Immunodeficiency: Back from the brink of obscurity
Throughout history, human health has been perpetually challenged by pestilence. In the late 19th century, 'germ theory' established the role of specific microbes in infectious diseases. However, it later became apparent that only a few of the individuals who are exposed to a microbe go on to develop a disease, and even fewer die. Why does this happen? Now, in eLife, Jean-Laurent Casanova and colleagues – including Antoine Guérin as first author – report one answer to this question for an obscure infection known as Whipple's disease (Guérin et al., 2018).
In 1907, George Whipple described a fatal disease that was marked by pain spreading through the joints, chronic diarrhea, excessive fat in the feces, and weight loss (Whipple, 1907). Under the microscope, he saw fatty material accumulate in the intestine and surrounding lymph nodes (Figure 1); he also noted abnormal 'foamy' cells with an associated rod-shaped microbe. Subsequent reports highlighted that this disease spread systemically through the body, affecting various organs.
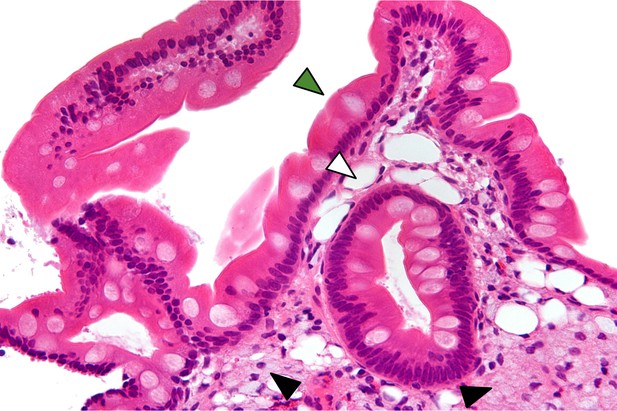
Biopsy of the small intestine showing features classic for Whipple’s disease.
The epithelial cells that line the gut are smooth because the microvilli normally found on them have been blunted (green arrowhead). The area beneath the epithelial cells, the lamina propria, is filled with numerous round cells with a 'foamy' appearance: these are the macrophages (black arrowheads). Extracellular fat has also accumulated in this area (white arrowhead). The tissue is stained with hematoxylin and eosin stain.
IMAGE CREDIT: Micrograph by Nephron (CC BY-SA 3.0).
The bacterium was fastidious and could not be grown in the laboratory. In 1949, however, a new staining technique allowed the foamy cells, which are actually macrophages, to be recognized in affected tissues, thus enabling diagnosis of the disease (Black-Schaffer, 1949). Later, in 1961, electron microscopy identified the distinctive three layers of 'Whipple’s bacillus' (Yardley and Hendrix, 1961), and in 1992 genetic sequencing revealed it to be a previously unknown organism, which was designated Tropheryma whippelii and later renamed Tropheryma whipplei (Relman et al., 1992; La Scola et al., 2001). During this time it was also shown that antibiotics could transform Whipple’s disease into a treatable condition.
As with any disease, doctors seek to better understand the condition in order to improve diagnosis and treatment. PCR-based methods demonstrated that the organism was ubiquitous; for example, it was found in sewage water, human saliva and feces from people unaffected by Whipple’s disease (Maiwald et al., 1998; Street et al., 1999). Blood tests also revealed that human exposure was common (Raoult et al., 2000). So, why was the incidence of disease so low? Since T. whipplei could not be cultured conventionally, researchers could not develop experimental infection models to understand how the disease develops and what makes hosts susceptible. Thus, most researchers studied naturally occurring infections in humans, and made observations that were consistent with Whipple’s original description, including dysfunctional macrophages in affected patients.
Meanwhile, astute clinical observation opened an alternate line of investigations. In 1955, a familial case of Whipple’s disease was reported, involving a mother and only two of her five adult children. This implied a hereditary component of susceptibility (Puite and Tesluk, 1955), and while no further genetic studies were reported from that family, several similar cases were described in other families (Gross et al., 1959). These discoveries implied that genetic approaches could circumvent the microbe’s fastidiousness and finally provide insight into this obscure disease.
Guérin et al. – who are based in institutions in France, Qatar, the United States, and Australia – evaluated 26 members of a French family: four people had Whipple’s disease; five were carriers of the bacterium but did not have the disease; 13 were healthy non-carriers; and four were healthy individuals whose carrier status was unknown. Elegant genomic studies and bioinformatics analyses identified a new variant of IRF4 (the gene for an immune transcription factor) in all those with the disease; in all the carriers; in two of the healthy non-carriers; and in one whose carrier status was unknown. The new variant was very rare and did not appear in any genomics database. Each individual carried one copy of the rare variant alongside a more typical version of the gene (i.e. they were all heterozygous).
Molecular investigations revealed that the rare variant differed by one amino acid (a tryptophan in place of an arginine). This change compromised the protein’s ability to bind to DNA and activate transcription. However, the mutant version of the protein did not interfere with the normal version, meaning it did not exert a negative dominance effect. Instead, Guérin et al. presumed the susceptibility occurs because people must need working protein from both versions of this gene to be protected against the bacteria. This phenomenon is referred to as haploinsufficiency.
In experiments that capitalized on the unique expertise of Didier Raoult's lab in culturing T. whipplei in vitro, the loss-of-function in IRF4 was linked to a distinct gene expression profile in response to the bacterium. Similar results were seen for a related bacterium, Mycobacterium bovis strain BCG. The genes that were expressed differently in response to T. whipplei could not be organized into a defined pathway, but those that differed in the response to BCG highlighted the role of IRF4 in regulating macrophages in cell-mediated immunity.
Together these findings raise a number of exciting questions. First, which IRF4-expressing cell types mitigate the disease? What molecular pathway connects IRF4 to the recognition and effective control or eradication of T. whipplei? What is the basis for the macrophages and lipid accumulation phenotypes that characterize this disease? And are mutations in IRF4 or other molecules at play in other patients with Whipple’s disease? These and other questions can now be pursued, guided by the study of this single yet informative family. On a grander scale, this work also reinforces the value of studying humans, including those with rare inborn errors of immunity, to understand human immunobiology, using approaches that cross disciplines.
References
-
The tinctoral demonstration of a glycoprotein in Whipple's diseaseExperimental Biology and Medicine 72:225–227.https://doi.org/10.3181/00379727-72-17388
-
Whipple's disease; report of four cases, including two in brothers, with observations on pathologic physiology, diagnosis, and treatmentGastroenterology 36:65–93.
-
Description of Tropheryma whipplei gen. nov., sp. nov., the Whipple's disease bacillusInternational Journal of Systematic and Evolutionary Microbiology 51:1471–1479.https://doi.org/10.1099/00207713-51-4-1471
-
Environmental occurrence of the Whipple's disease bacterium (Tropheryma whippelii)Applied and Environmental Microbiology 64:760–762.
-
Whipple's diseaseThe American Journal of Medicine 19:383–400.https://doi.org/10.1016/0002-9343(55)90127-3
-
Cultivation of the bacillus of Whipple's diseaseNew England Journal of Medicine 342:620–625.https://doi.org/10.1056/NEJM200003023420903
-
Identification of the uncultured bacillus of Whipple's diseaseNew England Journal of Medicine 327:293–301.https://doi.org/10.1056/NEJM199207303270501
-
A hitherto undescribed disease characterized anatomically by deposits of fat and fatty acids in the intestinal and mesenteric lymphatic tissuesBulletin of the Johns Hopkins Hospital 18:382–393.
-
Combined electron and light microscopy in Whipple's disease. Demonstration of "bacillary bodies" in the intestineBulletin of the Johns Hopkins Hospital 109:80–98.
Article and author information
Author details
Publication history
Copyright
© 2018, Vinh
This article is distributed under the terms of the Creative Commons Attribution License, which permits unrestricted use and redistribution provided that the original author and source are credited.
Metrics
-
- 1,071
- views
-
- 63
- downloads
-
- 1
- citations
Views, downloads and citations are aggregated across all versions of this paper published by eLife.
Download links
Downloads (link to download the article as PDF)
Open citations (links to open the citations from this article in various online reference manager services)
Cite this article (links to download the citations from this article in formats compatible with various reference manager tools)
Further reading
-
- Cell Biology
- Immunology and Inflammation
The endothelial blood-brain barrier (BBB) strictly controls immune cell trafficking into the central nervous system (CNS). In neuroinflammatory diseases such as multiple sclerosis, this tight control is, however, disturbed, leading to immune cell infiltration into the CNS. The development of in vitro models of the BBB combined with microfluidic devices has advanced our understanding of the cellular and molecular mechanisms mediating the multistep T-cell extravasation across the BBB. A major bottleneck of these in vitro studies is the absence of a robust and automated pipeline suitable for analyzing and quantifying the sequential interaction steps of different immune cell subsets with the BBB under physiological flow in vitro. Here, we present the under-flow migration tracker (UFMTrack) framework for studying immune cell interactions with endothelial monolayers under physiological flow. We then showcase a pipeline built based on it to study the entire multistep extravasation cascade of immune cells across brain microvascular endothelial cells under physiological flow in vitro. UFMTrack achieves 90% track reconstruction efficiency and allows for scaling due to the reduction of the analysis cost and by eliminating experimenter bias. This allowed for an in-depth analysis of all behavioral regimes involved in the multistep immune cell extravasation cascade. The study summarizes how UFMTrack can be employed to delineate the interactions of CD4+ and CD8+ T cells with the BBB under physiological flow. We also demonstrate its applicability to the other BBB models, showcasing broader applicability of the developed framework to a range of immune cell-endothelial monolayer interaction studies. The UFMTrack framework along with the generated datasets is publicly available in the corresponding repositories.
-
- Immunology and Inflammation
The gut biome, a complex ecosystem of micro- and macro-organisms, plays a crucial role in human health. A disruption in this evolutive balance, particularly during early life, can lead to immune dysregulation and inflammatory disorders. ‘Biome repletion’ has emerged as a potential therapeutic approach, introducing live microbes or helminth-derived products to restore immune balance. While helminth therapy has shown some promise, significant challenges remain in optimizing clinical trials. Factors such as patient genetics, disease status, helminth species, and the optimal timing and dosage of their products or metabolites must be carefully considered to train the immune system effectively. We aim to discuss how helminths and their products induce trained immunity as prospective to treat inflammatory and autoimmune diseases. The molecular repertoire of helminth excretory/secretory products (ESPs), which includes proteins, peptides, lipids, and RNA-carrying extracellular vesicles (EVs), underscores their potential to modulate innate immune cells and hematopoietic stem cell precursors. Mimicking natural delivery mechanisms like synthetic exosomes could revolutionize EV-based therapies and optimizing production and delivery of ESP will be crucial for their translation into clinical applications. By deciphering and harnessing helminth-derived products’ diverse modes of action, we can unleash their full therapeutic potential and pave the way for innovative treatments.