Chemotaxis: Cider vinegar rules
Running through an unfamiliar area, the familiar odor of fast food wafts into your nostrils and you turn your head to see where it is coming from – even though you know that fast food is not good for your health. Flies respond in a similar way when they smell apple cider vinegar. But how are humans or flies able to locate the source of a scent or odor? The answer lies in a process called chemotaxis – the technical term for orienting to a scent or odor and pursuing or avoiding it.
Chemotaxis has been studied for decades. In The Orientation of Animals, first published in 1940, Gottfried Fraenkel and Donald Gunn defined many of the concepts and terms that are central to chemotaxis (Fraenkel and Gunn, 1961). In particular, they recognized that chemicals – unlike other stimuli such as gravity and light – have no inherent directional component and thus require different strategies for taxis.
In relatively still water or air, chemical gradients are diffusion limited and relatively stable: thus, by sampling the chemical over time, the organism can determine the structure of a chemical gradient. When confronted with such a gradient some species, such as E. coli (Berg, 2004) and C. elegans (Lockery, 2011), move in a straight line and then randomly change direction when the chemical cue becomes weaker.
Drosophila larvae, on the other hand, are more sophisticated and preferentially turn toward higher concentrations of the chemical cue. The ability of Drosophila larvae to detect very small decreases in the concentration of chemical cues, combined with the widespread availability of tools for the genetic manipulation of flies, has resulted in this species becoming an important model system for the study of chemotaxis (Gepner et al., 2015; Hernandez-Nunez et al., 2015; Schulze et al., 2015; Calabrese, 2015).
However, the air is not still in many environments, with air currents disrupting chemical gradients and odor plumes. Close to the ground or another surface, the air flow may be laminar (that is, not turbulent), and the structure of odor plumes is relatively stable. Under these conditions, the air flow provides directional information that helps flies find the source of the odor. For a flying insect, however, locating the source of an odor means navigating a turbulent odor plume where changes in odor concentration can be rapid and do not directly tell the insect if it is moving toward or away from a source.
The ability of moths to follow the odor plume of a sex pheromone has been extensively studied (see, for example, Baker, 1990). The moths fly upwind in the presence of the odor until they lose the scent, and then start flying across the wind; once the odor is encountered again, they resume flying upwind. Adult flying Drosophila employ a similar strategy, with the wind providing a directional cue that is not present in the temporal structure of the chemical signal (Bhandawat et al., 2010). Now, in eLife, Katherine Nagel of New York University (NYU) and co-workers – including Efrén Álvarez-Salvado as first author – shed new light on the rules that govern chemotaxis in flies walking in laminar flow and also explore what happens in more turbulent flow conditions.
Key to their effort was the development of small rectangular wind tunnels in which a constant laminar flow can be maintained, in which the flies can be constrained to walk in one plane, and in which the concentration of odor pulses delivered from a point source at the upwind end of a tunnel can be monitored at multiple points along the tunnel. With multiple tunnels in parallel, Álvarez-Salvado et al. – who are based at NYU, Weill Cornell Medical College and the University of Colorado Boulder – were able to monitor large numbers of flies under similar experimental conditions. They mostly used genetically blinded flies to limit the influence of visual cues, but sighted flies showed similar (though sometimes weaker) responses.
In trials with no odors, the flies tended to spend most of their time in the downwind end of the tunnel. However, when they first detected an odor pulse they usually responded by rapidly turning upwind, increasing their upwind velocity and decreasing the probability of turning: this is called the ON response. And after the odor pulse had passed, the flies decreased their upwind velocity and increased their turn probability, which resulted in a meandering local search for the source of the odor (Figure 1): this was the OFF response.
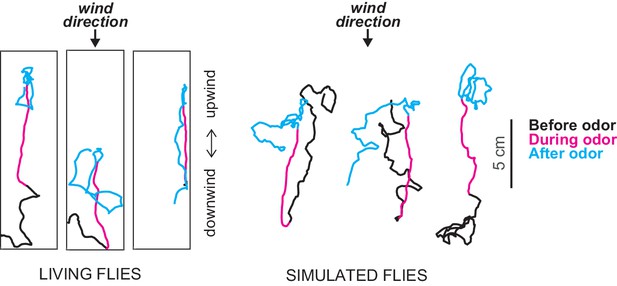
Exploring chemotaxis in flies in the presence of air currents.
Álvarez-Salvado et al. performed experiments in which they tracked the movement of flies in small-scale wind tunnels when pulses of an odor (apple cider vinegar) were released from a point source (not shown) and were carried by the wind through the tunnel: in the figure the wind is blowing from top to bottom. Left: tracks of three genetically blinded flies before (black), during (magenta) and after (cyan) a 10 second odor pulse. At the onset of the pulse the flies turn rapidly toward the source of the odor and increase their upwind velocity. When the pulse ends the flies start to change direction in an effort to locate the odor. Right: based on these findings Álvarez-Salvado et al. built a model to simulate the movement of flies in the wind tunnels. The model does a good job of capturing the responses of the flies to various odor pulses.
Álvarez-Salvado et al. then showed that both the ON response and the OFF response depended on the odor concentration in a sigmoidal manner: that is, each response started to change at a particular concentration, continued to change as the concentration increased, and then stopped changing when the concentration reached a saturation value. The researchers were also able to make flies 'blind' to the wind by applying glue to their antennae: these files walked in all directions – not just upwind – in response to odor pulses.
The researchers concluded that, under their experimental conditions, the ON response is multi-modal, with changes in speed and turn probability depending on the odor concentration, and changes in orientation depending on wind direction (as detected by the mechanoreceptors on the antennae of the flies). The OFF response, on the other hand, is elicited by decreasing odor concentration.
These conclusions were corroborated and extended in experiments with more complicated odor pulses (including, for example, pulses in which the odor concentration fluctuated at different frequencies). A number of findings emerged from these experiments. First, the researchers observed a sensitivity adaptation that allows the fly to respond to a small decrease from a saturating concentration of odor much like Drosophila larvae in a stable odor gradient. Second, it appeared that the ON and OFF responses can be driven at the same time. Third, the response of the flies to fluctuations in the odor concentration depended on the frequency of the fluctuations. Fourth, the ON and OFF responses depended on the history of the odor in different ways, with rapid fluctuations (or a prolonged odor) leading to sustained ON responses and suppressed OFF responses.
Álvarez-Salvado et al. then tested these ideas in three interesting ways. First, they constructed simple models that embody these rules and used them to simulate how a fly in a wind tunnel responded to various odor pulses. The model produced realistic responses for both normal blind flies (Figure 1) and wind-blind flies. Second, they tested this model further by taking advantage of behavioral variability (that is, the fact that all flies will have slightly different responses to the same odor pulses). By scaling the ON and OFF response functions to individual flies, they were able to capture their individual responses and generate individually realistic tracks.
Third, Álvarez-Salvado et al. performed experiments in bigger wind tunnels in which the air flow was turbulent rather than laminar; the flies were also constrained to walk on the bottom surface of the wind tunnel. In the absence of odor, flies tended to move to the downwind end of the tunnel. When apple cider vinegar was introduced into the air stream, the flies responded by moving upwind, and performed local searches if they left the odor plume. While this behavior was similar to that seen in laminar flows in the small wind tunnels, the flies were less successful in locating the source of the odor. Simulated flies showed similar tracks and success rates.
As a result of these studies, we are beginning to understand how terrestrial navigation can be guided by air-borne odors. Although a chemical odor is an inherently non-directional cue, it acquires a directional component that can be used for navigation if the direction of air flow can be determined. Moreover, these studies show that the process of navigation itself can be broken down into component responses. In the case of the walking fly, the ON response (which depends on both odor and air-flow detection) turns the animal into the wind and keeps it on a relatively straight track, whereas the OFF response (which depends on odor detection only) leads to a local search to relocate the odor. These simple rules can also guide navigation under more natural or turbulent conditions (as in larger wind tunnels).
The importance of having done these experiments in Drosophila is that powerful genetic, optogenetic and electrophysiological tools can now be deployed to explore the neuronal mechanisms underlying the ON and OFF responses and their integration with the motor system that governs locomotion.
Keeping these cider vinegar rules in mind might also prove helpful when you next encounter the odor of fast food: just turn crosswind and head straight to carry yourself away from temptation.
References
-
ConferenceUpwind flight and casting flight: Complementary phasic and tonic systems used for location of sex pheromone sources by male mothProceedings of the 10th International Symposium on Olfaction and Taste (Oslo). pp. 18–25.
-
Olfactory modulation of flight in Drosophila is sensitive, selective and rapidJournal of Experimental Biology 213:3625–3635.https://doi.org/10.1242/jeb.040402
-
The computational worm: spatial orientation and its neuronal basis in C. elegansCurrent Opinion in Neurobiology 21:782–790.https://doi.org/10.1016/j.conb.2011.06.009
Article and author information
Author details
Publication history
Copyright
© 2018, Calabrese
This article is distributed under the terms of the Creative Commons Attribution License, which permits unrestricted use and redistribution provided that the original author and source are credited.
Metrics
-
- 1,638
- views
-
- 69
- downloads
-
- 0
- citations
Views, downloads and citations are aggregated across all versions of this paper published by eLife.
Download links
Downloads (link to download the article as PDF)
Open citations (links to open the citations from this article in various online reference manager services)
Cite this article (links to download the citations from this article in formats compatible with various reference manager tools)
Further reading
-
- Cell Biology
- Neuroscience
The assembly and maintenance of neural circuits is crucial for proper brain function. Although the assembly of brain circuits has been extensively studied, much less is understood about the mechanisms controlling their maintenance as animals mature. In the olfactory system, the axons of olfactory sensory neurons (OSNs) expressing the same odor receptor converge into discrete synaptic structures of the olfactory bulb (OB) called glomeruli, forming a stereotypic odor map. The OB projection neurons, called mitral and tufted cells (M/Ts), have a single dendrite that branches into a single glomerulus, where they make synapses with OSNs. We used a genetic method to progressively eliminate the vast majority of M/T cells in early postnatal mice, and observed that the assembly of the OB bulb circuits proceeded normally. However, as the animals became adults the apical dendrite of remaining M/Ts grew multiple branches that innervated several glomeruli, and OSNs expressing single odor receptors projected their axons into multiple glomeruli, disrupting the olfactory sensory map. Moreover, ablating the M/Ts in adult animals also resulted in similar structural changes in the projections of remaining M/Ts and axons from OSNs. Interestingly, the ability of these mice to detect odors was relatively preserved despite only having 1–5% of projection neurons transmitting odorant information to the brain, and having highly disrupted circuits in the OB. These results indicate that a reduced number of projection neurons does not affect the normal assembly of the olfactory circuit, but induces structural instability of the olfactory circuitry of adult animals.
-
- Neuroscience
Specialized chemosensory signals elicit innate social behaviors in individuals of several vertebrate species, a process that is mediated via the accessory olfactory system (AOS). The AOS comprising the peripheral sensory vomeronasal organ has evolved elaborate molecular and cellular mechanisms to detect chemo signals. To gain insight into the cell types, developmental gene expression patterns, and functional differences amongst neurons, we performed single-cell transcriptomics of the mouse vomeronasal sensory epithelium. Our analysis reveals diverse cell types with gene expression patterns specific to each, which we made available as a searchable web resource accessed from https://www.scvnoexplorer.com. Pseudo-time developmental analysis indicates that neurons originating from common progenitors diverge in their gene expression during maturation with transient and persistent transcription factor expression at critical branch points. Comparative analysis across two of the major neuronal subtypes that express divergent GPCR families and the G-protein subunits Gnai2 or Gnao1, reveals significantly higher expression of endoplasmic reticulum (ER) associated genes within Gnao1 neurons. In addition, differences in ER content and prevalence of cubic membrane ER ultrastructure revealed by electron microscopy, indicate fundamental differences in ER function.