Olfaction: Minority odors get equal say
How well could you see a small green leaf if your world was enveloped in a bright purple haze? You would probably struggle to see it at all. But what if instead of detecting colors, you were detecting smells? In this case, you might detect the equivalent of the leaf pretty clearly, but hardly even notice the equivalent of the purple haze. Now, in eLife, Alexander Fleischman and colleagues – including Benjamin Roland, Rebecca Jordan and Dara Sosulski as joint first authors – report on the neural mechanisms that allow information about ‘minority’ odors to be detected through the overwhelming haze of another smell (Roland et al., 2016).
Scents are detected when odor molecules bind to odorant receptors on the surface of olfactory receptor neurons. These neurons then send signals to other neurons in a structure called the olfactory bulb, which processes this information and sends it to other olfactory regions in the brain.
To investigate the neural activity that underlies odor detection, Roland et al. – who are based at a number of institutions in France, Germany, the UK and the US – have revisited the ‘monoclonal nose’ mouse, an intriguing mouse model that was first reported in 2008 (Fleischmann et al., 2008). Mice normally express about 1100 subtypes of odorant receptor and scatter these evenly over several million receptor neurons (with each receptor neuron having one odorant receptor subtype). However, monoclonal nose mice express the same receptor (called M71) in 95% of their receptor neurons (Figure 1).
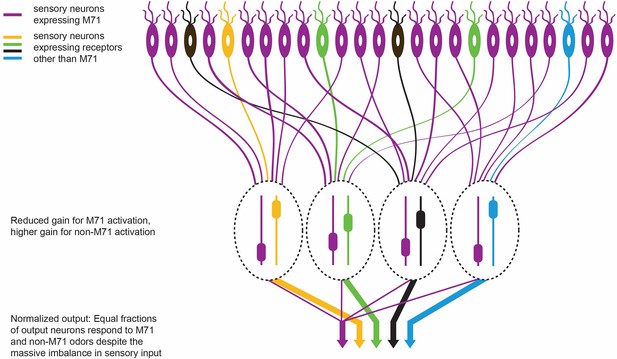
The olfactory response in M71 transgenic mice.
M71 transgenic mice are genetically modified to over-express the M71 receptor (shown in purple) in olfactory receptor neurons (top). However, mice can still detect other ‘minority’ odors because the neurons in the olfactory bulb (which receive signals from the olfactory receptor neurons) become less sensitive to signals from the dominant M71 receptors, and more sensitive to signals from other receptors (shown here in yellow, green, black and blue). The heights of the sliders in the ovals represent the level of gain in that circuit: high gain leads to high sensitivity. This ultimately enables each odor to produce an equal-sized output from the olfactory bulb (represented by the width of the arrows at the bottom of the figure).
One of the more unexpected findings of the 2008 study was the apparent inability of these mice to detect acetophenone: this was surprising because acetophenone (which is found in mouse urine) is the primary molecule that activates the M71 receptor. The study involved a behavioral task in which the animals had to discriminate unscented air from air that contained various concentrations of odors. The M71 transgenic mice were capable of quite subtle discriminations for all the tested odors except acetophenone.
Roland et al. now confirm the above behavior but also show that M71 transgenic mice start sniffing when exposed to acetophenone, suggesting that they can in fact detect this odor. If the earlier results were unexpected, the new data are even more intriguing and open up possibilities for teasing apart the mechanisms that underlie these apparently contradictory behaviors.
As a starting point, Roland et al. used a wide range of measures to examine what goes on at the output of the olfactory bulb in the M71 transgenic mice. For example, they used multiphoton imaging to record the activity of populations of neurons called mitral cells that transmit information from the bulb to the other olfactory regions of the brain. They also used whole-cell patch-clamp recordings to acquire detailed readouts from individual mitral cells in both anesthetized and awake mice. These measurements showed that the responses of the mitral cells in M71 transgenic mice are very similar to those of normal mice. The fraction of cells that respond to odors, including acetophenone, was indistinguishable from that seen in normal mice. The same was true for the time courses of the responses, and also for the dependence of odor-driven mitral cell activity on the mouse’s breathing patterns. The only apparent difference was that the output responses were more variable in the transgenic mice, and were slightly weaker to non-acetophenone odors.
This is rather impressive from a computational perspective. Not only does the olfactory system amplify responses to weak odorant inputs (that is, odors other than acetophenone) in the M71 transgenic mice, it also suppresses the massive sensory input (from acetophenone) that drives 95% of the sensory neurons (Figure 1). This normalization preserves much of the information about the detected odors despite the sensory input being hugely distorted.
How does the olfactory bulb accomplish this feat? Roland et al. trace the mechanism to a change in the balance of the inhibitory inputs to the mitral cells. These inputs increase in response to acetophenone, while there is less inhibition of other ‘minority’ odors than there is in normal mice. Based on the results of optical imaging, Roland et al. ascribe this change in the pattern of inhibition to cells called periglomerular cells that are found in the input layer of the olfactory bulb, but other explanations are also possible (Mori et al., 1999; Boyd et al., 2012; Kato et al., 2013; Miyamichi et al., 2013; Banerjee et al., 2015). Future work can build on the results of Roland et al. and isolate the contributions of the various olfactory circuit elements using modern genetic approaches and the large battery of tools that neuroscientists can use to manipulate neuronal activity.
One peculiarity that remains to be resolved is the discrepancy between the neuronal and behavioral responses of M71 transgenic mice to acetophenone. However, the study by Roland et al. sets up a powerful system for understanding the computations that occur in the first stages of the olfactory system to allow different smells to be detected.
In the language of electronic circuit theory, the olfactory bulb employs a “gain-control” layer early in processing to suppress large swings in the size of the input signals it receives. The outcome of such filtering (as seen in M71 transgenic mice) is exactly what one sees in an electronic circuit forced to find a small signal on a huge background. The circuit and bulb both become a little less sensitive and a little noisier. However, a typical electronic circuit has to do this for just one signal. The remarkable accomplishment of the olfactory bulb, as highlighted by Roland, Jordan, Sosulski, Fleischman and colleagues, is to do this for a variety of different signals.
References
Article and author information
Author details
Publication history
Copyright
© 2016, Gupta et al.
This article is distributed under the terms of the Creative Commons Attribution License, which permits unrestricted use and redistribution provided that the original author and source are credited.
Metrics
-
- 1,066
- views
-
- 134
- downloads
-
- 0
- citations
Views, downloads and citations are aggregated across all versions of this paper published by eLife.
Download links
Downloads (link to download the article as PDF)
Open citations (links to open the citations from this article in various online reference manager services)
Cite this article (links to download the citations from this article in formats compatible with various reference manager tools)
Further reading
-
- Neuroscience
The concept that dimeric protein complexes in synapses can sequentially replace their subunits has been a cornerstone of Francis Crick’s 1984 hypothesis, explaining how long-term memories could be maintained in the face of short protein lifetimes. However, it is unknown whether the subunits of protein complexes that mediate memory are sequentially replaced in the brain and if this process is linked to protein lifetime. We address these issues by focusing on supercomplexes assembled by the abundant postsynaptic scaffolding protein PSD95, which plays a crucial role in memory. We used single-molecule detection, super-resolution microscopy and MINFLUX to probe the molecular composition of PSD95 supercomplexes in mice carrying genetically encoded HaloTags, eGFP, and mEoS2. We found a population of PSD95-containing supercomplexes comprised of two copies of PSD95, with a dominant 12.7 nm separation. Time-stamping of PSD95 subunits in vivo revealed that each PSD95 subunit was sequentially replaced over days and weeks. Comparison of brain regions showed subunit replacement was slowest in the cortex, where PSD95 protein lifetime is longest. Our findings reveal that protein supercomplexes within the postsynaptic density can be maintained by gradual replacement of individual subunits providing a mechanism for stable maintenance of their organization. Moreover, we extend Crick’s model by suggesting that synapses with slow subunit replacement of protein supercomplexes and long-protein lifetimes are specialized for long-term memory storage and that these synapses are highly enriched in superficial layers of the cortex where long-term memories are stored.
-
- Neuroscience
Complex macro-scale patterns of brain activity that emerge during periods of wakeful rest provide insight into the organisation of neural function, how these differentiate individuals based on their traits, and the neural basis of different types of self-generated thoughts. Although brain activity during wakeful rest is valuable for understanding important features of human cognition, its unconstrained nature makes it difficult to disentangle neural features related to personality traits from those related to the thoughts occurring at rest. Our study builds on recent perspectives from work on ongoing conscious thought that highlight the interactions between three brain networks – ventral and dorsal attention networks, as well as the default mode network. We combined measures of personality with state-of-the-art indices of ongoing thoughts at rest and brain imaging analysis and explored whether this ‘tri-partite’ view can provide a framework within which to understand the contribution of states and traits to observed patterns of neural activity at rest. To capture macro-scale relationships between different brain systems, we calculated cortical gradients to describe brain organisation in a low-dimensional space. Our analysis established that for more introverted individuals, regions of the ventral attention network were functionally more aligned to regions of the somatomotor system and the default mode network. At the same time, a pattern of detailed self-generated thought was associated with a decoupling of regions of dorsal attention from regions in the default mode network. Our study, therefore, establishes that interactions between attention systems and the default mode network are important influences on ongoing thought at rest and highlights the value of integrating contemporary perspectives on conscious experience when understanding patterns of brain activity at rest.