Hearing: Letting the calcium flow
Hearing the voice of your lover, the symphonies of Beethoven or a fire alarm, all rely on acoustic information being translated into messages that can be understood by the brain. This process depends on sensory cells known as hair cells which are housed in a hollow within the inner ear known as the cochlea. When sound waves enter the ear, their vibrations cause fluid in the cochlea to move and bend protrusions at the top of hair cells known as stereocilia (Fettiplace, 2017). This activates hair cells and triggers calcium to enter, leading to secretion of the neurotransmitter glutamate which then activates auditory nerve fibers that convey signals to the brain (Moser et al., 2020).
The calcium influx that drives glutamate release is a critical step in the process of hearing (Pangrsic et al., 2018). In most cell types, calcium enters via channels which open upon cell activation and then inactivate themselves by closing. However, the calcium channels in hair cells (known as Cav1.3) show an interesting property: they do not inactivate and remain open for as long as the hair cell is stimulated. When the Cav1.3 channel is expressed in other cell types, the calcium channel closes itself like other calcium channels (Cui et al., 2007; Yang et al., 2006), suggesting that there is something in hair cells that stops Cav1.3 from inactivating.
Calcium channels can be inactivated in a voltage-dependent or calcium-dependent manner. Previous work found that deletion of a calcium-binding protein known as CaBP2 prevented voltage-dependent inactivation (Picher et al., 2017); a mutation in the gene encoding this protein was also shown to cause human deafness (Schrauwen et al., 2012). However, calcium-dependent inactivation was mostly unaffected by the disruption of CaBP2. Moreover, deletion of another calcium-binding protein, CaBP1, did not affect calcium-mediated inactivation, nor the voltage-dependent mechanism (Yang et al., 2018). Now, in eLife, Tina Pangrsic and colleagues from the University Medical Centre Göttingen and Max Planck Institute for Multidisciplinary Science – including David Oestreicher as first author – report that CaBP1 and CaBP2 work together to prevent calcium channels from closing during hair cell stimulation (Figure 1; Oestreicher et al., 2024).
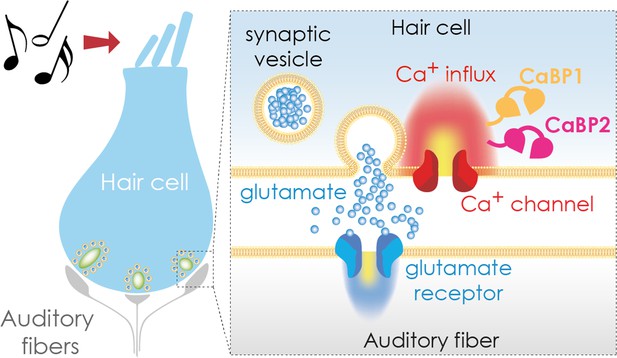
Transmitting sound from the ear to the brain.
The apical region of a hair cell (left) contains bundles of stereocilia (top) that move in response to sound waves. This movement activates the hair cell, causing calcium ions to enter the cell via calcium channels (red; right hand side inset) in the basolateral region. The calcium binding proteins CaBP1 (orange) and CaBP2 (pink) both contribute to keeping the calcium channel open for as long as the hair cell is stimulated. This leads to an influx of calcium ions (Ca2+), which trigger the release of the neurotransmitter glutamate (blue circles), which is stored in synaptic vesicles. Once secreted, glutamate travels across a synapse (white gap) to an auditory fiber (gray) that conveys the signal to the brain, enabling the perception of sound.
The team found that deleting the genes for CaBP1 and CaBP2 simultaneously led to greater inactivation of calcium channels than deleting either gene alone. As a result of the calcium channels not staying fully open, calcium influx and glutamate release were depressed, leading to reduced activation of auditory fibers. Consequently, the neural message conveyed along the auditory pathway was degraded, resulting in severe hearing loss in the genetically modified mice. Notably, even when there was a small amount of calcium influx through the temporarily open calcium channels, glutamate secretion was still almost completely abolished. This suggests that CaBP1 and CaBP2 may affect the release of glutamate independently from their role on calcium channels.
To strengthen the data, Oestreicher et al. re-expressed the gene for CaBP2 in the mice. This led to a substantial – but not complete – rescue of calcium channel activity, glutamate secretion, auditory fiber activation and hearing. Taken together, the experiments demonstrate that both CaBP1 and CaBP2 contribute to calcium-triggered glutamate release in hair cells of the inner ear, suggesting they have partially overlapping roles.
These findings also raise exciting questions for future studies. For instance, how do CaBP1 and CaBP2 work together to control calcium channel activity? Additionally, how do these calcium-binding proteins directly influence the secretion of glutamate in addition to controlling calcium channels? Further experiments that help to answer these questions, and others, could provide more insights into how hair cells transmit sound to the brain, which could potentially help identify therapeutic targets for hearing impairments.
References
-
Ca2+-binding proteins tune Ca2+-feedback to Cav1.3 channels in mouse auditory hair cellsThe Journal of Physiology 585:791–803.https://doi.org/10.1113/jphysiol.2007.142307
-
Hair cell transduction, tuning, and synaptic transmission in the mammalian cochleaComprehensive Physiology 7:1197–1227.https://doi.org/10.1002/cphy.c160049
-
Sensory processing at ribbon synapses in the retina and the cochleaPhysiological Reviews 100:103–144.https://doi.org/10.1152/physrev.00026.2018
-
Voltage-gated calcium channels: Key players in sensory coding in the retina and the inner earPhysiological Reviews 98:2063–2096.https://doi.org/10.1152/physrev.00030.2017
-
A mutation in CABP2, expressed in cochlear hair cells, causes autosomal-recessive hearing impairmentAmerican Journal of Human Genetics 91:636–645.https://doi.org/10.1016/j.ajhg.2012.08.018
-
Switching of Ca2+-dependent inactivation of Ca(v)1.3 channels by calcium binding proteins of auditory hair cellsThe Journal of Neuroscience 26:10677–10689.https://doi.org/10.1523/JNEUROSCI.3236-06.2006
Article and author information
Author details
Publication history
Copyright
© 2024, Nouvian
This article is distributed under the terms of the Creative Commons Attribution License, which permits unrestricted use and redistribution provided that the original author and source are credited.
Metrics
-
- 880
- views
-
- 50
- downloads
-
- 0
- citations
Views, downloads and citations are aggregated across all versions of this paper published by eLife.
Download links
Downloads (link to download the article as PDF)
Open citations (links to open the citations from this article in various online reference manager services)
Cite this article (links to download the citations from this article in formats compatible with various reference manager tools)
Further reading
-
- Neuroscience
Memory consolidation during sleep depends on the interregional coupling of slow waves, spindles, and sharp wave-ripples (SWRs), across the cortex, thalamus, and hippocampus. The reuniens nucleus of the thalamus, linking the medial prefrontal cortex (mPFC) and the hippocampus, may facilitate interregional coupling during sleep. To test this hypothesis, we used intracellular, extracellular unit and local field potential recordings in anesthetized and head restrained non-anesthetized cats as well as computational modelling. Electrical stimulation of the reuniens evoked both antidromic and orthodromic intracellular mPFC responses, consistent with bidirectional functional connectivity between mPFC, reuniens and hippocampus in anesthetized state. The major finding obtained from behaving animals is that at least during NREM sleep hippocampo-reuniens-mPFC form a functional loop. SWRs facilitate the triggering of thalamic spindles, which later reach neocortex. In return, transition to mPFC UP states increase the probability of hippocampal SWRs and later modulate spindle amplitude. During REM sleep hippocampal theta activity provides periodic locking of reuniens neuronal firing and strong crosscorrelation at LFP level, but the values of reuniens-mPFC crosscorrelation was relatively low and theta power at mPFC was low. The neural mass model of this network demonstrates that the strength of bidirectional hippocampo-thalamic connections determines the coupling of oscillations, suggesting a mechanistic link between synaptic weights and the propensity for interregional synchrony. Our results demonstrate the presence of functional connectivity in hippocampo-thalamo-cortical network, but the efficacy of this connectivity is modulated by behavioral state.
-
- Neuroscience
Studying infant minds with movies is a promising way to increase engagement relative to traditional tasks. However, the spatial specificity and functional significance of movie-evoked activity in infants remains unclear. Here, we investigated what movies can reveal about the organization of the infant visual system. We collected fMRI data from 15 awake infants and toddlers aged 5–23 months who attentively watched a movie. The activity evoked by the movie reflected the functional profile of visual areas. Namely, homotopic areas from the two hemispheres responded similarly to the movie, whereas distinct areas responded dissimilarly, especially across dorsal and ventral visual cortex. Moreover, visual maps that typically require time-intensive and complicated retinotopic mapping could be predicted, albeit imprecisely, from movie-evoked activity in both data-driven analyses (i.e. independent component analysis) at the individual level and by using functional alignment into a common low-dimensional embedding to generalize across participants. These results suggest that the infant visual system is already structured to process dynamic, naturalistic information and that fine-grained cortical organization can be discovered from movie data.