Neuropharmacology: How ketamine helps to overcome depression
Depression affects hundreds of millions of people and is one of the leading causes of disability worldwide. Unfortunately, current treatments for depression are limited in their efficacy because their therapeutic benefits can take several weeks before setting in and because they are only effective in approximately one third of people with depression. Individuals with treatment-resistant depression often suffer greatly in the absence of an effective drug treatment, which can lead to suicide in severe cases.
Several studies have suggested that ketamine may hold the key to developing a fast-acting antidepressant therapy. Ketamine targets a large protein complex found on the surface of neurons called the N-methyl-D aspartate receptor (NMDAR). Studies have shown that blocking NMDAR proteins with ketamine reverses depressive-like behavior more quickly and more effectively than traditional antidepressant medications (Zarate et al., 2006; Li et al., 2010; Autry et al., 2011). It can also reduce suicidal thoughts in individuals with treatment-resistant depression (DiazGranados et al., 2010). However, the popularity of ketamine as a recreational drug has led to recent research focusing on how it works rather than exploring its potential to treat depression.
One way that ketamine exerts its antidepressant effects is by increasing protein synthesis and increasing excitatory activity (as opposed to inhibitory activity) in neurons (Li et al., 2010). Also, it has similar effects to drugs that specifically block one of the subunits of the NMDAR protein, known as GluN2B (Li et al., 2010). Chemicals that block this subunit were demonstrated to have fast acting antidepressant effects in both humans and rodents (Preskorn et al., 2008; Li et al., 2010). However, not all NMDAR proteins contain this subunit, so it is not clear if drugs that block the GluN2B subunit specifically and ketamine (which blocks all NMDAR proteins) have similar effects on protein synthesis and the excitatory activity of neurons. Now, in eLife, Benjamin Hall and colleagues from Tulane University and Vanderbilt University Medical Center—including Oliver Miller and Lingling Yang as joint first authors—address this question (Miller et al., 2014).
To study how ketamine influences NMDAR proteins, Miller, Yang et al. generated a mouse line (called 2BΔCtx) that lacks the GluN2B subunit in excitatory neurons in the cortex. They assessed the baseline levels of depressive-like characteristics of the 2BΔCtx mice using two tests—the tail suspension test and the forced swim test. In these tests, mice with depressive-like characteristics will spend less time moving compared to wild-type mice: this reflects one of the symptoms of depression in humans known as behavioral despair.
The 2BΔCtx mice spent more time moving than their wild-type counterparts during both tests, suggesting that they have lower levels of depressive-like behavior. In fact, the effect was so strong that ketamine—which increased the amount of time the wild-type mice were moving during the tests—had no impact on the 2BΔCtx mice.
Previous work had shown that ketamine leads to an increase in the transmission of excitatory signals in neurons, so Miller, Yang et al. hypothesized that these changes would be mimicked in the 2BΔCtx mice (Li et al., 2010). Indeed, the 2BΔCtx mice had higher levels of excitatory activity in the prefrontal cortex of the brain, and ketamine—which increased excitatory activity in the wild-type mice—had no additional effect. These results indicate that the antidepressant action of ketamine may be caused by the changes in the excitation of neurons.
The 2BΔCtx mice also allowed Miller, Yang et al. to study how ketamine reduces depressive-like behavior, and how NMDAR subunits are involved, in greater detail. It had been shown previously that ketamine increased the levels of specific proteins in the cortex. However, the overall levels of protein synthesis following ketamine treatment have not been measured (Li et al., 2010). Using a method of protein tagging called FUNCAT—which makes it possible to see when proteins are being made (Dieterich et al., 2010)—they confirmed that there is an increase in the baseline levels of protein synthesis in neurons from 2BΔCtx mice compared to neurons from wild-type mice.
Finally, this work raises the question of how ketamine seems to produce similar effects as chemicals that specifically target the GluN2B subunit. The complete NMDAR protein is made of four subunits that fit together to form a channel across the cell membrane. The neurotransmitter glutamate activates NMDAR proteins via the GluN2B subunit to open the channel and allow positively charged ions to pass through the membrane, which leads to a series of events that inhibit protein synthesis. The FUNCAT data shows that NMDAR proteins containing a GluN2B subunit are constantly active: Miller, Yang et al. suggest that this is due to the low levels of glutamate found in the neurons (Figure 1) (Meldrum, 2000). By treating 2BΔCtx mice with several different chemicals, they elegantly showed that the flow of positive ions across the membrane generated by low levels of glutamate was due to NMDAR proteins that contained GluN2B subunits.
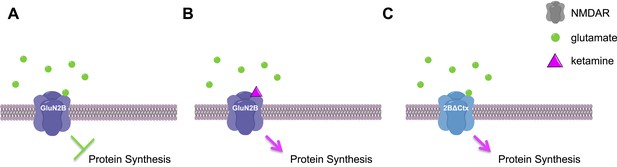
Ketamine increases protein synthesis in neurons and decreases depressive-like behaviors.
(A) In the neurons of wild-type mice, low levels of glutamate (green circles) activate NMDAR proteins that contain GluN2B subunits, leading to a decrease in protein synthesis and an increase in depressive-like behaviors. (B) If ketamine (purple triangle) is administered to these mice it blocks the NMDAR proteins, leading to an increase in protein synthesis and a decrease in depressive-like behaviors. (C) The NMDAR proteins in 2BΔCtx mice—which lack the GluN2B subunit in cortical neurons—cannot be activated by glutamate, so there is an increase in protein synthesis and a decrease in depressive-like behaviors, even in the absence of ketamine. This image was generated using ChemDraw.
Harnessing the properties of ketamine into a more specific drug therapy has been a goal of many researchers since the discovery of ketamine as a fast-acting antidepressant. Miller, Yang et al. have made a huge leap in helping to uncover exactly how ketamine may be working. Although drugs that target the GluN2B subunit also have been reported to have antidepressant properties, this latest work significantly expands on the previous literature confirming that the GluN2B subunits are viable therapeutic targets for depression.
References
-
In situ visualization and dynamics of newly synthesized proteins in rat hippocampal neuronsNature Neuroscience 13:897–905.https://doi.org/10.1038/nn.2580
-
Glutamate as a neurotransmitter in the brain: review of physiology and pathologyThe Journal of Nutrition 130:1007S–1015S.
-
An innovative design to establish proof of concept of the antidepressant effects of the NR2B subunit selective N-methyl-D-aspartate antagonist, CP-101,606, in patients with treatment-refractory major depressive disorderJournal of Clinical Psychopharmacology 28:631–637.https://doi.org/10.1097/JCP.0b013e31818a6cea
-
A randomized trial of an N-methyl-D-aspartate antagonist in treatment-resistant major depressionArchives of General Psychiatry 63:856–864.https://doi.org/10.1001/archpsyc.63.8.856
Article and author information
Author details
Publication history
Copyright
© 2014, Huynh and Klann
This article is distributed under the terms of the Creative Commons Attribution License, which permits unrestricted use and redistribution provided that the original author and source are credited.
Metrics
-
- 3,134
- views
-
- 144
- downloads
-
- 1
- citations
Views, downloads and citations are aggregated across all versions of this paper published by eLife.
Download links
Downloads (link to download the article as PDF)
Open citations (links to open the citations from this article in various online reference manager services)
Cite this article (links to download the citations from this article in formats compatible with various reference manager tools)
Further reading
-
- Cell Biology
Endometriosis is a debilitating disease affecting 190 million women worldwide and the greatest single contributor to infertility. The most broadly accepted etiology is that uterine endometrial cells retrogradely enter the peritoneum during menses, implant and form invasive lesions in a process analogous to cancer metastasis. However, over 90% of women suffer retrograde menstruation, but only 10% develop endometriosis, and debate continues as to whether the underlying defect is endometrial or peritoneal. Processes implicated in invasion include: enhanced motility; adhesion to, and formation of gap junctions with, the target tissue. Endometrial stromal (ESCs) from 22 endometriosis patients at different disease stages show much greater invasiveness across mesothelial (or endothelial) monolayers than ESCs from 22 control subjects, which is further enhanced by the presence of EECs. This is due to enhanced responsiveness of endometriosis ESCs to the mesothelium, which induces migration and gap junction coupling. ESC-PMC gap junction coupling is shown to be required for invasion, while coupling between PMCs enhances mesothelial barrier breakdown.
-
- Cell Biology
How the fate (folding versus degradation) of glycoproteins is determined in the endoplasmic reticulum (ER) is an intriguing question. Monoglucosylated glycoproteins are recognized by lectin chaperones to facilitate their folding, whereas glycoproteins exposing well-trimmed mannoses are subjected to glycoprotein ER-associated degradation (gpERAD); we have elucidated how mannoses are sequentially trimmed by EDEM family members (George et al., 2020; 2021 eLife). Although reglucosylation by UGGT was previously reported to have no effect on substrate degradation, here we directly tested this notion using cells with genetically disrupted UGGT1/2. Strikingly, the results showed that UGGT1 delayed the degradation of misfolded substrates and unstable glycoproteins including ATF6α. An experiment with a point mutant of UGGT1 indicated that the glucosylation activity of UGGT1 was required for the inhibition of early glycoprotein degradation. These and overexpression-based competition experiments suggested that the fate of glycoproteins is determined by a tug-of-war between structure formation by UGGT1 and degradation by EDEMs. We further demonstrated the physiological importance of UGGT1, since ATF6α cannot function properly without UGGT1. Thus, our work strongly suggests that UGGT1 is a central factor in ER protein quality control via the regulation of both glycoprotein folding and degradation.