Vision: Paying attention
In many ways, we discover the world by looking at it. Through rapid eye movements – known as saccades – we can turn our gaze to specific objects, enabling us to pay attention to and scrutinize objects that are of high importance at the time. As well as this overt form of attention, we can also attend to objects in our periphery covertly (without moving the gaze).
Both covert and overt attention can be directed endogenously (on purpose) or exogenously – automatically attracted by a conspicuous stimulus, such as a sudden light (e.g., Carrasco, 2011). It has been shown that the brain processes required for covert attention and overt saccades are linked. Just before a saccade is directed at a target object, covert attention is already present, improving perception of the target (for example, see Deubel and Schneider, 1996; Hoffman and Subramaniam, 1995). Therefore, before a saccade begins, covert and overt attention seem to be coupled.
Most research on this topic has focused on how the coupling of covert attention and saccade planning supports visual perception. Now, in eLife, Allison Goldstein, Terrence Stanford and Emilio Salinas report insights into how paying covert attention to a peripheral object influences the subsequent saccade towards or away from the object (Goldstein et al., 2024).
The team (who are based at Wake Forest School of Medicine) asked participants to look at a central stimulus, which disappeared and was then replaced by two stimuli to the left and right. One of these peripheral stimuli (called the ‘cue’ stimulus) took one of two colors, indicating whether participants should look towards it (pro-saccade) or away from it (anti-saccade). The second peripheral stimulus, known as the non-cue, was always the same color. The location of the cue stimulus remained the same throughout a block of trials, meaning that participants knew its location and could attend to it covertly.
To investigate how this covert attendance of the cue stimulus affected the next saccade to the same or opposite location, Goldstein et al. combined the task with time pressure. Participants were instructed to saccade within a certain time after the initial center stimulus disappeared. The time between the center stimulus disappearing and the cue stimulus appearing varied. Sometimes, the cue followed shortly, informing the saccade. However, other times, the cue followed later, increasing the time pressure such that participants needed to saccade before the cue in order to meet the deadline. This was used to examine how performance (i.e., whether participants’ saccades were directed at the correct stimulus) was affected by these differences in cue time (before saccade).
The experiments showed that when the cue stimulus was visible too briefly to inform the saccade, performance was the same as it would if participants had guessed where to look (Figure 1B). When the cue was visible for longer, performance rose to a high level, indicating that the cue guided the saccade. Goldstein et al. also discovered that to saccade successfully, that is, to reach a set level of performance, participants needed the cue to be visible for about 30ms longer for anti-saccades than for pro-saccades (Figure 1B). This suggests that covert attention is directed at the cue initially, supporting saccades toward it, but this influence can be overcome quickly (in ~30ms), if the cue has indicated the saccade should be made away from the cue stimulus.
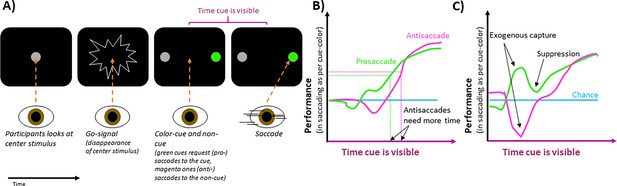
The effect of peripheral cues on intentional eye movements.
(A) In an experimental trial, participants are asked to look at a central stimulus (gray circle in first screen). This stimulus then disappears, acting as a ‘go-signal’ (second screen). After this, two stimuli appear on the left and right of the center (third screen). One, known as the cue-stimulus is colored either green (as shown) or pink, while the other (the non-cue) remains gray. A green cue requests participants to look at it, whereas a pink one requests that they look away from it, towards the non-cue stimulus (fourth screen). The location of the cue was the same across a block of trials, ensuring participants knew where to attend. If cues quickly followed the go-signal, participants had enough time to make the requested saccades. If the cues were later, time pressure was high, and participants had to guess their saccade direction. (B) For pro-saccades (green), where participants are asked to look at the cue-stimulus, performance increases with the length of time the stimulus is present before the saccade. Anti-saccades (pink), where participants were asked to look away from the cue stimulus, require the cue to be visible for a longer time to reach this same level of performance (indicated by horizontal dashed lines), which is higher than that of chance (blue line). (C) When brighter cues are used, the gaze is captured more strongly, which increases or decreases performance, depending on whether participants were requested to saccade towards or away from the cue stimulus, respectively. After this initial exogenous capture, performance briefly moves in the other direction, suggesting capture suppression.
Image credit: Figure modified from Figures 1, 2 and 3 in Goldstein et al., 2024. Please note that the graphs in (B) and (C) are simplified depictions of the overall trends shown in Goldstein et al.’s figures and do not reflect accurate values.
Bright stimuli more strongly attract covert attention and exogenous overt saccades. By manipulating the brightness of cue and non-cue stimuli using dark backgrounds, Goldstein et al. influenced how saccades were automatically attracted to the stimuli, irrespective of whether the cue was pro- or anti-saccade. Brighter stimuli captured the gaze more strongly. Therefore, if participants were to look at a brighter cue stimulus, performance increased for a brief period (Figure 1C).
Interestingly, after this exogenous influence on performance, the relationship between performance and cue-viewing-time quickly moved in the opposite direction. This suggests that after the automatic capture of gaze, the exogenous influence seemed suppressed and facilitated saccades in the opposite direction. The brief suppression worked against the endogenous control of the saccade based on the cue, which manifested later as a gradual rise of performance toward its maximum. This suggests that the two forms of saccade control rely on independent underlying mechanisms.
In sum, Goldstein et al.’s findings reveal that saccades depend on where voluntary, covert attention is deployed before, but that this dependency can be overcome quickly. They also show that the gaze is attracted by conspicuous stimuli automatically, but that this attraction is suppressed afterwards, and eye movements become intentionally driven. The findings are based on a time pressure task that can require saccades to both possible locations to be planned before the cue, resulting in guesses if time pressure becomes too high. It is an open question how covert attention affects the next intentional saccade in more naturalistic situations, where upcoming saccades cannot be planned in such a way.
References
-
Visual attention: the past 25 yearsVision Research 51:1484–1525.https://doi.org/10.1016/j.visres.2011.04.012
-
The role of visual attention in saccadic eye movementsPerception & Psychophysics 57:787–795.https://doi.org/10.3758/BF03206794
Article and author information
Author details
Publication history
- Version of Record published: June 10, 2024 (version 1)
Copyright
© 2024, Poth
This article is distributed under the terms of the Creative Commons Attribution License, which permits unrestricted use and redistribution provided that the original author and source are credited.
Metrics
-
- 305
- views
-
- 35
- downloads
-
- 0
- citations
Views, downloads and citations are aggregated across all versions of this paper published by eLife.
Download links
Downloads (link to download the article as PDF)
Open citations (links to open the citations from this article in various online reference manager services)
Cite this article (links to download the citations from this article in formats compatible with various reference manager tools)
Further reading
-
- Biochemistry and Chemical Biology
- Neuroscience
Amyloid β (Aβ) peptides accumulating in the brain are proposed to trigger Alzheimer’s disease (AD). However, molecular cascades underlying their toxicity are poorly defined. Here, we explored a novel hypothesis for Aβ42 toxicity that arises from its proven affinity for γ-secretases. We hypothesized that the reported increases in Aβ42, particularly in the endolysosomal compartment, promote the establishment of a product feedback inhibitory mechanism on γ-secretases, and thereby impair downstream signaling events. We conducted kinetic analyses of γ-secretase activity in cell-free systems in the presence of Aβ, as well as cell-based and ex vivo assays in neuronal cell lines, neurons, and brain synaptosomes to assess the impact of Aβ on γ-secretases. We show that human Aβ42 peptides, but neither murine Aβ42 nor human Aβ17–42 (p3), inhibit γ-secretases and trigger accumulation of unprocessed substrates in neurons, including C-terminal fragments (CTFs) of APP, p75, and pan-cadherin. Moreover, Aβ42 treatment dysregulated cellular homeostasis, as shown by the induction of p75-dependent neuronal death in two distinct cellular systems. Our findings raise the possibility that pathological elevations in Aβ42 contribute to cellular toxicity via the γ-secretase inhibition, and provide a novel conceptual framework to address Aβ toxicity in the context of γ-secretase-dependent homeostatic signaling.
-
- Computational and Systems Biology
- Neuroscience
Normal aging leads to myelin alterations in the rhesus monkey dorsolateral prefrontal cortex (dlPFC), which are positively correlated with degree of cognitive impairment. It is hypothesized that remyelination with shorter and thinner myelin sheaths partially compensates for myelin degradation, but computational modeling has not yet explored these two phenomena together systematically. Here, we used a two-pronged modeling approach to determine how age-related myelin changes affect a core cognitive function: spatial working memory. First, we built a multicompartment pyramidal neuron model fit to monkey dlPFC empirical data, with an axon including myelinated segments having paranodes, juxtaparanodes, internodes, and tight junctions. This model was used to quantify conduction velocity (CV) changes and action potential (AP) failures after demyelination and subsequent remyelination. Next, we incorporated the single neuron results into a spiking neural network model of working memory. While complete remyelination nearly recovered axonal transmission and network function to unperturbed levels, our models predict that biologically plausible levels of myelin dystrophy, if uncompensated by other factors, can account for substantial working memory impairment with aging. The present computational study unites empirical data from ultrastructure up to behavior during normal aging, and has broader implications for many demyelinating conditions, such as multiple sclerosis or schizophrenia.