Cerebellum: Sensing how to balance
Keeping your head upright may seem like a trivial task, but the neural circuitry required to perform this task is rather complex and not fully understood. This circuitry starts with the vestibular system: a sensory system in the inner ear that relies on hair cells to detect movements, and to provide our sense of balance and spatial awareness. The vestibular system contains five organs that are sensitive to different types of movement. The sacculus and the utricle detect gravity and linear movements, respectively, and there are three semi-circular canals that detect rotation. Information about these movements is sent from the vestibular system to the cerebellum, which co-ordinates the motor movements needed to maintain posture and balance (Ito, 2006).
The hair cells in the vestibular system contact VG (vestibular ganglion) neurons, which then send sensory information along nerve cells called mossy fibers to the vestibular region of the cerebellum (Dow, 1936). The fibers that send signals directly to the cerebellum are called primary afferents, and the fibers that send signals indirectly via the brainstem nuclei (which also receive information from other sensory systems) are called secondary afferents (Maklad and Fritzsch, 2003; see Figure 1).
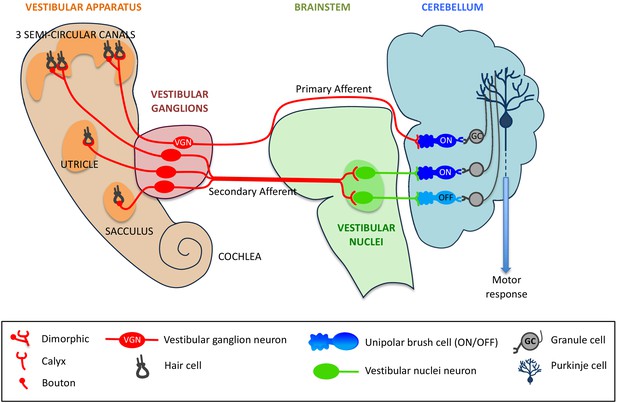
Primary and secondary afferents from the vestibular system to the cerebellum.
Neurons from the hair cells (black) within the five organs of the vestibular system (left) form different types of synapses – dimorphic, calyx or bouton – with vestibular ganglion (VG) neurons (red). Mossy fibers (also in red) can project directly from the VG neurons to the cerebellum (in which case they are called primary afferents), or indirectly via vestibular nuclei within the brainstem (secondary afferents). The primary afferents (red) form synapses with a type of unipolar brush cell (UBC) called an ON UBC, whereas secondary afferents form synapses with both ON UBCs (dark blue) and OFF UBCs (light blue). UBCs form synapses with granule cells (grey), which in turn make contact with Purkinje cells (dark blue), which convey motor responses to the rest of the body.
Both the primary and secondary afferents form synapses with neurons called granule cells in the cerebellum: granule cells are the most numerous excitatory neurons in the brain (Chadderton et al., 2004). A single mossy fiber can activate hundreds of granule cells which, in turn, form synapses with the dendrites of Purkinje cells. These cells are the sole output neurons from the cerebellar cortex and they have a crucial role in motor learning.
However, this is not the full story because the vestibular region of the cerebellum also contains a high proportion of excitatory neurons called unipolar brush cells (UBCs). These cells, which receive input from just a single mossy fiber, form synapses with the granule cells (Mugnaini et al., 2011). UBCs essentially create an intermediate step in the circuitry, where signals sent between mossy fibers and granule cells can be modified. How the signal is modified depends on the type of UBC involved: ON UBCs will have an amplified response, whereas OFF UBCs will have a dampened response (Borges-Merjane and Trussell, 2015). However, there is much about the pathways connecting the vestibular system and cerebellum that is not fully understood: for instance, how is information from the vestibular system processed once it reaches the cerebellum? Now, in eLife, Timothy Balmer and Laurence Trussell of Oregon Health and Science University report the results from experiments on genetically-modified mice that will help to answer such questions (Balmer and Trussell, 2019).
The two researchers used a combination of transgenic mice and retrograde-infecting viruses to map the morphology of the VG neurons. These experiments showed that the primary afferents largely originated at the three semi-circular canals of the vestibular system, and that the dendrites of the VG neurons mostly had a dimorphic morphology (see Figure 1). These results, combined with our current knowledge of the sensory organs of the vestibular system, led Balmer and Trussell to conclude that the primary afferents are responsible for sensing rotational movements of the head (Fernández et al., 1988).
An optogenetic approach was then employed to assess which neurons in the cerebellum were targeted by these dimorphic VG neurons. Using light to stimulate light-sensitive ion channels in VG neurons led to electric impulses being observed in UBCs in the cerebellum. The characteristics of this response were distinctive of ON UBCs, and a response could not be detected from the OFF UBCs. This finding was further bolstered by immunohistochemical staining, which showed primary afferent synapses projecting solely onto the ON UBC subtype. These data suggest that direct projections of VG neurons solely target ON UBCs, but not OFF UBCs.
Finally, Balmer and Trussell investigated the differences between the direct and the indirect pathways by expressing a light-sensitive channel in the vestibular region of the brainstem. In contrast with primary afferents, secondary afferents targeted both ON and OFF UBCs to a similar degree (see Figure 1).
The complexity of the circuitry revealed by Balmer and Trussell seems suited to the delicate task of balancing one's head, but a number of questions remain. In particular, how and where do the primary and secondary afferent pathways converge to trigger the relevant responses? An interesting follow up to this study would be to compare the role played by UBCs in maintaining balance and posture with their role in processing the other types of sensory inputs that are sent to the cerebellum.
References
-
The fiber connections of the posterior parts of the cerebellum in the rat and catThe Journal of Comparative Neurology 63:527–548.https://doi.org/10.1002/cne.900630308
-
Cerebellar circuitry as a neuronal machineProgress in Neurobiology 78:272–303.https://doi.org/10.1016/j.pneurobio.2006.02.006
-
Development of vestibular afferent projections into the hindbrain and their central targetsBrain Research Bulletin 60:497–510.https://doi.org/10.1016/S0361-9230(03)00054-6
-
The unipolar brush cell: a remarkable neuron finally receiving deserved attentionBrain Research Reviews 66:220–245.https://doi.org/10.1016/j.brainresrev.2010.10.001
Article and author information
Author details
Publication history
Copyright
© 2019, Ango and Dos Reis
This article is distributed under the terms of the Creative Commons Attribution License, which permits unrestricted use and redistribution provided that the original author and source are credited.
Metrics
-
- 21,037
- views
-
- 475
- downloads
-
- 7
- citations
Views, downloads and citations are aggregated across all versions of this paper published by eLife.
Download links
Downloads (link to download the article as PDF)
Open citations (links to open the citations from this article in various online reference manager services)
Cite this article (links to download the citations from this article in formats compatible with various reference manager tools)
Further reading
-
- Developmental Biology
- Neuroscience
Williams syndrome (WS; OMIM#194050) is a rare disorder, which is caused by the microdeletion of one copy of 25–27 genes, and WS patients display diverse neuronal deficits. Although remarkable progresses have been achieved, the mechanisms for these distinct deficits are still largely unknown. Here, we have shown that neural progenitor cells (NPCs) in WS forebrain organoids display abnormal proliferation and differentiation capabilities, and synapse formation. Genes with altered expression are related to neuronal development and neurogenesis. Single cell RNA-seq (scRNA-seq) data analysis revealed 13 clusters in healthy control and WS organoids. WS organoids show an aberrant generation of excitatory neurons. Mechanistically, the expression of transthyretin (TTR) are remarkably decreased in WS forebrain organoids. We have found that GTF2IRD1 encoded by one WS associated gene GTF2IRD1 binds to TTR promoter regions and regulates the expression of TTR. In addition, exogenous TTR can activate ERK signaling and rescue neurogenic deficits of WS forebrain organoids. Gtf2ird1-deficient mice display similar neurodevelopmental deficits as observed in WS organoids. Collectively, our study reveals critical function of GTF2IRD1 in regulating neurodevelopment of WS forebrain organoids and mice through regulating TTR-ERK pathway.
-
- Cell Biology
- Neuroscience
The assembly and maintenance of neural circuits is crucial for proper brain function. Although the assembly of brain circuits has been extensively studied, much less is understood about the mechanisms controlling their maintenance as animals mature. In the olfactory system, the axons of olfactory sensory neurons (OSNs) expressing the same odor receptor converge into discrete synaptic structures of the olfactory bulb (OB) called glomeruli, forming a stereotypic odor map. The OB projection neurons, called mitral and tufted cells (M/Ts), have a single dendrite that branches into a single glomerulus, where they make synapses with OSNs. We used a genetic method to progressively eliminate the vast majority of M/T cells in early postnatal mice, and observed that the assembly of the OB bulb circuits proceeded normally. However, as the animals became adults the apical dendrite of remaining M/Ts grew multiple branches that innervated several glomeruli, and OSNs expressing single odor receptors projected their axons into multiple glomeruli, disrupting the olfactory sensory map. Moreover, ablating the M/Ts in adult animals also resulted in similar structural changes in the projections of remaining M/Ts and axons from OSNs. Interestingly, the ability of these mice to detect odors was relatively preserved despite only having 1–5% of projection neurons transmitting odorant information to the brain, and having highly disrupted circuits in the OB. These results indicate that a reduced number of projection neurons does not affect the normal assembly of the olfactory circuit, but induces structural instability of the olfactory circuitry of adult animals.