Development: Transforming a transcription factor
As an embryo develops, complex regulatory networks control where and when genes are activated, resulting in tissues and organs forming at the right time and place. Changes to these networks, in particular to DNA sequences that bind transcription factors, can affect how an organism develops and looks (Carroll, 2008; Wray, 2007; Peter and Davidson, 2011).
Transcription factors are proteins that help turn specific genes on or off by binding to nearby DNA, and a somewhat controversial theory suggests that changes affecting the strength of transcription factor binding may modify regulatory networks (Lynch and Wagner, 2008). Such changes are, however, less favored, as they could affect many genes and thus have a negative impact on the fitness of an organism. Now, in eLife, Jian Ming Khor and Charles Ettensohn of Carnegie Mellon University report how a specific region on a transcription factor can indeed affect skeleton formation in sea urchins (Khor and Ettensohn, 2017).
Sea urchins are a popular model organism in developmental biology and like most other echinoderms, the larvae of sea urchins are very different to their adult form. Sea urchins develop from an egg into a planktonic larva, before transforming into a bottom-dwelling juvenile, and unlike most echinoderms, they form a larval skeleton in the early embryonic stages (McClay, 2011).
A well-studied group of cells called the micromeres are key to this process, and are the first cells internalized as the embryo acquires its form (McIntyre et al., 2014). The molecular mechanisms that determine their fate have thus received considerable attention and were one of the first examples of a developmental gene regulatory network (Davidson et al., 2002; Ettensohn, 2009). Some of the molecular components of this network are located in the unfertilized egg and are divided unequally between new cells. Within the micromeres, regulatory genes control a hierarchical network of genes, which causes them to build a precisely positioned and patterned skeleton (Figure 1A–D).
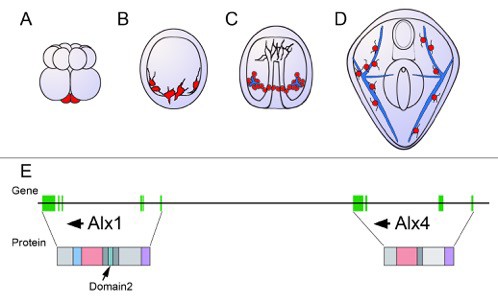
Schematic showing the development of the sea urchin from embryo to larva.
(A) During the 16-cell stage, a cluster of four small cells called the micromeres (red) form at one pole of the embryo. (B) The micromeres then develop into primary mesenchyme cells (also shown in red) and migrate into the embryo. (C) As the embryo turns into a hollow ball of cells, the primary mesenchyme assembles into a ring with two clusters of cells (red), positioned ventrally. During this phase, the cells fuse and begin to secrete a calcium carbonate skeleton (shown in blue). (D) In the larva, the skeleton has developed into a pair of skeletal rods (blue) that grow to support and shape the larva. (E) The genes Alx1 and Alx4 are adjacent and are thought to have arisen from a duplication early during the evolution of echinoderms. Arrows indicate the orientation of the genes. The genes are similar, but differ in the regions of the gene that encode the protein sequence (vertical green bars). The proteins also have several identical domains, but Domain 2 (shown in turquoise), which is critical to skeleton formation, is only found in Alx1. Data for the gene organization of the purple sea urchin Strongylocentrotus purpuratus (as displayed here) has been obtained from the echinoderm genomic database ‘Echinobase’.
One key transcription factor in this regulatory network is Alx1, which is exclusively activated or expressed in the micromeres soon after they form (Ettensohn et al., 2003). Previous research has shown that when Alx1 is blocked, the embryos of sea urchins develop without forming a skeleton, but when Alx1 is overexpressed in cells other than the micromeres, they develop into skeleton-forming cells.
Khor and Ettensohn blocked Alx1 with a compound called a morpholino, and at the same time, injected the embryo with a version of Alx1 that is insensitive to this substance and restores skeleton formation. To identify the roles of the Alx1 protein, they deleted or mutated various parts of the morpholino-insensitive Alx1 and tested if the embryos were still able to build a skeleton. Most parts of the protein were dispensable, but a small domain unique to Alx1, called Domain 2, turned out to be essential for skeleton formation. Furthermore, Khor and Ettensohn discovered that when Domain 2 was inserted into Alx4, which is an adjacent copy of Alx1, sea urchins were able to form a normal skeleton.
Khor and Ettensohn then compared the genomes of other echinoderms and discovered that Alx4 was highly conserved within all members of this group, whereas Alx1 varied greatly. The Alx1 proteins of close relatives of the sea urchin were functionally interchangeable, while the Alx1 proteins of more distantly related echinoderms were not. Khor and Ettensohn suggest that this is due to differences in the regions outside Domain 2. Alx1 and Alx4 are thought to be the result of an ancient gene duplication, and the acquisition of Domain 2 may have determined their different roles (Figure 1E).
This study is a sterling example of a transcription factor altering its protein sequence and presumably its affinities, leading to the functional differences of Alx1 and Alx4. Khor and Ettensohn emphasize that to fully understand these evolutionary changes more research is needed to clarify how Alx4 contributes to the formation of the skeleton in adults. We also need to examine the protein structure of Alx1 more deeply to discover what Domain 2 interacts with, and how it initiates skeleton formation.
The transcription factor Alx has the potential to become an informative model for transcription factor evolution because of its detailed gene regulatory network and the comparisons that can be made between species that diverged at different times. This will deepen our knowledge of how mechanisms beyond mutations in DNA sequences have shaped the evolution of gene regulatory networks.
References
-
A genomic regulatory network for developmentScience 295:1669–1678.https://doi.org/10.1126/science.1069883
-
Evolutionary crossroads in developmental biology: sea urchinsDevelopment 138:2639–2648.https://doi.org/10.1242/dev.048967
-
The evolutionary significance of cis-regulatory mutationsNature Reviews Genetics 8:206–216.https://doi.org/10.1038/nrg2063
Article and author information
Author details
Publication history
Copyright
© 2018, Burke
This article is distributed under the terms of the Creative Commons Attribution License, which permits unrestricted use and redistribution provided that the original author and source are credited.
Metrics
-
- 1,549
- views
-
- 123
- downloads
-
- 1
- citations
Views, downloads and citations are aggregated across all versions of this paper published by eLife.
Download links
Downloads (link to download the article as PDF)
Open citations (links to open the citations from this article in various online reference manager services)
Cite this article (links to download the citations from this article in formats compatible with various reference manager tools)
Further reading
-
- Developmental Biology
- Genetics and Genomics
Paternal obesity has been implicated in adult-onset metabolic disease in offspring. However, the molecular mechanisms driving these paternal effects and the developmental processes involved remain poorly understood. One underexplored possibility is the role of paternally induced effects on placenta development and function. To address this, we investigated paternal high-fat diet-induced obesity in relation to sperm histone H3 lysine 4 tri-methylation signatures, the placenta transcriptome, and cellular composition. C57BL6/J male mice were fed either a control or high-fat diet for 10 weeks beginning at 6 weeks of age. Males were timed-mated with control-fed C57BL6/J females to generate pregnancies, followed by collection of sperm, and placentas at embryonic day (E)14.5. Chromatin immunoprecipitation targeting histone H3 lysine 4 tri-methylation (H3K4me3) followed by sequencing (ChIP-seq) was performed on sperm to define obesity-associated changes in enrichment. Paternal obesity corresponded with altered sperm H3K4me3 at promoters of genes involved in metabolism and development. Notably, altered sperm H3K4me3 was also localized at placental enhancers. Bulk RNA-sequencing on placentas revealed paternal obesity-associated sex-specific changes in expression of genes involved in hypoxic processes such as angiogenesis, nutrient transport, and imprinted genes, with a subset of de-regulated genes showing changes in H3K4me3 in sperm at corresponding promoters. Paternal obesity was also linked to impaired placenta development; specifically, a deconvolution analysis revealed altered trophoblast cell lineage specification. These findings implicate paternal obesity effects on placenta development and function as one potential developmental route to offspring metabolic disease.
-
- Developmental Biology
Although the impact of gender-affirming hormone therapy (GAHT) on spermatogenesis in trans women has already been studied, data on its precise effects on the testicular environment is poor. Therefore, this study aimed to characterize, through histological and transcriptomic analysis, the spermatogonial stem cell niche of 106 trans women who underwent standardized GAHT, comprising estrogens and cyproterone acetate. A partial dedifferentiation of Sertoli cells was observed, marked by the co-expression of androgen receptor and anti-Müllerian hormone which mirrors the situation in peripubertal boys. The Leydig cells also exhibited a distribution analogous to peripubertal tissue, accompanied by a reduced insulin-like factor 3 expression. Although most peritubular myoid cells expressed alpha-smooth muscle actin 2, the expression pattern was disturbed. Besides this, fibrosis was particularly evident in the tubular wall and the lumen was collapsing in most participants. A spermatogenic arrest was also observed in all participants. The transcriptomic profile of transgender tissue confirmed a loss of mature characteristics - a partial rejuvenation - of the spermatogonial stem cell niche and, in addition, detected inflammation processes occurring in the samples. The present study shows that GAHT changes the spermatogonial stem cell niche by partially rejuvenating the somatic cells and inducing fibrotic processes. These findings are important to further understand how estrogens and testosterone suppression affect the testis environment, and in the case of orchidectomized testes as medical waste material, their potential use in research.