Endoplasmic Reticulum: Keeping in shape
The endoplasmic reticulum is the largest single structure in eukaryotic cells. It consists of a range of interconnected shapes, including sheets and tubules, and comprises a lumen enclosed by a membrane that is continuous with the membrane that surrounds the nucleus of the cell (Figure 1). The structure and dynamic nature of the endoplasmic reticulum allow it to be involved in many processes in cells: these processes include protein production and degradation, cell signaling, and the synthesis and distribution of lipids and fat molecules. Form follows function, and understanding how the distinct shapes of the endoplasmic reticulum are regulated and maintained is currently an area of intense interest in cell biology (Goyal and Blackstone, 2013; Westrate et al., 2015).
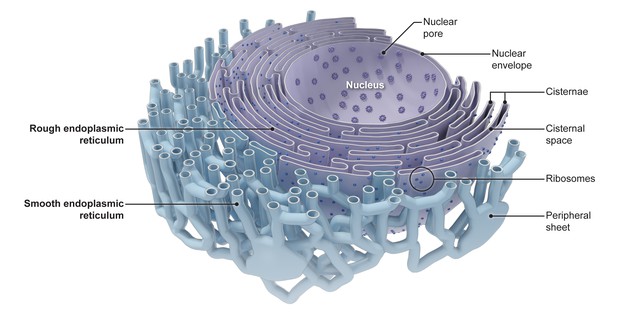
The endoplasmic reticulum consists of various interconnected shapes.
At the center of the cell, the nuclear envelope contains pores that control what molecules enter and exit the nucleus. The nuclear envelope is also connected to the stacked sheets (cisternae) of the rough endoplasmic reticulum, which is specialized for protein production. From the rough endoplasmic reticulum, the tubules of the smooth endoplasmic reticulum (blue) form a network that extends across the cell and is interspersed with sheet-like structures (peripheral sheets). From Goyal and Blackstone (2013).
Image credit: Goyle and Blackstone (public domain).
Over the past decade, several proteins that shape the endoplasmic reticulum have been identified. In many cases, these proteins are evolutionarily conserved across eukaryotes, from yeast to mammalian cells. Membrane proteins of the reticulon and REEP families can generate curves in membranes and act to maintain the tubules (Voeltz et al., 2006). Atlastin proteins mediate the tethering and fusion of tubules to one other to form three-way junctions (Hu et al., 2009; Orso et al., 2009), which appear to be stabilized by a membrane protein called lunapark (Shemesh et al., 2014; Chen et al., 2015). Several other proteins help the endoplasmic reticulum to maintain contact with the cell membrane, other cell compartments and the cytoskeleton. Increasingly, studies have revealed dynamic changes in the shape of the endoplasmic reticulum in processes such as cell division and during electrical activity in neurons (Goyal and Blackstone, 2013; Phillips and Voeltz, 2016).
Proteins involved in shaping the endoplasmic reticulum have mostly been studied individually, even though they are known to interact with one another. Now, in eLife, Tom Rapoport and co-workers at Harvard Medical School – including Songyu Wang, Hanna Tukachinsky and Fabian Romano – report on how three key proteins work together to shape and maintain the endoplasmic reticulum (Wang et al., 2016).
Wang et al. performed CRISPR/Cas9 gene knock outs and stable gene transfections in mammalian cells and also investigated egg extracts from the frog Xenopus, which can form an endoplasmic reticulum network in vitro that is strikingly similar to that seen in intact cells. They found that in addition to being required for the formation of three-way junctions, atlastins are also necessary to maintain such junctions. Wang et al. further report on the interplay among the proteins that are involved in shaping the endoplasmic reticulum. For instance, lunapark is not required for three-way junctions to form, but its depletion appears to cause a loss of tubule junctions and an increase in the number of sheet-like structures.
Another remarkable finding is that the endoplasmic reticulum network fragments if atlastin is inhibited (see also Orso et al., 2009), or if the reticulon proteins are overexpressed. This indicates that the network can spontaneously disassemble in some circumstances and may explain why no proteins specifically involved in the splitting of tubules have ever been identified. Although the endoplasmic reticulum is generally thought to be continuous, previous studies have shown that it can split up in certain situations, for example during the fertilization of starfish eggs or during excessive electrical activity in neurons (Goyal and Blackstone, 2013). A future challenge will be to find out how and why cells might fragment their endoplasmic reticulum.
Finally, Wang et al. propose a compelling mechanism for how lunapark is regulated by phosphorylation during cell division. Modifying lunapark to mimic phosphorylated lunapark caused it to disappear from three-way junctions. This result, coupled with a recent study showing that lunapark is a component of a ubiquitin ligase complex at three-way junctions (Zhao et al., 2016), will probably lead to additional studies into how structural modifications regulate these proteins to control the shape of the endoplasmic reticulum.
We have likely just scratched the surface of how the endoplasmic reticulum is shaped, and additional proteins and regulatory mechanisms will surely be uncovered. Investigating the dynamic interactions of the endoplasmic reticulum with other cell compartments and the plasma membrane seems a particularly exciting area. Furthermore, numerous endoplasmic reticulum shaping proteins are mutated in inherited neurological disorders, particularly the hereditary spastic paraplegias (Blackstone, 2012). Future studies will benefit from emerging new super-resolution microscopy tools, improving our understanding of how the endoplasmic reticulum is dynamically shaped in health and disease.
References
-
Cellular pathways of hereditary spastic paraplegiaAnnual Review of Neuroscience 35:25–47.https://doi.org/10.1146/annurev-neuro-062111-150400
-
Untangling the web: mechanisms underlying ER network formationBiochimica Et Biophysica Acta 1833:2492–2498.https://doi.org/10.1016/j.bbamcr.2013.04.009
-
Structure and function of ER membrane contact sites with other organellesNature Reviews Molecular Cell Biology 17:69–82.https://doi.org/10.1038/nrm.2015.8
-
Form follows function: the importance of endoplasmic reticulum shapeAnnual Review of Biochemistry 84:791–811.https://doi.org/10.1146/annurev-biochem-072711-163501
-
Lunapark is a component of a ubiquitin ligase complex localized to the endoplasmic reticulum three-way junctionsJournal of Biological Chemistry 291:18252–18262.https://doi.org/10.1074/jbc.M116.737783
Article and author information
Author details
Publication history
Copyright
This is an open-access article, free of all copyright, and may be freely reproduced, distributed, transmitted, modified, built upon, or otherwise used by anyone for any lawful purpose. The work is made available under the Creative Commons CC0 public domain dedication.
Metrics
-
- 17,703
- views
-
- 488
- downloads
-
- 7
- citations
Views, downloads and citations are aggregated across all versions of this paper published by eLife.
Download links
Downloads (link to download the article as PDF)
Open citations (links to open the citations from this article in various online reference manager services)
Cite this article (links to download the citations from this article in formats compatible with various reference manager tools)
Further reading
-
- Cell Biology
The steroid hormone progesterone (P4) regulates multiple aspects of reproductive and metabolic physiology. Classical P4 signaling operates through nuclear receptors that regulate transcription. In addition, P4 signals through membrane P4 receptors (mPRs) in a rapid nongenomic modality. Despite the established physiological importance of P4 nongenomic signaling, the details of its signal transduction cascade remain elusive. Here, using Xenopus oocyte maturation as a well-established physiological readout of nongenomic P4 signaling, we identify the lipid hydrolase ABHD2 (α/β hydrolase domain-containing protein 2) as an essential mPRβ co-receptor to trigger meiosis. We show using functional assays coupled to unbiased and targeted cell-based lipidomics that ABHD2 possesses a phospholipase A2 (PLA2) activity that requires mPRβ. This PLA2 activity bifurcates P4 signaling by inducing clathrin-dependent endocytosis of mPRβ, resulting in the production of lipid messengers that are G-protein coupled receptor agonists. Therefore, P4 drives meiosis by inducing an ABHD2 PLA2 activity that requires both mPRβ and ABHD2 as obligate co-receptors.
-
- Cell Biology
- Neuroscience
The claustrum complex is viewed as fundamental for higher-order cognition; however, the circuit organization and function of its neuroanatomical subregions are not well understood. We demonstrated that some of the key roles of the CLA complex can be attributed to the connectivity and function of a small group of neurons in its ventral subregion, the endopiriform (EN). We identified a subpopulation of EN neurons by their projection to the ventral CA1 (ENvCA1-proj. neurons), embedded in recurrent circuits with other EN neurons and the piriform cortex. Although the ENvCA1-proj. neuron activity was biased toward novelty across stimulus categories, their chemogenetic inhibition selectively disrupted the memory-guided but not innate responses of mice to novelty. Based on our functional connectivity analysis, we suggest that ENvCA1-proj. neurons serve as an essential node for recognition memory through recurrent circuits mediating sustained attention to novelty, and through feed-forward inhibition of distal vCA1 neurons shifting memory-guided behavior from familiarity to novelty.