Virology: Pushing the envelope
To counter the constant threat posed by viruses, vertebrate species have evolved a variety of antiviral mechanisms. In return, however, rapid mutation and turnover rates permit viruses to swiftly evolve to evade such mechanisms. This on-going ‘arms race’ between viruses and their hosts has had an important role in shaping the evolution of the species we observe today (tenOever, 2016).
Before a virus infects a cell, envelope proteins displayed on its surface must bind to a receptor located on the surface of the host cell. This receptor-envelope interaction is highly specific and, in turn, determines whether the virus is able to infect a particular cell type or species. In other words, in the absence of the receptor the virus loses its ability to infect the cell.
In a process termed ‘receptor interference’, an envelope protein from a previous viral infection can block cell receptors, preventing infection by a new virus – even an unrelated one – that also binds to that receptor (Figure 1). Remarkably, envelope proteins from such pre-infecting retroviruses can mediate receptor interference even if they have been extinct for millions of years (Boeke and Stoye, 1997). To date, clear examples of such interference have been limited to animal models, including chickens (Payne et al., 1971) and mice (Buller et al., 1987). Now, in eLife, Daniel Blanco-Melo, Robert Gifford and Paul Bieniasz of the Rockefeller University and MRC-University of Glasgow Centre for Virus Research report the first example of such interference in humans (Blanco-Melo et al., 2017).
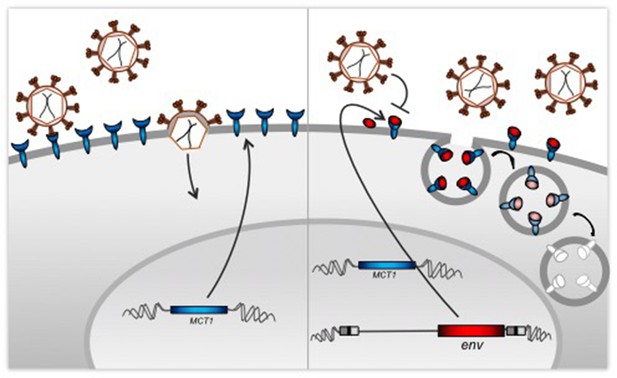
Co-option of a viral protein for receptor interference.
Left: Cells expressing MCT1 (blue), which is the receptor for a retrovirus called HERV-T, are susceptible to infection from a virus that encodes a surface envelope protein produced by an ancestral form of HERV-T (red). Right: Our genome contains proviruses – copies of the DNA of ancient retroviruses, including HERV-T. Blanco-Melo et al. found that human cells can still produce envelope proteins from their copy of the env gene of the HERV-T provirus. These envelope proteins protect the cells from the resurrected virus by blocking the MCT1 receptors directly, or through the degradation of the resulting receptor-protein complex.
When a retrovirus infects a cell it integrates a DNA copy of its own genome (called a provirus) into the host cell’s genome. Because retroviruses occasionally infect germ line cells, the infection may lead to a provirus that is passed to offspring, and that can sometimes become fixed in the population. We refer to such proviruses as ‘endogenous retroviruses’. Over millions of years, large numbers of endogenous retroviruses have accumulated within vertebrate genomes, including humans, thus providing a ‘fossil record’ of previously circulating retroviruses that covers vast evolutionary scales.
While the majority of endogenous retrovirus lineages are ancient and now contain many mutations, recently formed examples tend to more closely resemble their infectious counterparts. This similarity means that, unless it is harmful, an endogenous retrovirus may retain the ability to produce functional RNA and protein products for long periods of time. Indeed, a few such endogenous retroviruses have been ‘co-opted’ to produce RNA or proteins that benefit the host (Ting et al., 1992; Mi et al., 2000; Stoye and Coffin, 2000).
The HERV-T lineage of endogenous retroviruses is an ancient member of a large group of retroviruses called gammaretroviruses (which includes leukemia viruses that affect cats and rodents). As detailed by Blanco-Melo et al., the HERV-T ancestor appears to have first invaded primate germlines about 43 to 32 million years ago, with the last invasion happening around 8 million years ago. Why did this lineage become extinct? Blanco-Melo et al. used an approach known as ‘paleovirology’ (Emerman and Malik, 2010) to begin to investigate this question.
By assessing the distribution of fossilized endogenous retroviruses among modern primate species, Blanco-Melo et al. identified a single HERV-T locus that appeared in the germline approximately 19 to 7 million years ago, whose envelope (env) gene, remarkably, has retained the ability to be translated. As other genes in the same provirus have been inactivated by mutations, this observation strongly suggests that this env gene has been selectively retained. However, its product cannot perform any ‘normal’ retroviral functions. Why, then, should this gene have been so clearly preserved throughout evolution?
Blanco-Melo et al. reconstructed an ancestral HERV-T sequence from the HERV-T proviruses found in the genomes of modern humans and other primates. A variety of cell lines could be infected by reconstructed viruses that encoded the ancestral env product, but those cells that had the version of env that is found in the host were resistant to infection. Blanco-Melo et al. then demonstrated that the product of the host-maintained env gene is able to block infection by viruses that encode the ancestral env gene by depleting the receptor they identified as MCT-1 (monocarboxylate transporter-1) from the surface of the host cell. The results imply that the HERV-T env gene in the host was co-opted and selected for antiviral protection through receptor interference.
The work from Blanco-Melo et al. highlights how endogenous retroviruses can act as raw material for potential use by the host in the host-virus arms race. This result raises the issue of the extent to which the co-option of endogenous retrovirus coding material for antiviral protection is widespread among vertebrates. Could such maintained env genes have contributed to the apparent extinction of other infectious retroviruses in the lineage leading to contemporary humans? We are eager to see whether the HERV-T picture represents a predictable pattern among endogenous retroviruses in other vertebrate lineages.
References
-
RetrovirusesRetrotransposons, Endogenous Retroviruses, and the Evolution of Retroelements, Retroviruses, Cold Spring Harbor, NY, Cold Spring Harbor Laboratory Press.
-
Identification of two forms of an endogenous murine retroviral env gene linked to the rmcf locusJournal of Virology 61:29–34.
-
A dominant epistatic gene which inhibits cellular susceptibility to RSV(RAV-O)Journal of General Virology 13:455–462.https://doi.org/10.1099/0022-1317-13-3-455
-
The evolution of antiviral defense systemsCell Host & Microbe 19:142–149.https://doi.org/10.1016/j.chom.2016.01.006
Article and author information
Author details
Publication history
Copyright
© 2017, Wildschutte et al.
This article is distributed under the terms of the Creative Commons Attribution License, which permits unrestricted use and redistribution provided that the original author and source are credited.
Metrics
-
- 1,428
- views
-
- 183
- downloads
-
- 0
- citations
Views, downloads and citations are aggregated across all versions of this paper published by eLife.
Download links
Downloads (link to download the article as PDF)
Open citations (links to open the citations from this article in various online reference manager services)
Cite this article (links to download the citations from this article in formats compatible with various reference manager tools)
Further reading
-
- Ecology
- Evolutionary Biology
While host phenotypic manipulation by parasites is a widespread phenomenon, whether tumors, which can be likened to parasite entities, can also manipulate their hosts is not known. Theory predicts that this should nevertheless be the case, especially when tumors (neoplasms) are transmissible. We explored this hypothesis in a cnidarian Hydra model system, in which spontaneous tumors can occur in the lab, and lineages in which such neoplastic cells are vertically transmitted (through host budding) have been maintained for over 15 years. Remarkably, the hydras with long-term transmissible tumors show an unexpected increase in the number of their tentacles, allowing for the possibility that these neoplastic cells can manipulate the host. By experimentally transplanting healthy as well as neoplastic tissues derived from both recent and long-term transmissible tumors, we found that only the long-term transmissible tumors were able to trigger the growth of additional tentacles. Also, supernumerary tentacles, by permitting higher foraging efficiency for the host, were associated with an increased budding rate, thereby favoring the vertical transmission of tumors. To our knowledge, this is the first evidence that, like true parasites, transmissible tumors can evolve strategies to manipulate the phenotype of their host.
-
- Evolutionary Biology
- Microbiology and Infectious Disease
Accurate estimation of the effects of mutations on SARS-CoV-2 viral fitness can inform public-health responses such as vaccine development and predicting the impact of a new variant; it can also illuminate biological mechanisms including those underlying the emergence of variants of concern. Recently, Lan et al. reported a model of SARS-CoV-2 secondary structure and its underlying dimethyl sulfate reactivity data (Lan et al., 2022). I investigated whether base reactivities and secondary structure models derived from them can explain some variability in the frequency of observing different nucleotide substitutions across millions of patient sequences in the SARS-CoV-2 phylogenetic tree. Nucleotide basepairing was compared to the estimated ‘mutational fitness’ of substitutions, a measurement of the difference between a substitution’s observed and expected frequency that is correlated with other estimates of viral fitness (Bloom and Neher, 2023). This comparison revealed that secondary structure is often predictive of substitution frequency, with significant decreases in substitution frequencies at basepaired positions. Focusing on the mutational fitness of C→U, the most common type of substitution, I describe C→U substitutions at basepaired positions that characterize major SARS-CoV-2 variants; such mutations may have a greater impact on fitness than appreciated when considering substitution frequency alone.