Mathematical Modeling: Telomeres open a window on stem cell division
Stem cells are undifferentiated cells that can develop into more specialized cells. There are two kinds of stem cells: embryonic stem cells and adult stem cells. Embryonic stem cells are active during early development and give rise to all the different cell types in the body. Adult stem cells are specific to each tissue and give rise to all the specialized cells in a particular tissue or organ. When a stem cell divides, each new cell has the potential to either remain a stem cell or differentiate into a more specialized type of cell (Figure 1A). However, it can be difficult to analyze these division patterns in humans. Now, in eLife, Benjamin Werner, Fabian Beier, Arne Traulsen and colleagues have used a mathematical model to reconstruct the dynamics of blood stem cells from measurements of telomere length (Werner et al., 2015).
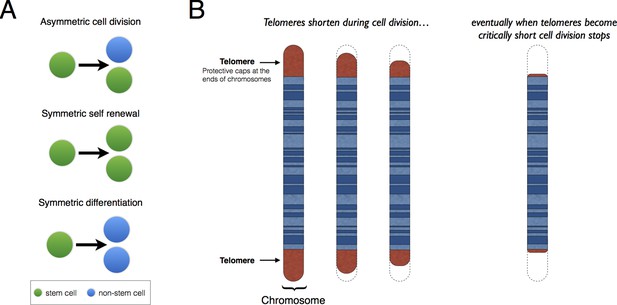
Patterns of stem cell division and the protective role of telomeres.
(A) Stem cells can divide asymmetrically to produce a new stem cell and a non-stem cell that can differentiate to replace other types of cells that are lost from the tissue because of cell death (top). Stem cells can also divide symmetrically, producing two daughter stem cells (symmetric self-renewal, middle) or two non-stem cells (symmetric differentiation, bottom). (B) Telomeres are the regions of DNA that cap and protect the ends of linear chromosomes. Each time a cell divides the telomeres get shorter. When telomeres become too short, cell division stops.
Telomeres are lengths of DNA that cap both ends of linear chromosomes (Figure 1B), and they protect the chromosomes by preventing their natural ends from being interpreted as breaks in the DNA. During cell division, the enzymes that duplicate DNA cannot copy the very ends of chromosomes; this ‘end-replication problem’ is part of the reason why the telomeres get shorter each time a cell divides (Martinez and Blasco, 2015). When telomeres become very short, they lose their protective properties and cell division stops. This process is known as ‘replicative senescence’ and is correlated with aging: put simply, telomeres get shorter as people get older.
Replicative senescence is believed to have evolved as a means to curb excessive cell division, which is a hallmark of cancer. However, human cancers find ways to bypass this process, typically by expressing an enzyme called telomerase that acts to lengthen the telomeres. Telomerase is highly active in embryonic stem cells, but it is not expressed in most normal cells.
Werner, Beier, Traulsen and colleagues – who are based at the Max Planck Institute for Evolutionary Biology, RWTH Aachen University Hospital, University Hospital Zürich and the Mayo Clinic – measured the average telomere lengths from blood samples taken from 356 individuals aged between 0 and 85 years old. Two alternative models of stem cell dynamics were then analyzed. The first model considered that the stem cells only divide asymmetrically, producing one stem cell and one non-stem cell. The second model included both asymmetric cell division and symmetric self-renewal (where a stem cell divides to form two daughter stem cells; Figure 1A). Werner, Beier et al. found that the first model predicted a linear relationship between average telomere length and the donor’s age, whereas the second model predicted a nonlinear decrease in telomere length. The data strongly favored the second model.
The findings suggest that symmetric self-renewal is more frequent during adolescence. Since symmetric self-renewal could promote the accumulation of mutations (Tomasetti and Vogelstein, 2015), this has implications for understanding how cancer emerges. A previous theoretical study argued that the high number of cell divisions that occur during fetal development puts us at risk of acquiring mutations even before birth (Frank and Nowak, 2003). The new results extend this argument into childhood and adolescence. That is, before adulthood is reached, there is possibly a relatively high risk of acquiring mutations that may predispose an individual to cancer – even if the onset of cancer typically occurs much later in life.
An important question that arises from this study concerns the exact nature of the cell divisions that ensure tissue maintenance in adulthood. In the model of Werner, Beier et al., tissues are maintained in adulthood through asymmetric cell divisions. However, as they point out, this model cannot be mathematically distinguished from an alternative mechanism that relies on a mixture of symmetric self-renewal and symmetric differentiation (i.e., when the stem cell divides to produce two non-stem cells). This is because tissues can also be maintained if the probabilities of symmetric self-renewal and differentiation are balanced and controlled through feedback loops (Lander et al., 2009); this latter model is supported by stem cell data from both humans and other animals (see Shahryiari and Komarova, 2013 for references). Some mathematical models suggest that the prevalence of mutations depends on the division patterns, so it may be possible to distinguish between them mathematically (Shahryiari and Komarova, 2013).
Using telomere length as an indicator of biological processes is not a new idea. Telomere length has previously been singled out as a marker to identify adult stem cells and their location in the body (Flores et al., 2008). From a modeling perspective, the length of telomeres has also been proposed as a signal to assess the risk posed by pre-cancerous mutations in healthy individuals (Rodriguez-Brenes et al., 2014). Telomere length might also help predict the success rate of cancer therapies, and studies of telomerase inhibitors predict better outcomes for patients with short telomere length (Chiappori et al., 2015). The work by Werner, Beier et al. adds an important contribution in this context, allowing us to use telomere lengths to gain insights into cell division patterns that occur in vivo.
References
-
The longest telomeres: a general signature of adult stem cell compartmentsGenes & Development 22:654–667.https://doi.org/10.1101/gad.451008
-
Replicating through telomeres: a means to an endTrends in Biochemical Sciences 40:504–515.https://doi.org/10.1016/j.tibs.2015.06.003
Article and author information
Author details
Publication history
- Version of Record published: January 25, 2016 (version 1)
Copyright
© 2016, Rodriguez-Brenes et al.
This article is distributed under the terms of the Creative Commons Attribution License, which permits unrestricted use and redistribution provided that the original author and source are credited.
Metrics
-
- 1,780
- views
-
- 296
- downloads
-
- 3
- citations
Views, downloads and citations are aggregated across all versions of this paper published by eLife.
Download links
Downloads (link to download the article as PDF)
Open citations (links to open the citations from this article in various online reference manager services)
Cite this article (links to download the citations from this article in formats compatible with various reference manager tools)
Further reading
-
- Computational and Systems Biology
- Evolutionary Biology
A comprehensive census of McrBC systems, among the most common forms of prokaryotic Type IV restriction systems, followed by phylogenetic analysis, reveals their enormous abundance in diverse prokaryotes and a plethora of genomic associations. We focus on a previously uncharacterized branch, which we denote coiled-coil nuclease tandems (CoCoNuTs) for their salient features: the presence of extensive coiled-coil structures and tandem nucleases. The CoCoNuTs alone show extraordinary variety, with three distinct types and multiple subtypes. All CoCoNuTs contain domains predicted to interact with translation system components, such as OB-folds resembling the SmpB protein that binds bacterial transfer-messenger RNA (tmRNA), YTH-like domains that might recognize methylated tmRNA, tRNA, or rRNA, and RNA-binding Hsp70 chaperone homologs, along with RNases, such as HEPN domains, all suggesting that the CoCoNuTs target RNA. Many CoCoNuTs might additionally target DNA, via McrC nuclease homologs. Additional restriction systems, such as Type I RM, BREX, and Druantia Type III, are frequently encoded in the same predicted superoperons. In many of these superoperons, CoCoNuTs are likely regulated by cyclic nucleotides, possibly, RNA fragments with cyclic termini, that bind associated CARF (CRISPR-Associated Rossmann Fold) domains. We hypothesize that the CoCoNuTs, together with the ancillary restriction factors, employ an echeloned defense strategy analogous to that of Type III CRISPR-Cas systems, in which an immune response eliminating virus DNA and/or RNA is launched first, but then, if it fails, an abortive infection response leading to PCD/dormancy via host RNA cleavage takes over.
-
- Computational and Systems Biology
Imputing data is a critical issue for machine learning practitioners, including in the life sciences domain, where missing clinical data is a typical situation and the reliability of the imputation is of great importance. Currently, there is no canonical approach for imputation of clinical data and widely used algorithms introduce variance in the downstream classification. Here we propose novel imputation methods based on determinantal point processes (DPP) that enhance popular techniques such as the multivariate imputation by chained equations and MissForest. Their advantages are twofold: improving the quality of the imputed data demonstrated by increased accuracy of the downstream classification and providing deterministic and reliable imputations that remove the variance from the classification results. We experimentally demonstrate the advantages of our methods by performing extensive imputations on synthetic and real clinical data. We also perform quantum hardware experiments by applying the quantum circuits for DPP sampling since such quantum algorithms provide a computational advantage with respect to classical ones. We demonstrate competitive results with up to 10 qubits for small-scale imputation tasks on a state-of-the-art IBM quantum processor. Our classical and quantum methods improve the effectiveness and robustness of clinical data prediction modeling by providing better and more reliable data imputations. These improvements can add significant value in settings demanding high precision, such as in pharmaceutical drug trials where our approach can provide higher confidence in the predictions made.