis.
Results
AT2 cells are actively maintained by Hippo signaling
We have previously shown that in the developing lung heterozygous inactivation of epithelial Yap1 results in a striking lung phenotype Volckaert et al., 2019. We found that, in the lung epithelium, Yap suppresses Wwtr1 (Taz) expression and that cytoplasmic Yap inhibits β-catenin signaling. We further showed that nuclear Taz is specifically expressed in AT1 cells and that epithelial inactivation of Wwtr1, but not Yap1, impaired AT1 cell differentiation Volckaert et al., 2019.
To better evaluate the expression of Hippo pathway mediators in the lung we performed single nuclear RNAseq on E18.5 ctrl lungs. We chose this time point as most lung cells have been differentiated at this time and the lung is still small enough that isolating nuclei at this stage likely would allow us to sequence all major cell types. Interestingly, we find that Wwtr1 is expressed the highest in AT1 cells which also express Tead1 and Tead4. However, while Tead1 is expressed also at lower levels in other lung epithelial cells the expression of Tead4 was specific to AT1 cells. Together these data further support an important role for Taz-Tead4 mediated transcription in AT1 cell specification and maintenance (Figure 1).
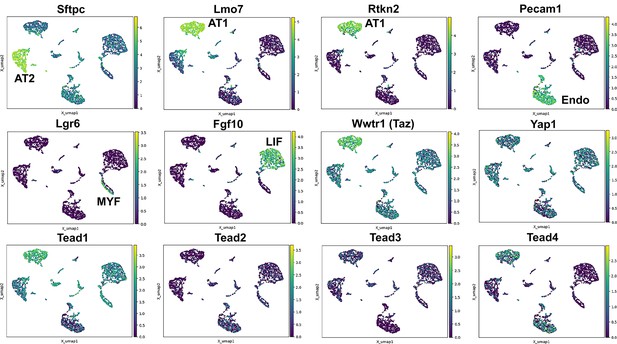
Hippo pathway expression in the lung.
Single nuclei RNA-seq was performed on E18.5 lungs. (Top and middle row) Cell types were identified based on gene expression: Sftpc (AT2); Lmo7, Rtkn2 (AT1); Pecam1 (endothelial); Lgr6 (myofibroblast/airway smooth muscle); Fgf10 (lipofibroblast). (MIddle and bottom row) Tead 1–4, Wwtr1 (Taz), and Yap1 mapped to these cell types.
Because many developmental pathways (Fgf, Shh, Wnt, etc) are also important in maintaining homeostasis and become activated in the response to injury in the adult lung, we sought to determine how the Hippo pathway maintains homeostasis and promotes regeneration following bleomycin injury in AT2 stem cells. Here, we report that in the adult lung Merlin (Nf2), the best-known upstream activator of the Hippo pathway is highly expressed in AT2 but not AT1 cells during homeostasis. We further find strong phosho-Mst1/2 staining in AT2 cells during homeostasis, suggesting that active Hippo signaling, preventing nuclear Yap/Taz localization (Figure 2A–I), is required for AT2 stem cell maintenance. Just like during lung development, AT1 cells in the adult lung were characterized by nuclear Taz staining (Figure 2D–F), suggesting that inactivation of the Hippo pathway in AT2 cells might be necessary for their differentiation into AT1 cells. Indeed, upon bleomycin injury, we find reduced phosho-Mst1/2 staining in regenerated alveolar epithelial cells (Figure 2J–L) compared to uninjured lungs (Figure 2G–I). To investigate whether inactivation of the Hippo pathway is sufficient to drive AT2 to AT1 cell differentiation we inactivated Nf2 or the downstream hippo kinases Stk4 and Stk3 (Mst1 and Mst2, respectively) in adult AT2 cells and found that this drives their spontaneous differentiation and flattening into AT1 cells (Figure 3A–H–). However, simultaneous or single inactivation of Yap1 and Wwtr1 in AT2 cells did not result in an obvious homeostatic lung phenotype in the absence of injury suggesting that Yap and/or Taz are not required for AT2 stem cell maintenance (Figure 3I–L and U and data not shown).
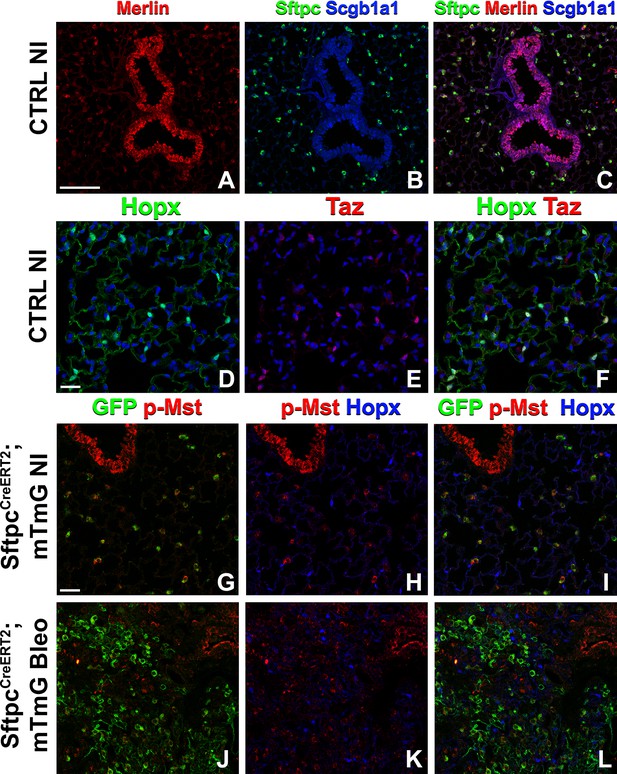
The Hippo pathway is active in alveolar type 2 (AT2) cells but not in AT1 cells.
Left lung lobes of 8-week-old mice were inflation fixed, embedded in paraffin, and sectioned. (A–C) Immunostaining for Sftpc (A–C), Merlin (A, C), and Scgb1a1 (B, C) and (D–F) immunostaining for Hopx (D, F) and Taz (E, F) on control non-injured (NI) lungs. (G–L) At 8 weeks of age, mice were placed on tamoxifen chow for 3 weeks, and left lung lobes were inflation fixed, embedded in paraffin, and sectioned 9 weeks after being placed on normal chow (at 20 weeks of age). Intratracheal bleomycin was administered 3 weeks after mice were removed from tamoxifen and placed on normal chow. Bleomycin-injured lungs were harvested at 6 weeks after injury (at 20 weeks of age). Immunostaining for GFP (G–L), phosphorylated-Mst (p-Mst; G, I, J, L), and Hopx (H, I, K, L) on non-injured (NI) SftpcCreERT2; mTmG (G–I) and on bleomycin injured SftpcCreERT2; mTmG (J–L) lungs. Representative images are presented. Scale bars, 100 µm (A–C), 50 µm (D–F), 50 µm (G–L).
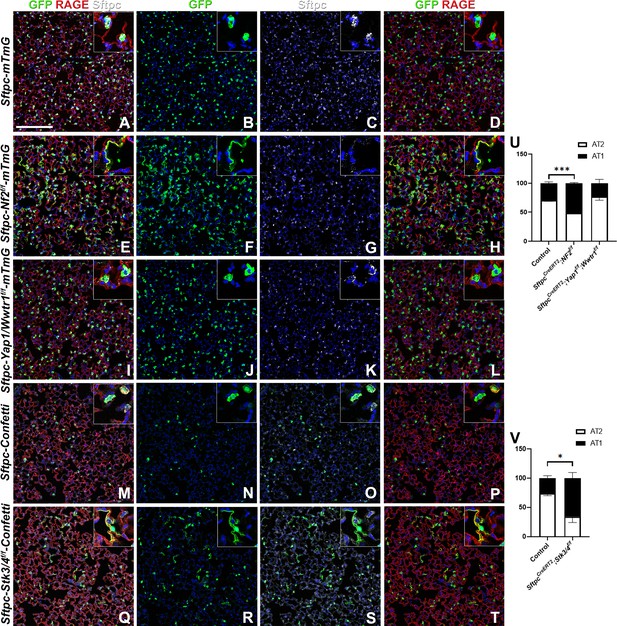
The Hippo pathway actively maintains alveolar type 2 (AT2) cells during homeostasis.
At 8 weeks of age, mice were placed on tamoxifen chow for 3 weeks, and left lung lobes were inflation fixed, embedded in paraffin, and sectioned 9 weeks after being placed on normal chow (at 20 weeks of age). (A–T) Immunostaining for GFP (B, F, J, N, R), Sftpc (C, G, K, O, S), and RAGE (D, H, L, P, T) on SftpcCreERT2; mTmG (A-D, n=4) SftpcCreERT2; Nf2f/f;mTmG (E-H, n=3), SftpcCreERT2; Yap1f/f; Wwtr1f/f; mTmG (I-L, n=4), SftpcCreERT2; Confetti (M-P, n=3), SftpcCreERT2; Stk3/4f/f;Confetti (Q-T, n=3). Representative images are presented. Scale bar, 200 µm. (U) Image analysis GFP+/Sftpc+ (AT2) and GFP+/RAGE+ (AT1) on staining in A-L and (V) M-T. Data are mean ± SEM. Student’s t-test was used to determine significance. *p<0.05, **p<0.01, ****p<0.0001.
Taz promotes alveolar epithelial regeneration and the resolution of bleomycin-induced fibrosis
To investigate the role of epithelial hippo signaling in pulmonary fibrosis, we set out to inactivate different components of the Hippo pathway in AT2 cells prior to bleomycin injury while simultaneously lineage tracing them. To investigate the requirement for Yap and/or Taz in alveolar epithelial regeneration and fibrosis development upon bleomycin injury, we inactivated Yap1 or Wwtr1 alone and in combination in AT2 cells. Inactivation of Wwtr1 alone or in combination with Yap1 caused a drastic decrease in AT2 to AT1 cell differentiation, increased pulmonary fibrosis (Figure 4A, B, E–H and K–N & Figure 4—figure supplement 1), and increased mortality in the latter (data not shown). However, the inactivation of Yap1 by itself in AT2 cells resulted in increased AT2 to AT1 cell differentiation and decreased pulmonary fibrosis based on hydroxyproline content and significantly less Col1a1 and Col3a1 RNA expression (Figure 4A–D and K–N & Figure 4—figure supplement 1). We found that Yap1 deficient alveolar epithelial cells showed increased Taz after bleomycin injury (Figure 4—figure supplement 2), in line with our previous findings that epithelial inactivation of Yap1 during lung development results in increased epithelial Taz expression and suggesting that Taz rather than Yap is the critical downstream effector of the Hippo pathway that drives AT1 cell differentiation.
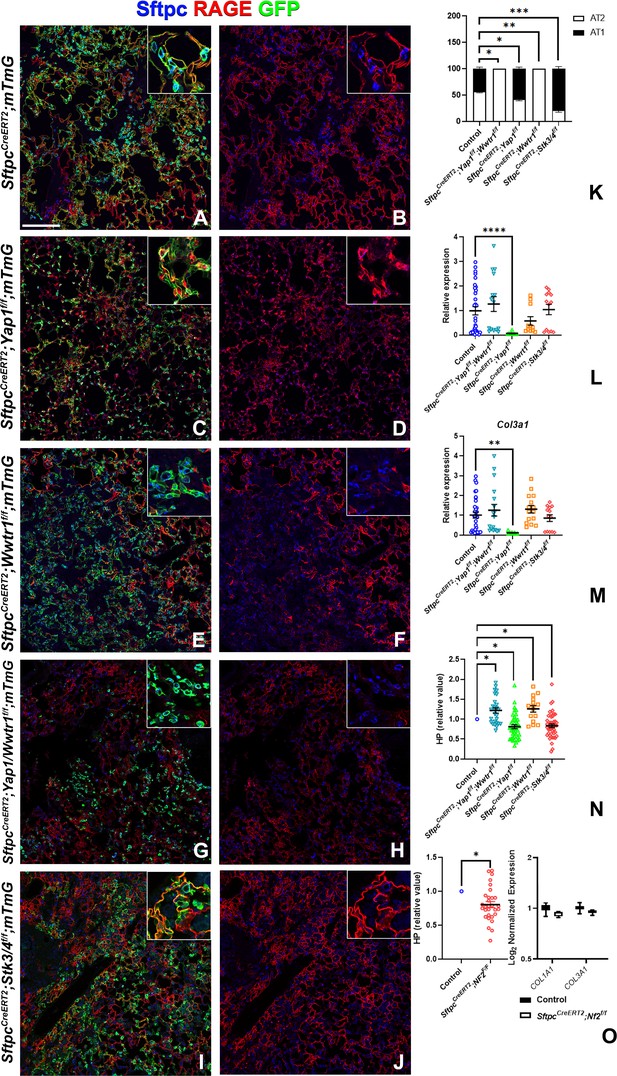
Taz is required for alveolar type 2 (AT2) into AT1 cell differentiation following bleomycin injury.
At 8 weeks of age, mice were placed on tamoxifen chow for 3 weeks, and following a 3-week washout period, mice were injured with intratracheal administration of bleomycin. At 6 weeks post-injury (at 20 weeks of age), left lung lobes were inflation fixed, embedded in paraffin, and sectioned. (A–J) Immunostaining for GFP, Sftpc, and RAGE on SftpcCreERT2; mTmG (A, B, n=3), SftpcCreERT2; Yap1f/f;mTmG (C, D, n=4), SftpcCreERT2; Wwtr1f/f; mTmG (E, F, n=5), SftpcCreERT2;Yap1f/f; Wwtr1f/f; mTmG (G, H, n=4), SftpcCreERT2; Stk3/4f/f; mTmG (I, J, f/f=6). Representative images are presented. Scale bar, 200 µm. (K) Image analysis GFP+/Sftpc+ (AT2) and GFP+/RAGE+ (AT1) on staining in A-J. (L–M) qPCR analysis for Col1a1 (L) and Col3a1 (M) on control (n=33), SftpcCreERT2; Yap1f/f; Wwtr1f/f (n=15), SftpcCreERT2; Yap1f/f (n=9), SftpcCreERT2; Wwtr1f/f (n=11), SftpcCreERT2; Stk3/4 f/f (n=13). Values are represented as 2-ΔΔCt normalized to Control. (N) Hydroxyproline analysis for soluble collagen on control, SftpcCreERT2; Yap1f/f; Wwtr1f/f (n=27, control n=25), SftpcCreERT2; Yap1f/f (n=40, control n=37), SftpcCreERT2; Wwtr1f/f (n=15, control n=10), SftpcCreERT2; Stk3/4 f/f (n=46, control n=53). Values are normalized to each genotype’s Cre- control. (O) Hydroxyproline analysis for soluble collagen on control (n=15) and SftpcCreERT2; Nf2f/f (n=30) and Log2 normalized values for RNA expression for Col1a1 and Col3a1 from NanoString analysis (control n=21, SftpcCreERT2; Nf2f/f n=6). Values are normalized to control. (K-N) Data are mean ± SEM. (O) Data are mean ± SEM (left panel) or box and whisker plot (right panel). Student’s t-test was used to determine significance. *p<0.05, **p<0.01, ****p<0.0001.
To investigate whether increased nuclear Taz would promote alveolar epithelial regeneration and fibrosis resolution, we performed bleomycin injury on mice in which we inactivated Nf2 or Stk3/4 in AT2 cells and found that this resulted in increased AT1 cell regeneration and decreased pulmonary fibrosis based on hydroxyproline content (Figure 4A, B, I, J and K–O & Figure 4—figure supplement 1). SftpcCreERT2; Nf2f/f mice also featured decreased Col1a1 and Col3a1 expression (Figure 4O).
Impaired alveolar epithelial regeneration upon inactivation of Yap/Taz in AT2 cells results in increased bronchiolization
We performed spatial transcriptomics on Control (Ctrl), SftpcCreERT2; Stk3/4f/f, and SftpcCreERT2; Yap1f/f, Wwtr1f/f lungs in the absence of injury or 6 weeks post bleomycin injury for lung epithelial cell markers and found that as expected compare to control lungs AT1 markers (Rtkn2, Cav1, Cldn18, Vegfa) were strongly reduced in SftpcCreERT2; Yap1f/f, Wwtr1f/f lungs before and after bleomycin injury (Figure 5). Compared to control lungs, the AT2 marker (Lamp3) was reduced in SftpcCreERT2; Stk3/4 f/f lungs in the absence of injury in line with our immunostaining findings that Stk3/4 inactivation in AT2 cells causes their premature differentiation into AT1 cells. Additionally, Lamp3 was reduced in SftpcCreERT2; Yap1f/f, Wwtr1f/f lungs after injury coinciding with a decrease of cells entering the S phase in SftpcCreERT2; Yap1f/f, Wwtr1f/f lungs after bleomycin injury (Figure 5—figure supplement 1). We also found an increase in AT1 markers (Rtkn2, Cav1, Cldn18, Vegfa) in SftpcCreERT2; Stk3/4 f/f lungs compared to control lungs in the absence of injury.
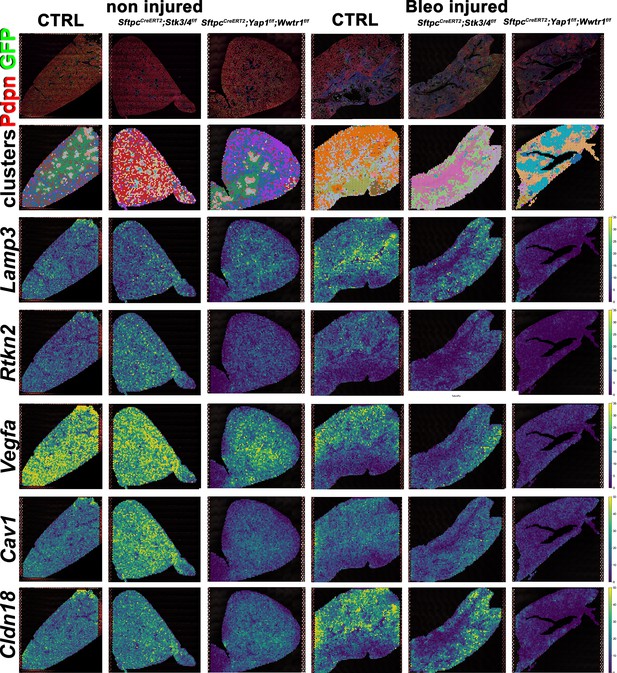
Decreased alveolar epithelial regeneration following bleomycin injury upon inactivation of Yap/Taz in alveolar type 2 (AT2) cells.
At 8 weeks of age, mice were placed on tamoxifen chow for 3 weeks, and following a 3-week washout period, mice were non-injured or injured with intratracheal administration of bleomycin. At 6 weeks post-injury (at 20 weeks of age), left lung lobes were inflation fixed, embedded in paraffin, and sectioned. Spatial transcriptomics was performed on injured control SftpcCreERT2; mTmG, SftpcCreERT2; Yap1f/f;Wwtr1f/f, mTmG, SftpcCreERT2; Stk3/4f/f; mTmG and non-injured control SftpcCreERT2; mTmG, SftpcCreERT2; Yap1f/f; Wwtr1f/f, mTmG, SftpcCreERT2; Stk3/f/ff/f; mTmG lungs. Immunofluorescence colocalization of Pdpn (red) and GFP (green) on non-injured lungs and lungs 6 weeks after bleomycin injury. Projection of spot clusters onto immunofluorescence image of the tissue sample. Spatial gene expression transcripts of cell type-specific markers were mapped onto spot coordinates. Lamp3 (AT2) and Rtkn2, Vegfa, Cav1, Cldn18 (AT1) markers. Color scale reflects the abundance of indicated transcripts.
However, unexpectedly we found increased bronchiolization (Scgb3a2, Ltf, Krt5, Krt15, Trp63) and an apparent increase in basal pods (Krt5, Krt15, Trp63) in bleomycin injured SftpcCreERT2; Yap1f/f, Wwtr1f/f lungs compared to bleomycin injured Ctrl or SftpcCreERT2; Stk3/4 f/f lungs (Figure 6). Bronchiolization is a hallmark of IPF but is only commonly observed upon severe or repetitive bleomycin injury in mice (Figure 6—figure supplement 1) Redente et al., 2021. Immunostaining and qPCR for Krt5 and Trp63 confirmed that SftpcCreERT2; Yap1f/f, Wwtr1f/f, and to a lesser extent SftpcCreERT2; Wwtr1f/f lungs showed a drastic increase in basal cell pods after bleomycin injury (Figure 7A–C). In addition, SftpcCreERT2; Yap1f/f, Wwtr1f/f lungs featured a drastic increase in Muc5b RNA expression (Figure 7A–C). Together, our findings indicate that impaired alveolar epithelial regeneration and/or increased alveolar injury upon inactivation of Yap1/Wwtr1 in AT2 cells results in increased bronchiolization. This finding is very interesting and suggests that alveolar epithelial and bronchial epithelial stem cells compete against each other to re-epithelialize the injured lung after injury. Bronchiolization in SftpcCreERT2; Yap1f/f, Wwtr1f/f lungs after bleomycin injury resembled the bronchiolization observed after catastrophic H1N1 influenza-mediated lung injury Kumar et al., 2011; Ray et al., 2016.
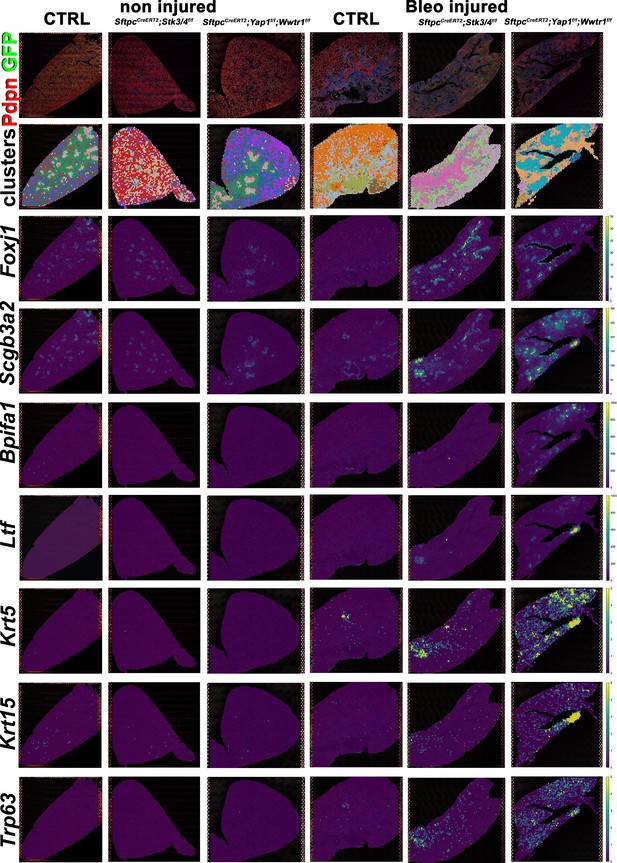
Increased bronchiolization upon Yap/Taz inactivation in alveolar type 2 (AT2) cells.
At 8 weeks of age, mice were placed on tamoxifen chow for 3 weeks, and following a 3-week washout period, mice were non-injured or injured with intratracheal administration of bleomycin. At 6 weeks post-injury (at 20 weeks of age), left lung lobes were inflation fixed, embedded in paraffin, and sectioned. Spatial transcriptomics was performed on injured (6 weeks after bleomycin injury) control SftpcCreERT2; mTmG, SftpcCreERT2; Yap1f/f;Wwtr1f/f, mTmG, SftpcCreERT2; Stk3/4f/f; mTmG and non-injured control SftpcCreERT2; mTmG, SftpcCreERT2; Yap1f/f; Wwtr1f/f, mTmG, SftpcCreERT2; Stk3/ f/ff/f; mTmG lungs. Immunofluorescence colocalization of Pdpn (red) and GFP (green) on non-injured lungs and lungs 6 weeks after bleomycin injury. Projection of spot clusters onto immunofluorescence image of the tissue sample. Spatial gene expression transcripts of cell type-specific markers were mapped onto spot coordinates. Foxj1 (ciliated), Scgb3a2 (serous and club), Bpifa1, Ltf (serous) and Krt5, Krt15, Trp63 (basal) markers. Color scale reflects the abundance of indicated transcripts.
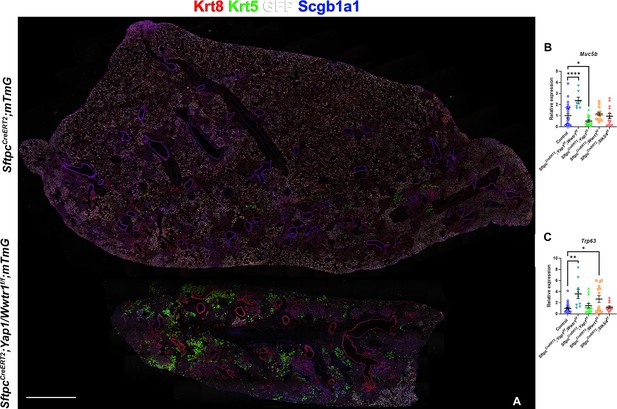
Inactivation of Yap and Taz in alveolar type 2 (AT2) cells enhances bronchiolization following bleomycin injury.
At 8 weeks of age, mice were placed on tamoxifen chow for 3 weeks, and following a 3-week washout period, mice were injured with intratracheal administration of bleomycin. At 6 weeks post-injury (at 20 weeks of age), left lung lobes were inflation fixed, embedded in paraffin, and sectioned. (A) Immunostaining for GFP, Krt8, Krt5, and Scgb1a1 on control SftpcCreERT2; mTmG and SftpcCreERT2; Yap1f/f; Wwtr1f/f, mTmG. Scale bar, 666 µm. (B–C) qPCR analysis for Muc5b (B) and Trp63 (I), on control (n=29), SftpcCreERT2; Yap1f/f; Wwtr1f/f (n=7), SftpcCreERT2; Yap1f/f (n=14), SftpcCreERT2; Wwtr1f/f (n=14), SftpcCreERT2; Stk3/4 f/f (n=14) lungs. Data are mean ± SEM. Student’s t-test was used to determine significance. *p<0.05, **p<0.01, ****p<0.0001.
Activation of profibrotic transcriptional programs upon inactivation of Yap/Taz in AT2 cells
We next analyzed predicted transcription factor activity in our spatial transcriptomics data and found increased Trp63 activity in SftpcCreERT2; Yap1f/f, Wwtr1f/f lungs consistent with the increase in basal cells. Differential analysis of transcription factor activity in our different mutant lungs indicated that one of the most strikingly differentially activated transcription factors was the profibrotic transcription factor Sp3 Lee et al., 2020; Kum et al., 2007; Wohlfahrt et al., 2019, the activity of which is upregulated in the bronchiolarized areas of bleomycin injured SftpcCreERT2; Yap1f/f, Wwtr1f/f lungs (Figure 7—figure supplement 1, Figure 7—figure supplement 2). While the role of Sp3 in the lung is unclear, Sp3-deficient embryos are growth retarded and invariably die at the birth of respiratory failure. We further found an upregulation in Nr1h3 (Lxra) and SRF activity in SftpcCreERT2; Yap1f/f, Wwtr1f/f lung after injury. Nr1h3 (Lxra) is an activator of SREBP-1 which suppresses Srebf1-activation of fibroblasts and the progression of pulmonary fibrosis Shichino et al., 2019. Serum response factor (SRF) on the other hand has been shown to be essential for myofibroblast differentiation and the development of pulmonary fibrosis Bernau et al., 2021. Together, these data suggest that the lack of AT1 cell differentiation leads to increased pro-fibrotic transcription factor activity and promotes fibrosis at the expense of epithelial regeneration in bleomycin-injured lungs.
Discussion
In summary, our data suggest that facilitating AT1 cell differentiation after injury promotes the resolution of pulmonary fibrosis. Our data indicate that increased nuclear Taz drives alveolar epithelial regeneration and fibrosis resolution, since both loss of Yap1 or inactivation of Stk3/4 or Nf2, which all result in increased nuclear Taz promote alveolar epithelial regeneration and fibrosis resolution, whereas inactivation of Wwtr1 alone impairs regeneration and fibrosis resolution. As such our findings clearly demonstrate that Yap and Taz play distinctive, even contrasting roles in AT2 stem cells in driving alveolar epithelial regeneration upon bleomycin injury.
While our data is partially supported by previous works, we also demonstrate a possible injury-specific regulation by Taz. In a S. pneumoniae infection injury, simultaneous inactivation of both Yap1 and Wwtr1 resulted in impaired alveolar epithelial regeneration. However, single Yap1 or Wwtr1 mutant mice had minor or no phenotypes LaCanna et al., 2019. We provide evidence that after bleomycin injury, single inactivation of Yap1 promotes alveolar regeneration by increasing nuclear Taz in AT2 cells. Additionally, in a pneumonectomy model, complete Yap1 inactivation in AT2 cells reduced their proliferation and differentiation compared to heterozygous inactivation of Yap in AT2 cells Liu et al., 2016. These findings raise the intriguing possibility that reducing Yap levels rather than complete inactivation of Yap has additional advantages in regard to promoting alveolar epithelial regeneration.
Activation of Yap and Taz has been demonstrated in fibrosis of other organs (reviewed in Kim et al., 2019 and Mia and Singh, 2022). In cardiac fibrosis, heart failure patients had increased nuclear Yap and increased proliferation of cardiac fibroblasts in left ventricular heart tissue Sharifi-Sanjani et al., 2021. In a mouse model of myocardial infarction, knocking out Yap1 and Wwtr1 or Yap1 alone in cardiac fibroblasts, reduced fibrosis after infarction Mia et al., 2022; Francisco et al., 2020. Interestingly, inactivation of Lats1/2, which results in increased nuclear Yap/Taz, in cardiomyocytes, reduced fibrosis after infarction suggesting a cell-specific response to infarction Shao et al., 2021. Additionally, in the liver, Yap/Taz was elevated in liver hepatocytes patients with fibrotic nonalcoholic steatohepatitis (NASH). Inactivation of Yap1 and Wwtr1 or Wwtr1 alone in hepatocytes reduced liver fibrosis in a mouse model of NASH Wang et al., 2016; Mooring et al., 2020. In patients with liver fibrosis, hepatic stellate cells (HSCs), the primary source of fibroblasts in liver fibrosis, feature elevated nuclear Yap compared to healthy control livers Mannaerts et al., 2015. Inhibiting the Yap-Tead binding with verteporfin prevented fibrosis development in a mouse model of liver fibrosis by inhibiting HSC activation and collagen deposition Mannaerts et al., 2015; Martin et al., 2016; Yu et al., 2019. In renal fibrosis, elevated Yap was identified in renal fibroblasts after unilateral ureteral obstruction (UUO), a mouse model of renal fibrosis, and inactivation of Yap1/Wwtr1 reduced the expression of fibronectin, collagen, and α-SMA Liang et al., 2017. Together, these data suggest that Yap and Taz are not identical twins, and understanding how each is regulated in a cell type-dependent manner in response to injury is critical to finding a therapy that can alleviate fibrosis in several organ systems.
Interestingly, while many reports suggest that Yap and Taz are equivalent and redundant, our data suggest that these Hippo effectors have nonredundant functions in terms of AT1 cell differentiation. We provide evidence that in response to bleomycin-induced pulmonary fibrosis, increased Taz through inactivation of Yap1, Stk3/4, or Nf2 is vital for AT1 cell differentiation and reduction of fibrosis. Interestingly, we found that in the absence of AT1 regeneration in SftpcCreERT2; Wwtr1f/f or SftpcCreERT2; Yap1f/f; Wwtr1f/f, there is an increase in bronchiolization, a hallmark of IPF, and increase in pro-fibrotic transcription factor activity in the latter. Thus, promoting Taz activity in alveolar stem cells may promote fibrosis resolution by inducing AT1 cell differentiation.
Materials and methods
Experimental model and subject details
Request a detailed protocolAll mice were bred and maintained in a pathogen-free environment with free access to food and water. Both male and female mice were used for all experiments. SftpcCreERT2 Chapman et al., 2011, Rosa26-mTmG (JAX 007676; RRID:IMSR_JAX:007676), Rosa26-Confetti (JAX 017492; RRID:IMSR_JAX:017492), Stk3/4 f/f (JAX 017635; RRID:IMSR_JAX:017635), Yap1f/f Xin et al., 2013, Wwtr1f/f 44, Nf2f/f Giovannini et al., 2000 mice were previously described.
For bleomycin injury, adult 8- to 12-week-old mice were intratracheally instilled with 50 uL bleomycin (0.8–2 U/kg body weight optimized for each strain, batch of bleomycin, and gender) as described previously Redente et al., 2014. For tamoxifen induction, mice were placed on tamoxifen-containing food (rodent diet with 400 mg/kg tamoxifen citrate; Harlan Teklad TD.130860) for 3 weeks and SftpcCreERT2 mice received two additional intraperitoneal tamoxifen injections (0.20 mg/g body weight, Enzo Life Sciences) in the last week of tamoxifen citrate feed. All experiments were approved by the Mayo Clinic Institutional Animal Care and Use Committee.
Immunohistochemistry and fluorescence
Request a detailed protocolAll staining was done on paraffin sections of formalin-fixed lungs or tracheas. Immunofluorescent staining was performed with the following primary antibodies: rabbit anti-Merlin (NF2; 1:250; clone A-19; sc-331; RRID:AB_2298548; Santa Cruz Biotechnology), rabbit anti-WWTR1 (Taz; 1:250; clone E115179; HPA007415; RRID:AB_1080602; Prestige Antibodies), rabbit anti-phosporylated-Mst1(Thr183)/2(Thr180) (1:200; 3681; RRID:AB_330269; Cell Signaling), goat anti-Scgb1a1 (1:200; clone T-18; sc-9772; RRID:AB_2238819; Santa Cruz Biotechnology Inc), chicken anti-GFP (1:250; GFP-1020; RRID:AB_10000240; Aves Labs Inc), rabbit anti-Keratin 5 (1:200; clone EP1601Y; MA5-14473; RRID:AB_10979451; Thermo Fisher Scientific), rabbit anti-SFTPC (1:200; WRAB-9337; RRID:AB_2335890; Seven hills bioreagents), rat anti-RAGE (1:500; Clone 175410; MAB1179; RRID:AB_2289349; R&D Systems), mouse anti-HOP (1:100; Clone E-1; sc-398703; RRID:AB_2687966; Santa Cruz Biotechnology Inc), rat anti-keratin 8 (1:100; TROMA-I; RRID:AB_531826; Developmental Studies Hybridoma Bank), and Syrian hamster anti-podoplanin (PDPN, T1a; 1:500; 8.1.1; RRID:AB_531893; Developmental Studies Hybridoma Bank). After deparaffinization, slides were rehydrated through a series of decreasing ethanol concentrations, antigen unmasked by either microwaving in citrate-based antigen unmasking solution (Vector Labs, H-3000) or by incubating sections with proteinase K (7.5 μg/ml) (Invitrogen, 25530–049) for 7 min at 37 °C. Tissue sections were then washed in TBS with 0.1% Tween-20 and blocked with 3% Bovine Serum Albumin (BSA), and 0.4% Triton in TBS for 30 min at room temperature followed by overnight incubation of primary antibodies diluted in 3% BSA, 0.1% Triton in TBS. The next day, slides were washed in TBS with 0.1% Tween-20 and incubated with secondary antibodies diluted in 3% BSA, and 0.1% Triton in TBS for 3 hr at room temperature. All fluorescent staining was performed with appropriate secondary antibodies from Jackson Immunoresearch. Slides were mounted using Vectashield (Vector Labs, H-1000). For alcian blue staining, the slides were deparaffinized and hydrated with distilled water, stained in a 1% Alcian blue solution (pH 2.5) for 30 min. After washing them in water for 2 min, the slides were dehydrated with ethanol, cleared in xylene, and mounted with a mounting medium.
Microscopy and imaging
Request a detailed protocolTissue was imaged using a micrometer slide calibrated Zeiss LSM800 Laser scanning confocal microscope using ZEN imaging software or Leica Stellaris 5 confocal microscope with LASX imaging software. Lungs were imaged using tiled stitched 20 x images covering the entire cross-section of the left or lower right lung lobe from ≥6 different lungs. Representative images were chosen. Images were processed and analyzed using Zen blue (Zeiss) or LASX and Adobe Photoshop Creative Suite 3 (Adobe) software.
Image quantification
Request a detailed protocolDifferentiation of GFP-positive cells was determined using artificial intelligence and machine learning image segmentation with Zeiss Zen Blue Intellesis or ImageJ software. The total area of GFP and GFP overlapping with different cell-specific antibody stains (Sftpc or RAGE) was determined. Image quantification and analysis were performed in a double-blinded fashion. Each quantification was ≥3 different lung images.
Quantitative real-time PCR
Request a detailed protocolTotal mRNA was extracted from lung accessory lobes stored in RNAlater (Invitrogen, AM7021) and using Total RNA Kit I (Omega Biotek, R6834-02) according to the manufacturer’s instructions. RNA concentration was determined by spectrophotometry. cDNA was generated using Maxima First Strand cDNA Synthesis (Fisher Scientific, FERK1642) according to the manufacturer’s instructions. Gene expression was analyzed by quantitative RT-PCR using TaqMan Gene Expression Assays (Applied Biosystems, 4369016) directed against the mouse targets β-glucuronidase (Mm00446953_m1), Trp63 (Mm00495788_m1), Muc5b (Mm00466391_m1), Col1a1 (Mm00801666_g1), Col3a1 (Mm01254476_m1). Quantitative real-time PCR was performed using a StepOne Plus system (Applied Biosystems). Data were presented as 2-ΔΔCt with β-glucuronidase as the internal sample control normalized to the control group. Each experiment was repeated with samples obtained from ≥6 different lung preparations.
Nanostring
Request a detailed protocolRNA was isolated from lung accessory lobes as described above. 100 ng of RNA was hybridized with a custom RNA probe panel designed by NanoString (NanoString Technologies) for 16 hr according to the manufacturer’s instructions. The RNA-probe hybridization was loaded on a NanoString cartridge and processed in a NanoString nCounter. Data were analyzed with Rosalind.bio (Rosalind, Inc) and Log2 Fold Changes were calculated and graphed. Each experiment was repeated with samples obtained from ≥3 different lung preparations.
Single nuclei RNA-sequencing
Embryonic day 18.5 (E18.5) lungs were collected from WT mice and flash-frozen in a dry ice/ethanol slurry. Frozen E18.5 tissues were homogenized with a dounce homogenizer and nuclei were isolated according to 10 x Genomics Nuclei Isolation from Embryonic Mouse Brain for Single Cell Multiome ATAC+ Gene Expression Sequencing. Nuclei viability was assessed and total nuclei were counted. ATAC and gene expression libraries were then constructed from the nuclei according to the 10 x Genomics Chromium Next GEM Single Cell Multiome ATAC+ Gene Expression User Guide. Sequencing was performed on a NextSeq 500. Alignment was performed using the ‘Cellranger count’ function provided in 10 x Genomics single-cell gene expression software.
Secondary analysis
Request a detailed protocolThe Python packages Scanpy and Muon were used to apply standard quality control metrics and unsupervised clustering for the generation of initial UMAP projections. Muon was used to integrate multiomics data.
Spatial transcriptomics
Request a detailed protocolRNA was isolated from formalin-fixed paraffin-embedded (FFPE) tissue sections using E.Z.N.A FFPE RNA Kit (Omega Bio-Tek). The RNA integrity in FFPE blocks was determined on an Agilent TapeStation. 5 μm FFPE lung sections that had a DV200% above 50 were placed within the frames of the capture areas on the active surface of the Visium spatial slide (10 x Genomics) and processed according to the manufacturer’s instructions. Tissues were stained with podoplanin (PDPN, T1a) and GFP and imaged with fluorescent secondary antibodies. Final library preparations and sequencing were completed by the Mayo Genomics Research Core according to the manufacturer’s instructions on an Illumina NextSeq. Count matrices were generated using the ‘spaceranger count’ function in Space Ranger 1.0.0. The resulting data were processed in Scanpy, Squidpy, and Decoupler. The Decoupler DoRothEA wrapper was used to predict transcription factor activity. DoRothEA is a comprehensive resource containing a curated collection of transcription factors (TFs) and their target genes.
Hydroxyproline
Request a detailed protocolThe right lobes were flash-frozen in dry ice at the time of harvest and stored at –80 °C. For acid hydrolysis, the lobes were baked in a 70 °C oven without lids for 2 days until completely dry. The weights of dry lobes were measured and 500 μl of 6 N HCl was added to each sample. The lungs were then hydrolyzed in an 85 °C oven for 2 days with occasional vortexing. The hydrolysates were cooled at room temperature and centrifuged at maximum speed for 10 min. The supernatants then were transferred to fresh 1.5 mL tubes and centrifuged at maximum speed for 10 min. Each sample or standard was diluted with citrate-acetate buffer (5% citric acid, 1.2% glacial acetic acid, 7.24% sodium acetate, and 3.4% sodium hydroxide) in a 96-well plate. Chloramine-T solution (1.4% chloramine-T, 10% N-propanol, and 80% citrate-acetate buffer) was added, and the mixture was incubated for 20 min at room temperature. Then, Ehrlich’s solution (1.27 M p-dimethylaminobenzaldehyde, 70% N-propanol, 20% perchloric acid) was added to each sample and the samples were incubated at 65 °C for 20 min. Absorbance was measured at 550 nm. Standard curves were generated for each experiment using the reagent hydroxyproline (Sigma H-1637) as a standard. The amount (μg) of hydroxyproline was calculated by comparison to the standard curve.
Quantification and statistical analysis
Request a detailed protocolAll results are expressed as mean values ± SEM. The ‘n’ represents biological replicates and can be found in the figure legends. The significance of differences between two sample means was determined by unpaired two-tailed student’s t-test (assuming unequal or equal variances as determined by the F-test of equality of variances). All datasets followed a normal distribution and p-values less than 0.05 were considered statistically significant. The number of samples to be used was based on the number of experimental paradigms multiplied by the number in each group that is necessary to yield statistically significant results based on power analysis, to reject the null hypothesis with 80% power (type I error = 0.05).
Data availability
Sequencing data have been uploaded to Dryad.
-
Dryad Digital RepositoryHippo signaling impairs alveolar epithelial regeneration in pulmonary fibrosis.https://doi.org/10.5061/dryad.tdz08kq3d
References
-
Cellular mechanisms of tissue fibrosis. 7. New insights into the cellular mechanisms of pulmonary fibrosisAmerican Journal of Physiology. Cell Physiology 306:C987–C996.https://doi.org/10.1152/ajpcell.00321.2013
-
Expression of serum response factor in the lung Mesenchyme is essential for development of pulmonary fibrosisAmerican Journal of Physiology. Lung Cellular and Molecular Physiology 321:L174–L188.https://doi.org/10.1152/ajplung.00323.2020
-
Integrin Alpha6Beta4 identifies an adult distal lung epithelial population with Regenerative potential in miceThe Journal of Clinical Investigation 121:2855–2862.https://doi.org/10.1172/JCI57673
-
Blockade of fibroblast YAP attenuates cardiac fibrosis and dysfunction through MRTF-A inhibitionJACC. Basic to Translational Science 5:931–945.https://doi.org/10.1016/j.jacbts.2020.07.009
-
Ineffectual type 2-to-type 1 alveolar epithelial cell differentiation in idiopathic pulmonary fibrosis: Persistence of the Krt8(Hi) transitional stateAmerican Journal of Respiratory and Critical Care Medicine 201:1443–1447.https://doi.org/10.1164/rccm.201909-1726LE
-
The genetic basis of idiopathic pulmonary fibrosisEuropean Respiratory Journal 45:1717–1727.https://doi.org/10.1183/09031936.00163814
-
Antifibrotic effect via the regulation of transcription factor Sp1 in lung fibrosisBiochemical and Biophysical Research Communications 363:368–374.https://doi.org/10.1016/j.bbrc.2007.08.176
-
Yap/Taz regulate alveolar regeneration and resolution of lung inflammationThe Journal of Clinical Investigation 129:2107–2122.https://doi.org/10.1172/JCI125014
-
Zeb1 mediates fibrosis in corneal endothelial Mesenchymal transition through Sp1 and Sp3Investigative Opthalmology & Visual Science 61:41.https://doi.org/10.1167/iovs.61.8.41
-
Yap/Taz deletion in Gli(+) cell-derived Myofibroblasts attenuates fibrosisJournal of the American Society of Nephrology 28:3278–3290.https://doi.org/10.1681/ASN.2015121354
-
The Hippo pathway Effector YAP controls mouse hepatic Stellate cell activationJournal of Hepatology 63:679–688.https://doi.org/10.1016/j.jhep.2015.04.011
-
Loss of Yap/Taz in cardiac fibroblasts attenuates adverse remodelling and improves cardiac functionCardiovascular Research 118:1785–1804.https://doi.org/10.1093/cvr/cvab205
-
A YAP/TAZ-induced feedback mechanism regulates Hippo pathway homeostasisGenes & Development 29:1271–1284.https://doi.org/10.1101/gad.262816.115
-
Tumor necrosis factor-alpha accelerates the resolution of established pulmonary fibrosis in mice by targeting Profibrotic lung MacrophagesAmerican Journal of Respiratory Cell and Molecular Biology 50:825–837.https://doi.org/10.1165/rcmb.2013-0386OC
-
Persistent, progressive pulmonary fibrosis and epithelial remodeling in miceAmerican Journal of Respiratory Cell and Molecular Biology 64:669–676.https://doi.org/10.1165/rcmb.2020-0542MA
-
Yes-associated protein (Yap) is up-regulated in heart failure and promotes cardiac fibroblast proliferationInternational Journal of Molecular Sciences 22:6164.https://doi.org/10.3390/ijms22116164
-
Molecular mechanisms in Progressive idiopathic pulmonary fibrosisAnnual Review of Medicine 64:265–276.https://doi.org/10.1146/annurev-med-042711-142004
-
Mechanisms of pulmonary fibrosisAnnual Review of Medicine 55:395–417.https://doi.org/10.1146/annurev.med.55.091902.103810
-
Activated alveolar epithelial cells initiate fibrosis through secretion of Mesenchymal proteinsThe American Journal of Pathology 183:1559–1570.https://doi.org/10.1016/j.ajpath.2013.07.016
Article and author information
Author details
Funding
National Heart, Lung, and Blood Institute (R35 HL161169)
- Stijn P De Langhe
National Heart, Lung, and Blood Institute (R01 HL146461)
- Stijn P De Langhe
National Heart, Lung, and Blood Institute (R01 HL132156)
- Stijn P De Langhe
National Heart, Lung, and Blood Institute (T32 HL105355)
- Rachel Warren
The funders had no role in study design, data collection and interpretation, or the decision to submit the work for publication.
Acknowledgements
This study was supported by NIH R01 HL146461, HL132156, and NIH R35 HL161169 to SDL. RW was supported by NIH T32 HL105355.
Ethics
This study was performed in strict accordance with the recommendations in the Guide for the Care and Use of Laboratory Animals of the National Institutes of Health. All of the animals were handled according to approved institutional animal care and use committee (IACUC) protocols (#A00006342-21) of the Mayo clinic.
Copyright
© 2023, Warren, Lyu et al.
This article is distributed under the terms of the Creative Commons Attribution License, which permits unrestricted use and redistribution provided that the original author and source are credited.
Metrics
-
- 2,555
- views
-
- 449
- downloads
-
- 18
- citations
Views, downloads and citations are aggregated across all versions of this paper published by eLife.
Download links
Downloads (link to download the article as PDF)
Open citations (links to open the citations from this article in various online reference manager services)
Cite this article (links to download the citations from this article in formats compatible with various reference manager tools)
Further reading
-
- Stem Cells and Regenerative Medicine
Tissue engineering strategies predominantly rely on the production of living substitutes, whereby implanted cells actively participate in the regenerative process. Beyond cost and delayed graft availability, the patient-specific performance of engineered tissues poses serious concerns on their clinical translation ability. A more exciting paradigm consists in exploiting cell-laid, engineered extracellular matrices (eECMs), which can be used as off-the-shelf materials. Here, the regenerative capacity solely relies on the preservation of the eECM structure and embedded signals to instruct an endogenous repair. We recently described the possibility to exploit custom human stem cell lines for eECM manufacturing. In addition to the conferred standardization, the availability of such cell lines opened avenues for the design of tailored eECMs by applying dedicated genetic tools. In this study, we demonstrated the exploitation of CRISPR/Cas9 as a high precision system for editing the composition and function of eECMs. Human mesenchymal stromal/stem cell (hMSC) lines were modified to knock out vascular endothelial growth factor (VEGF) and Runt-related transcription factor 2 (RUNX2) and assessed for their capacity to generate osteoinductive cartilage matrices. We report the successful editing of hMSCs, subsequently leading to targeted VEGF and RUNX2-knockout cartilage eECMs. Despite the absence of VEGF, eECMs retained full capacity to instruct ectopic endochondral ossification. Conversely, RUNX2-edited eECMs exhibited impaired hypertrophy, reduced ectopic ossification, and superior cartilage repair in a rat osteochondral defect. In summary, our approach can be harnessed to identify the necessary eECM factors driving endogenous repair. Our work paves the road toward the compositional eECMs editing and their exploitation in broad regenerative contexts.
-
- Developmental Biology
- Stem Cells and Regenerative Medicine
In holothurians, the regenerative process following evisceration involves the development of a ‘rudiment’ or ‘anlage’ at the injured end of the mesentery. This regenerating anlage plays a pivotal role in the formation of a new intestine. Despite its significance, our understanding of the molecular characteristics inherent to the constituent cells of this structure has remained limited. To address this gap, we employed state-of-the-art scRNA-seq and hybridization chain reaction fluorescent in situ hybridization analyses to discern the distinct cellular populations associated with the regeneration anlage. Through this approach, we successfully identified 13 distinct cell clusters. Among these, two clusters exhibit characteristics consistent with putative mesenchymal cells, while another four show features akin to coelomocyte cell populations. The remaining seven cell clusters collectively form a large group encompassing the coelomic epithelium of the regenerating anlage and mesentery. Within this large group of clusters, we recognized previously documented cell populations such as muscle precursors, neuroepithelial cells, and actively proliferating cells. Strikingly, our analysis provides data for identifying at least four other cellular populations that we define as the precursor cells of the growing anlage. Consequently, our findings strengthen the hypothesis that the coelomic epithelium of the anlage is a pluripotent tissue that gives rise to diverse cell types of the regenerating intestinal organ. Moreover, our results provide the initial view into the transcriptomic analysis of cell populations responsible for the amazing regenerative capabilities of echinoderms.