Bone-Targeting Drugs: From vesicle to cytosol
Despite its appearance, bone is a highly metabolic and dynamic tissue that is composed of a vast network of cells called osteocytes that are embedded in a matrix made mostly of collagen and various salts of calcium and phosphate. These osteocytes sense regions of damaged or weakened bone, and 'instruct' bone-destroying cells (called osteoclasts) and bone-forming cells (osteoblasts) to, respectively, remove old bone and deposit new bone (Figure 1). Hence, like a team of road-repairers, the osteocytes, osteoclasts and osteoblasts work together to repair bone and maintain our skeleton in good health (Crockett et al., 2011).
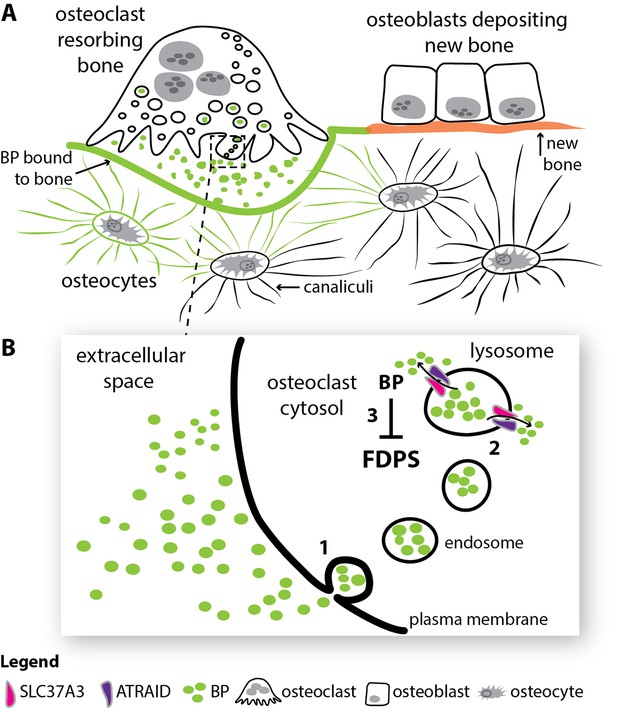
How bisphosphonates act in bone.
(A) Old and damaged bone is constantly being broken down by cells called osteoclasts in a process called resorption (top left), while new bone is deposited by cells called osteoblasts (top right). Cells called osteocytes (bottom) influence both of these processes through a spidery system of tiny canals called canaliculi. After entering the circulation, the drug bisphosphonate (green) binds very effectively to calcium ions on the bone mineral surface. During resorption, the bisphosphonate on the bone surface is released into the acidic extracellular space beneath the osteoclast. (B) The bisphosphonate in the extracellular space is engulfed into osteoclasts via a process called endocytosis (1). The resulting endosomes mature to form structures called lysosomes, and two proteins, SLC37A3 and ATRAID, then interact in the membrane of the lysosome to allow the bisphosphonate to enter the cytosol (2). Once in the cytosol, the nitrogen-containing bisphosphonates inhibit an enzyme called FDPS and prevent the osteoclast from breaking down bone (3). BP: bisphosphonate; FDPS: farnesyl diphosphate synthase; SLC37A3: solute carrier family 37 member A3.
In young adult life there is usually a balance between the amount of old bone broken down and the amount of new bone formed by this repair process, so there is no net gain or loss of bone mass. However, in diseases that affect the skeleton, such as post-menopausal osteoporosis or cancers growing in bone, this delicate balance can be disturbed by osteoclasts being over-active, which leads to excessive bone destruction and fractures. Drugs called bisphosphonates – which inhibit osteoclasts – have been used for more than three decades to treat such diseases and protect the skeleton from potentially catastrophic bone loss, although researchers still do not fully understand how they work. Now, in eLife, Erin O'Shea of Harvard University and the Howard Hughes Medical Institute (HHMI) and colleagues – including Zhou Yu as first author – report the answer to one of the remaining questions about these drugs (Yu et al., 2018).
Bisphosphonates are synthetic molecules that closely resemble the chemical structure of pyrophosphate, which is a natural by-product of numerous metabolic reactions. Importantly, bisphosphonates have two negatively-charged phosphonate groups that enable them to bind calcium ions very effectively, and hence to localize rapidly to any exposed calcium on the bone surface (Rogers et al., 2011). The mechanisms used by bisphosphonates to inhibit osteoclasts remained a mystery for several decades after they were first used in the clinic, but this did not stop the development of improved versions of the drugs (Russell et al., 2008). Eventually it was discovered that nitrogen-containing bisphosphonates (N-BPs), which are now widely used to treat osteoporosis and other bone diseases, work by inhibiting an enzyme called FDPS inside the osteoclasts (Luckman et al., 1998; Bergstrom et al., 2000; Dunford et al., 2001). The N-BP molecules displace the lipid substrates that the FDPS enzyme usually acts on, locking the enzyme in an inactive state (Kavanagh et al., 2006; Rondeau et al., 2006). Without FDPS activity, osteoclasts are no longer able to degrade bone (Rogers et al., 2011).
However, one question remained: how do the N-BPs and other bisphosphonates actually reach the FDPS enzyme, which is in the cytosol of the osteoclasts? There was little or no evidence that a receptor on the plasma membrane was involved (Thompson et al., 2006). Studies with fluorescently-tagged bisphosphonates showed that they first entered the osteoclasts via endocytosis – a process that involves the cell membrane folding inwards and then pinching off to create a vesicle inside the cell (Coxon et al., 2008). But how do the drugs leave these vesicles – which are enclosed by a membrane – to enter the cytosol? Bisphosphonate molecules have a large negative charge, which rules out passive diffusion across the vesicle membrane, which in turn suggests the possibility of a hitherto unidentified transport mechanism (Thompson et al., 2006).
Yu et al. – who are based at Harvard, HHMI, UCSF, MIT, the Broad, Koch and Whitehead Institutes, and Washington University – report that they have identified a protein called SLC37A3 that is required for the release of N-BP molecules from vesicles into the cytosol. Using a CRISPR-based approach to screen for genes that, when missing, confer resistance to bisphosphonates, they identified SLC37A3 as the gene with the strongest effect. Although the exact function of the SLC37A3 protein remains to be clarified, related members of this protein family are involved in the transport of charged molecules across membranes (Cappello et al., 2018).
Yu et al. found that SLC37A3 interacts and co-localizes with a protein called ATRAID at the vesicle membrane (Figure 1). Importantly, vesicles isolated from cells that did not express SLC37A3 or ATRAID appeared unable to release N-BP molecules, and these cells were much less sensitive to the pharmacological effect of N-BPs.
This new transport mechanism identified by Yu et al. raises interesting questions about how the SLC37A3/ATRAID complex specifically recognizes N-BP molecules, and how it transports them across the membrane of the vesicle. It will also be worthwhile to determine whether differences in the expression of SLC37A3 or ATRAID account for the different sensitivity of osteoclasts and other cell types to N-BP molecules, or whether variants in these genes affect the clinical responsiveness of patients to these drugs. Nevertheless, these elegant studies explain how negatively-charged N-BP molecules can gain access to the cell cytosol after endocytosis and, as a result, go on to benefit huge numbers of patients with potentially devastating bone diseases.
References
-
Alendronate is a specific, nanomolar inhibitor of farnesyl diphosphate synthaseArchives of Biochemistry and Biophysics 373:231–241.https://doi.org/10.1006/abbi.1999.1502
-
Bone remodelling at a glanceJournal of Cell Science 124:991–998.https://doi.org/10.1242/jcs.063032
-
Structure-activity relationships for inhibition of farnesyl diphosphate synthase in vitro and inhibition of bone resorption in vivo by nitrogen-containing bisphosphonatesJournal of Pharmacology and Experimental Therapeutics 296:235–242.
Article and author information
Author details
Publication history
Copyright
© 2018, Rogers et al.
This article is distributed under the terms of the Creative Commons Attribution License, which permits unrestricted use and redistribution provided that the original author and source are credited.
Metrics
-
- 1,230
- views
-
- 136
- downloads
-
- 6
- citations
Views, downloads and citations are aggregated across all versions of this paper published by eLife.
Download links
Downloads (link to download the article as PDF)
Open citations (links to open the citations from this article in various online reference manager services)
Cite this article (links to download the citations from this article in formats compatible with various reference manager tools)
Further reading
-
- Biochemistry and Chemical Biology
The protein ligase Connectase can be used to fuse proteins to small molecules, solid carriers, or other proteins. Compared to other protein ligases, it offers greater substrate specificity, higher catalytic efficiency, and catalyzes no side reactions. However, its reaction is reversible, resulting in only 50% fusion product from two equally abundant educts. Here, we present a simple method to reliably obtain 100% fusion product in 1:1 conjugation reactions. This method is efficient for protein-protein or protein-peptide fusions at the N- or C-termini. It enables the generation of defined and completely labeled antibody conjugates with one fusion partner on each chain. The reaction requires short incubation times with small amounts of enzyme and is effective even at low substrate concentrations and at low temperatures. With these characteristics, it presents a valuable new tool for bioengineering.
-
- Biochemistry and Chemical Biology
- Microbiology and Infectious Disease
Glutamine synthetases (GS) are central enzymes essential for the nitrogen metabolism across all domains of life. Consequently, they have been extensively studied for more than half a century. Based on the ATP-dependent ammonium assimilation generating glutamine, GS expression and activity are strictly regulated in all organisms. In the methanogenic archaeon Methanosarcina mazei, it has been shown that the metabolite 2-oxoglutarate (2-OG) directly induces the GS activity. Besides, modulation of the activity by interaction with small proteins (GlnK1 and sP26) has been reported. Here, we show that the strong activation of M. mazei GS (GlnA1) by 2-OG is based on the 2-OG dependent dodecamer assembly of GlnA1 by using mass photometry (MP) and single particle cryo-electron microscopy (cryo-EM) analysis of purified strep-tagged GlnA1. The dodecamer assembly from dimers occurred without any detectable intermediate oligomeric state and was not affected in the presence of GlnK1. The 2.39 Å cryo-EM structure of the dodecameric complex in the presence of 12.5 mM 2-OG demonstrated that 2-OG is binding between two monomers. Thereby, 2-OG appears to induce the dodecameric assembly in a cooperative way. Furthermore, the active site is primed by an allosteric interaction cascade caused by 2-OG-binding towards an adaption of an open active state conformation. In the presence of additional glutamine, strong feedback inhibition of GS activity was observed. Since glutamine dependent disassembly of the dodecamer was excluded by MP, feedback inhibition most likely relies on the binding of glutamine to the catalytic site. Based on our findings, we propose that under nitrogen limitation the induction of M. mazei GS into a catalytically active dodecamer is not affected by GlnK1 and crucially depends on the presence of 2-OG.