Intraflagellar Transport: Moving proteins along in the cilium
Bardet-Biedl syndrome is a genetic disease that causes blindness, obesity and kidney anomalies (Brown and Witman, 2014; Sheffield, 2010). It arises due to mutations in more than 20 genes, eight of which encode the proteins that form a large complex known as the BBSome (Nachury et al., 2007). This complex is found in the cilia that protrude from most eukaryotic cells and have important roles in motility and signaling (Wingfield et al., 2018). It is in charge of exporting membrane proteins from the cilium to the cell body through a process known as intraflagellar transport (Lechtreck et al., 2009; Figure 1).
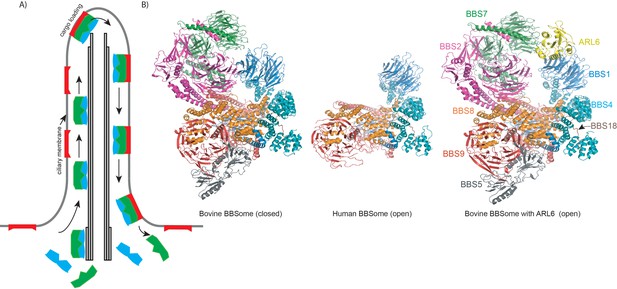
Export of ciliary signaling factors by the BBSome.
(A) Highly simplified schematic of the intraflagellar transport cycle in a cilium. The closed BBSome complex (green) binds the intraflagellar transport machinery (IFT, blue) at the base of the cilium and starts travelling towards the tip of the cilium along a structure called the axoneme (grey), which is formed by microtubules. Once near the tip, the complex binds ARL6, becoming activated, and it can take ciliary membrane proteins (red) as cargo and transport them to the base of the cilium. At this point, the BBSome releases its cargo (which remains embedded in the membrane) and dissociates from the IFT machinery. (B) Cartoon representation of closed and open conformations of bovine and human BBSome complexes with each subunit represented in a different color.
The details of how the BBSome proteins organize into a complex that can recognize signaling molecules in the cilium membrane for intraflagellar transport are not fully understood. Now, in eLife, two independent teams report how they have purified BBSome complexes and used cryo-electron microscopy to determine their structure with a resolution that reveals new insights into how they work. In the first study Alan Brown and colleagues at Harvard Medical School – including Sandeep Singh as first author – report the results of experiments on BBSomes from the bovine retina (Singh et al., 2020). In the second study, Stefan Raunser and colleagues at the Max Planck Institute of Molecular Physiology – including Björn Klink as first author – report the structure of the human BBSome (Klink et al., 2020).
The high resolution of the newly reported structures allowed the accurate visualization of amino acid side-chains in the complex, something that was not possible in a medium-resolution structure that had been published previously (Chou et al., 2019). This high resolution also enabled Singh et al. to map out the mutations reported in patients with Bardet-Biedl syndrome onto the three-dimensional structure of the BBSome, revealing that they cluster in specific regions.
The overall architecture of the human and bovine BBSomes is very similar and immediately reveals the protein interactions involved in the formation of the complex (Figure 1). Many of these interactions occur between protein domains known as β-propeller, tetratricopeptide, coil-coiled and γ-adaptin ear domains. The complex is glued together by BBS18, a mostly unstructured protein that snakes its way through the BBSome and interacts with several of the other proteins in the complex (Figure 1). Interestingly, the complex adopts both an open and a closed conformation.
Why does the BBSome adopt two different conformations? It has to be near the ciliary membrane to transport signaling factors embedded in the membrane. The complex becomes activated when it binds to ARL6, a small GTPase associated to the membrane, but to bind this protein the BBSome must be in an open conformation (Jin et al., 2010). However, the medium-resolution structure of the mouse BBSome reported previously revealed a closed conformation that was not compatible with another published structure for the BBS1-ARL6 subcomplex (Chou et al., 2019; Mourão et al., 2014). This suggests that the complex must undergo a large conformational change to accommodate ARL6 and facilitate membrane recruitment.
Singh et al. report structures for the BBSome on its own and for the complex binding ARL6, and they propose a mechanism for how it is activated. This mechanism involves the BBS1 β-propeller swiveling into a new position and the β-sheet between the BBS1 and the BBS2 subunits being broken. Many of the reported mutations in patients with Bardet-Biedl syndrome are in the regions that change conformation when ARL6 binds, suggesting the mutations may interfere with BBSome activation.
The conformational change in the proposed mechanism opens up the BBSome, which likely exposes the binding site for the proteins that the complex transports. This site may recognize specific amino acid sequences in certain ciliary signaling proteins, explaining why the BBSome is selective in the proteins it transports. The way the BBSome exposes its binding site may be similar to the mechanism adopted by certain vesicle-coating complexes, and many of the proteins in the BBSome are structurally similar to the proteins in these complexes (Jin et al., 2010; Singh et al., 2020).
The human BBSome structure reported by Klink et al. is also in the open conformation. The complex has a prominent negatively-charged patch on its surface, which is likely involved in the recognition of positively-charged regions in the proteins it traffics to and from the ciliary membrane.
With the BBSome structure revealed, several open questions remain. How does the complex specifically recognize sequences in the ciliary signaling factors it transports? And how does it couple to the intraflagellar transport machinery? The work of Singh et al. and Klink et al. lays out the basis for future work to analyze the BBSome in complex with various signaling factors. This work will likely reveal why the BBSome only transports a subset of ciliary signaling factors.
References
-
The Chlamydomonas reinhardtii BBSome is an IFT cargo required for export of specific signaling proteins from flagellaThe Journal of Cell Biology 187:1117–1132.https://doi.org/10.1083/jcb.200909183
-
Structural basis for membrane targeting of the BBSome by ARL6Nature Structural & Molecular Biology 21:1035–1041.https://doi.org/10.1038/nsmb.2920
-
The blind leading the obese: the molecular pathophysiology of a human obesity syndromeTransactions of the American Clinical and Climatological Association 121:172–182.
-
Trafficking of ciliary membrane proteins by the intraflagellar transport/BBSome machineryEssays in Biochemistry 62:753–763.https://doi.org/10.1042/EBC20180030
Article and author information
Author details
Publication history
- Version of Record published: February 13, 2020 (version 1)
Copyright
© 2020, Petriman and Lorentzen
This article is distributed under the terms of the Creative Commons Attribution License, which permits unrestricted use and redistribution provided that the original author and source are credited.
Metrics
-
- 988
- views
-
- 146
- downloads
-
- 3
- citations
Views, downloads and citations are aggregated across all versions of this paper published by eLife.
Download links
Downloads (link to download the article as PDF)
Open citations (links to open the citations from this article in various online reference manager services)
Cite this article (links to download the citations from this article in formats compatible with various reference manager tools)
Further reading
-
- Structural Biology and Molecular Biophysics
Bardet-Biedl syndrome (BBS) is a currently incurable ciliopathy caused by the failure to correctly establish or maintain cilia-dependent signaling pathways. Eight proteins associated with BBS assemble into the BBSome, a key regulator of the ciliary membrane proteome. We report the electron cryomicroscopy (cryo-EM) structures of the native bovine BBSome in inactive and active states at 3.1 and 3.5 Å resolution, respectively. In the active state, the BBSome is bound to an Arf-family GTPase (ARL6/BBS3) that recruits the BBSome to ciliary membranes. ARL6 recognizes a composite binding site formed by BBS1 and BBS7 that is occluded in the inactive state. Activation requires an unexpected swiveling of the β-propeller domain of BBS1, the subunit most frequently implicated in substrate recognition, which widens a central cavity of the BBSome. Structural mapping of disease-causing mutations suggests that pathogenesis results from folding defects and the disruption of autoinhibition and activation.
-
- Structural Biology and Molecular Biophysics
Roco proteins entered the limelight after mutations in human LRRK2 were identified as a major cause of familial Parkinson’s disease. LRRK2 is a large and complex protein combining a GTPase and protein kinase activity, and disease mutations increase the kinase activity, while presumably decreasing the GTPase activity. Although a cross-communication between both catalytic activities has been suggested, the underlying mechanisms and the regulatory role of the GTPase domain remain unknown. Several structures of LRRK2 have been reported, but structures of Roco proteins in their activated GTP-bound state are lacking. Here, we use single-particle cryo-electron microscopy to solve the structure of a bacterial Roco protein (CtRoco) in its GTP-bound state, aided by two conformation-specific nanobodies: NbRoco1 and NbRoco2. This structure presents CtRoco in an active monomeric state, featuring a very large GTP-induced conformational change using the LRR-Roc linker as a hinge. Furthermore, this structure shows how NbRoco1 and NbRoco2 collaborate to activate CtRoco in an allosteric way. Altogether, our data provide important new insights into the activation mechanism of Roco proteins, with relevance to LRRK2 regulation, and suggest new routes for the allosteric modulation of their GTPase activity.