Bacterial Protein Secretion: Looking inside an injection system
Ever since the emergence of life on earth all types of organisms have had to evolve clever strategies to cope with each other and with their changing environment. In bacteria and other micro-organisms survival often involves deploying toxins to eliminate other microbes. This can be done by using a molecular machine called the type VI secretion system (T6SS) to inject toxins into competitors (Ho et al., 2014). However, some bacteria take a different approach, releasing injection systems into the extracellular medium, from where they bind to the target organism and inject toxins or other proteins (Böck et al., 2017). Now, in eLife, two independent groups report new insights into these extracellular injection systems, which are less well understood than the T6SS.
It has been known for some time that bacteria can trigger the metamorphosis of larvae into adults in various animal species (Yang et al., 2013): for example, the marine bacterium Pseudoalteromonas luteoviolacea releases extracellular injection systems called MACs (short for metamorphosis-associated contractile structures) that trigger the metamorphosis of the tubeworm Hydroides elegans (Shikuma et al., 2014). Whereas the T6SS consists of a contractile sheath wrapped around an inner tube, a MAC array consists of about 100 contractile sheaths packed together. Upon contraction of the sheath, whatever is inside is injected into the target.
In the first paper Nicholas Shikuma (UC San Diego), Martin Pilhofer (ETH Zurich) and co-workers – including Charles Ericson and Fabian Eisenstein as joint first authors – used cryo-electron tomography to directly visualise a density in the inner tubes of a MAC array, which they propose is the toxin that is injected into targets (Ericson et al., 2019). Further experiments show that the toxin is a protein called Mif1: first, the density is not seen in bacteria in which the gene for Mif1 has been deleted; second, Mif1 co-purifies with the MAC arrays when they are prepared from the bacterial supernatant; third, electroporation of purified Mif1 into the tubeworm larvae induces metamorphosis. Together these experiments show that metamorphosis exclusively relies on Mif1 and that the MAC array is the delivery device. Other recent work by Shikuma, Pilhofer and co-workers has shown that MAC arrays can also deliver a nuclease called Pne1 that is cytotoxic to a range of eukaryotic cells (Rocchi et al., 2019).
The cryotomography images produced by Ericson et al. are notable as the cargos can be seen inside the contractile sheaths (Figure 1). Previously researchers had co-purified the antibacterial toxin Tse2 and the hexameric rings that make up the inner tube of T6SS, and had seen the toxin inside the individual rings (Silverman et al., 2013), but the latest work is the first time a toxin has been seen inside an intact injection system.
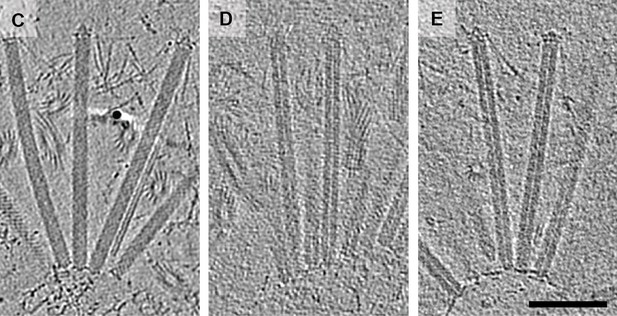
Using cryo-electron tomography to look at MACs.
Cryotomographic images of wild-type MACs (left), and two mutant MACs. It can be clearly seen that the wild-type MACs contain a cargo, whereas the mutant MACs are empty. The scale bar is 100 nanometres long.
In the second paper, Nicholas Waterfield (Warwick University), Guowei Yang (Capital Medical University) and co-workers – including Isabella Vlisidou (University Hospital of Wales) as first author – report the results of experiments on an extracellular contractile injection system called the Photorhabdus virulence cassette (PVC; Vlisidou et al., 2019). Photorhabdus is a bioluminescent bacterium with a remarkable life cycle: it establishes a symbiotic relationship with pathogenic nematodes that can penetrate into insect larvae and release Photorhabdus; in turn the bacteria produce multiple toxic compounds, including PVC devices, which kill the insect, thus providing the nematodes with a source of nutrients (McQuade and Stock, 2018). The PVC device is similar to the MAC array in that it is an extracellular injection system and is similar to T6SS in that it has just one contractile sheath.
Vlisidou et al. show that an effector toxin called Pnf (short for Photorhabdus necrosis factor) is bound to the PVC devices. Although it remains to be shown that delivery of Pnf into a target requires a functional PVC device, a clear impact on the cytoskeleton (which is a popular target for effector toxins; Lerm et al., 2000) was observed when purified Pnf protein was transfected into HeLa cells, but not when Pnf was added extracellularly. This suggests that PVC devices are needed to get the toxin inside the target.
Vlisidou et al. also show that Pnf has tranglutaminase and deamidase activity on a range of purified small GTPases, notably RhoA and Rac1, which in turn disrupts the cytoskeleton organisation through two reactions. The researchers were able to pin down this role of Pnf thanks to its homology with CNF2, a toxin released by Yersinia pseudotuberculosis (a bacterium that can cause fever in humans) that deamidates members of the Rho family (Hoffmann et al., 2004).
An outstanding question in the field of contractile injection systems – both intracellular systems like T6SS and extracellular systems like MAC and PVC – concerns how the toxins are delivered. Ericson et al. and Vlisidou et al. both show a direct association between the toxin and the contractile device, and the direct visualization of Mif1 within the inner tube of the MAC array by Ericson et al. demonstrates the potential of advanced microscopic techniques to elucidate mechanisms at molecular levels.
Working out the function of toxins and effector proteins also remains a challenge. Homology can help, as Vlisidou et al. showed with Pnf, but many effectors identified through genomic or secretome analysis have no characterised homologues. This is definitely the case for Mif1 and its role in metamorphosis.
And how do bacteria select the effectors and toxins to be loaded into these systems? The MAC array, for example, can deliver Mif1, Pne and other cargos – are they delivered simultaneously? This is an important question because the different cargos lead to very different outcomes (developmental support in the case of Mif1, toxicity for Pne). There is thus an increasingly complex love-hate relationship between organisms. All options remain open, allowing the environment to decide on the most beneficial outcome: basic Darwinian evolution, in other words.
References
-
A view to a kill: The bacterial type VI secretion systemCell Host & Microbe 15:9–21.https://doi.org/10.1016/j.chom.2013.11.008
-
The Yersinia pseudotuberculosis cytotoxic necrotizing factor (CNFY) selectively activates RhoAJournal of Biological Chemistry 279:16026–16032.https://doi.org/10.1074/jbc.M313556200
-
Bacterial protein toxins targeting Rho GTPasesFEMS Microbiology Letters 188:1–6.https://doi.org/10.1111/j.1574-6968.2000.tb09159.x
Article and author information
Author details
Publication history
- Version of Record published: September 17, 2019 (version 1)
Copyright
© 2019, Howard and Filloux
This article is distributed under the terms of the Creative Commons Attribution License, which permits unrestricted use and redistribution provided that the original author and source are credited.
Metrics
-
- 1,826
- views
-
- 137
- downloads
-
- 1
- citations
Views, downloads and citations are aggregated across all versions of this paper published by eLife.
Download links
Downloads (link to download the article as PDF)
Open citations (links to open the citations from this article in various online reference manager services)
Cite this article (links to download the citations from this article in formats compatible with various reference manager tools)
Further reading
-
- Microbiology and Infectious Disease
- Structural Biology and Molecular Biophysics
The swimming larvae of many marine animals identify a location on the sea floor to undergo metamorphosis based on the presence of specific bacteria. Although this microbe–animal interaction is critical for the life cycles of diverse marine animals, what types of biochemical cues from bacteria that induce metamorphosis has been a mystery. Metamorphosis of larvae of the tubeworm Hydroides elegans is induced by arrays of phage tail-like contractile injection systems, which are released by the bacterium Pseudoalteromonas luteoviolacea. Here we identify the novel effector protein Mif1. By cryo-electron tomography imaging and functional assays, we observe Mif1 as cargo inside the tube lumen of the contractile injection system and show that the mif1 gene is required for inducing metamorphosis. Purified Mif1 is sufficient for triggering metamorphosis when electroporated into tubeworm larvae. Our results indicate that the delivery of protein effectors by contractile injection systems may orchestrate microbe–animal interactions in diverse contexts.
-
- Microbiology and Infectious Disease
Antimicrobial resistance (AMR) poses a significant threat to human health. Although vaccines have been developed to combat AMR, it has proven challenging to associate specific vaccine antigens with AMR. Bacterial plasmids play a crucial role in the transmission of AMR. Our recent research has identified a group of bacterial plasmids (specifically, IncHI plasmids) that encode large molecular mass proteins containing bacterial immunoglobulin-like domains. These proteins are found on the external surface of the bacterial cells, such as in the flagella or conjugative pili. In this study, we show that these proteins are antigenic and can protect mice from infection caused by an AMR Salmonella strain harboring one of these plasmids. Furthermore, we successfully generated nanobodies targeting these proteins, that were shown to interfere with the conjugative transfer of IncHI plasmids. Considering that these proteins are also encoded in other groups of plasmids, such as IncA/C and IncP2, targeting them could be a valuable strategy in combating AMR infections caused by bacteria harboring different groups of AMR plasmids. Since the selected antigens are directly linked to AMR itself, the protective effect extends beyond specific microorganisms to include all those carrying the corresponding resistance plasmids.