Microscopy: Imaging far and wide
The idea of a microscope typically conjures up thoughts of a highly magnified image of a tiny sample captured in unprecedented detail. In principle, however, the physical mechanisms that allow us to see such microscopic details do not actually require the observed region or the specimen itself to be particularly small. Rather, the performance of a microscope is primarily limited by the diffraction of light as it passes through the various optical elements in the instrument.
The resolution of a microscope, , is defined as the shortest distance between two points on a specimen that can still be seen as two distinct points in the image. In 1873 Ernst Abbe showed that diffraction limited the resolution of a microscope to , where is the wavelength of the light, is the refractive index of the medium the light travels through, and is the half-angle at which the objective captures light from the sample: is also known as the "numerical aperture" of the objective.
As Abbe's equation makes clear, the resolution is independent of the magnification. Rather, it is the design of the objective that determines both the resolution of the microscope and its "field-of-view" (that is, the overall size of the sample that can be imaged). And although a variety of microscope objectives are available from commercial manufacturers, they are designed to either capture a fairly large field-of-view (typically a millimeter or so across) at low resolution, or a small field-of-view (typically a few hundred micrometers or less across) at high resolution. By contrast, to obtain a high-resolution image of a large specimen we need a combination of a large field-of-view, a long working distance (that is, the distance between the objective and the focal plane of the microscope), and a high numerical aperture: however, there are no commercial microscopes that offer this combination at present.
Now, in eLife, Gail McConnell of Strathclyde University and colleagues – Johanna Trägårdh, Rumelo Amor, John Dempster, Es Reid and Brad Amos (who is also at the MRC Laboratory for Molecular Biology) – report that they have developed an objective called the Mesolens that offers both a relatively large field-of-view and a relatively high resolution (Figure 1; McConnell et al., 2016). Using a custom-built confocal microscope equipped with the Mesolens, McConnell et al. were able to capture beautiful single-shot images of whole mouse embryos with cellular resolution, and also capture images of explants from the brains of rat embryos, again with cellular resolution.
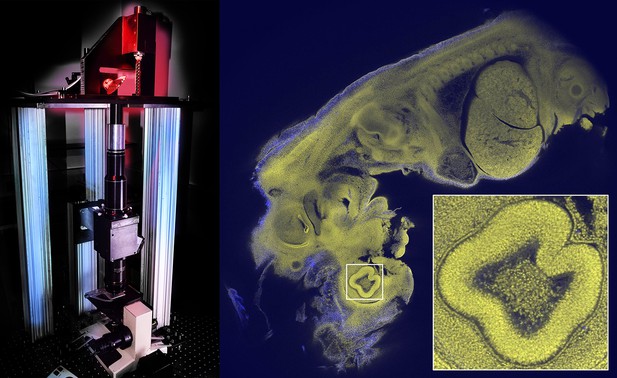
Imaging large samples with cellular resolution.
The Mesolens microscope (left) contains a scanning system with two large beryllium mirrors (top; the mirror on the right can be seen side-on), a scan lens (black and silver cylinders), the Mesolens (two black cylinders, black cube and lower black cylinder), and a stage system to translate and focus the specimen (at the base). The Mesolens (which is 550 mm in length) is an immersion lens, and matching the immersion medium to the optical properties of the specimen greatly reduces spherical aberration, which is a common problem in light microscopy. The Mesolens design also incorporates corrections for both flat-field and chromatic aberration over a range of wavelengths. Image of a 12.5 day old mouse embryo (right); the field-of-view is 5 mm for the main image, and 0.46 mm for the inset; see Figure 5 of McConnell et al. for more details. Images courtesy of David Blatchford (left) and Johanna Trägårdh (right).
The Mesolens was made possible by a combination of sophisticated optical design and skillful engineering, including excellent correction for the various optical aberrations that would otherwise compromise high-resolution imaging over a large field-of-view. Moreover, the Mesolens is an immersion lens, which means that the gap between the objective and the sample is filled with a medium such as oil, water or glycerol: McConnell et al. designed their lens to provide high-quality images in a variety of immersion media with different optical properties.
A confocal microscope is able to image a sample in all three dimensions by taking two-dimensional images at a range of different depths: the Mesolens allowed McConnell et al. to image volumes of up to 6 x 6 x 3 mm3 with a lateral resolution of 0.7–0.8 micrometers and an axial resolution of 7–8 micrometers (with a numerical aperture of 0.47). These figures are factors of at least two and three higher than the resolutions that can be achieved with currently available large field-of-view commercial objectives.
In addition to applications in developmental biology and embryology, including whole-embryo phenotyping and screening applications that require rapid imaging of large specimens, the ability to image samples measuring several millimeters across with near-cellular resolution will be useful in many other areas of the life sciences. In neuroscience, for example, researchers are trying to combine techniques such as tissue clearing and tissue expansion (Höckendorf et al., 2014; Marx, 2016) with large-volume light microscopy (Ji et al., 2016) to image entire mammalian brains at high resolution; the Mesolens could prove useful in these efforts. And extending beyond its application in confocal microscopy, the Mesolens could have applications in light-sheet microscopy (Keller and Ahrens, 2015), which excels in the rapid imaging of large volumes. In particular, combining the Mesolens with other techniques could reduce the need to use "optical tiling" when imaging large specimens, or to subject large specimens to sectioning and other destructive sample-preparation techniques.
The Mesolens highlights the increasingly important role of custom optical designs in improving the performance of light-based microscopes. Recent, complementary efforts in mesoscale imaging with two-photon microscopy also rely heavily on custom-built objectives. For example, the two-photon random access microscope developed by Karel Svoboda of the HHMI Janelia Research Campus and co-workers features a custom objective with a numerical aperture of 0.6 and a field-of-view of 5 millimeters, and enables imaging up to a depth of 1 millimeter (Sofroniew et al., 2016). The twin-region, panoramic two-photon microscope (Trepan2p) developed by Spencer Smith of the University of North Carolina and co-workers features a custom objective with a numerical aperture of 0.43 and a field of view of 3.5 millimeters, and has been used to image multiple cortical areas in mice (Stirman et al., 2016). Other examples of this emerging trend to develop new microscopes with custom-built optics include lattice light-sheet microscopy (Chen et al., 2014) and IsoView light-sheet microscopy (Chhetri et al., 2015).
By extending the scale of optical microscopy to several millimeters, this new generation of custom-built objectives also emphasizes the need for new wide-field detection systems that can take full advantage of the capabilities of these new lenses. To optimally sample the large field-of-view captured by the Mesolens in a wide-field microscope, gigapixel cameras will be needed: for comparison, a state-of-the-art scientific camera will typically have 4–6 megapixels. Various gigapixel designs are already under development (Brady et al., 2012; Zheng et al., 2014), and further advances in this domain will help exploit the full potential of the Mesolens in imaging large volumes at high resolution.
References
-
Technologies for imaging neural activity in large volumesNature Neuroscience 19:1154–1164.https://doi.org/10.1038/nn.4358
-
Optimizing probes to image cleared tissueNature Methods 13:205–209.https://doi.org/10.1038/nmeth.3774
-
0.5 gigapixel microscopy using a flatbed scannerBiomedical Optics Express 5:1–8.https://doi.org/10.1364/BOE.5.000001
Article and author information
Author details
Publication history
- Version of Record published: September 23, 2016 (version 1)
Copyright
© 2016, Chhetri et al.
This article is distributed under the terms of the Creative Commons Attribution License, which permits unrestricted use and redistribution provided that the original author and source are credited.
Metrics
-
- 2,493
- views
-
- 309
- downloads
-
- 3
- citations
Views, downloads and citations are aggregated across all versions of this paper published by eLife.
Download links
Downloads (link to download the article as PDF)
Open citations (links to open the citations from this article in various online reference manager services)
Cite this article (links to download the citations from this article in formats compatible with various reference manager tools)
Further reading
-
- Developmental Biology
- Structural Biology and Molecular Biophysics
The receptor tyrosine kinase ROR2 mediates noncanonical WNT5A signaling to orchestrate tissue morphogenetic processes, and dysfunction of the pathway causes Robinow syndrome, brachydactyly B, and metastatic diseases. The domain(s) and mechanisms required for ROR2 function, however, remain unclear. We solved the crystal structure of the extracellular cysteine-rich (CRD) and Kringle (Kr) domains of ROR2 and found that, unlike other CRDs, the ROR2 CRD lacks the signature hydrophobic pocket that binds lipids/lipid-modified proteins, such as WNTs, suggesting a novel mechanism of ligand reception. Functionally, we showed that the ROR2 CRD, but not other domains, is required and minimally sufficient to promote WNT5A signaling, and Robinow mutations in the CRD and the adjacent Kr impair ROR2 secretion and function. Moreover, using function-activating and -perturbing antibodies against the Frizzled (FZ) family of WNT receptors, we demonstrate the involvement of FZ in WNT5A-ROR signaling. Thus, ROR2 acts via its CRD to potentiate the function of a receptor super-complex that includes FZ to transduce WNT5A signals.
-
- Cell Biology
- Developmental Biology
The generation of distinct cell fates during development depends on asymmetric cell division of progenitor cells. In the central and peripheral nervous system of Drosophila, progenitor cells respectively called neuroblasts or sensory organ precursors use PAR polarity during mitosis to control cell fate determination in their daughter cells. How polarity and the cell cycle are coupled, and how the cell cycle machinery regulates PAR protein function and cell fate determination is poorly understood. Here, we generate an analog sensitive allele of CDK1 and reveal that its partial inhibition weakens but does not abolish apical polarity in embryonic and larval neuroblasts and leads to defects in polarisation of fate determinants. We describe a novel in vivo phosphorylation of Bazooka, the Drosophila homolog of PAR-3, on Serine180, a consensus CDK phosphorylation site. In some tissular contexts, phosphorylation of Serine180 occurs in asymmetrically dividing cells but not in their symmetrically dividing neighbours. In neuroblasts, Serine180 phosphomutants disrupt the timing of basal polarisation. Serine180 phosphomutants also affect the specification and binary cell fate determination of sensory organ precursors as well as Baz localisation during their asymmetric cell divisions. Finally, we show that CDK1 phosphorylates Serine-S180 and an equivalent Serine on human PAR-3 in vitro.