Neurogenesis: Delayed gratification in the adult brain
The history of adult neurogenesis – the ability of the developed brain to generate new neurons – is somewhat convoluted. In ground-breaking experiments at MIT in the early 1960s, Joseph Altman and Gopal Das injected thymidine that had been labeled with tritium into the brains of young rats: when labeled thymidine was later observed in neurons, the obvious explanation was that these neurons had been generated by the adult brain (Altman and Das, 1965). Altman and Das had speculated that ‘some’ progenitor cells must have been responsible, but it was only with the discovery of neural stem cells in the early 1990s that the origins of adult neurogenesis became clear (Cameron et al., 1993; Gage, 2000).
Many fish and birds can form new neurons from stem cells in their entire brain and throughout their life (Kaslin et al., 2008), but mammals seem to lack this ability – or have only limited potential to do so. The reduced regenerative capacity that might arise from this has been interpreted as the price that mammals pay for the unsurpassed complexity of the neocortex.
Neuronal development in the embryo is mainly a preset process that is defined by decisive cell divisions at the stem cell level. However, extrinsic regulation and behavioral activity have extensive influence in the adult brain, and control adult neurogenesis in mammals at many levels. But what if new neurons could also develop from other cells within the neurogenic lineage, such as dormant intermediate cells waiting to become activated? A similar mechanism can, for example, be found in human oocytes, where germ cell proliferation ceases well before sexual maturity and further development originates from dormant cells many years later.
Now, in eLife, Luca Bonfanti and colleagues – including Chiara La Rosa as first author – report new insights into this theory by describing immature neurons in the cortex of different mammals (La Rosa et al., 2020). Although first classified in 2008, we still know little about these cortical immature neurons, especially about their functional relevance (Gómez-Climent et al., 2008; Bonfanti and Nacher, 2012). These cells are created before birth but seem to be arrested in a state that lacks the final leap in maturation required for full functionality. It is thought that they could serve as a dormant reservoir of cells beyond the proliferative precursor cell stage, and as a delayed starting point for neurogenesis dissociated from stem cell division. Neurogenesis without cell division would presumably be faster and safer than the risky process of proliferation followed by differentiation (which requires many checkpoints in its early stages).
The researchers – who are based at the University of Turin and other research institutes in Italy, Spain, Switzerland, the UK and the US – studied the distribution and properties of cortical immature neurons in 80 brains across a phylogenetically diverse range of mammal species. They discovered that the larger the brain, the greater the number of immature neurons in the second cortical layer just below the brain surface (and thus far away from the niche for precursor cells near the ventricular wall). Moreover, brains with more folds contained greater densities of immature neurons across their neocortex (Figure 1).
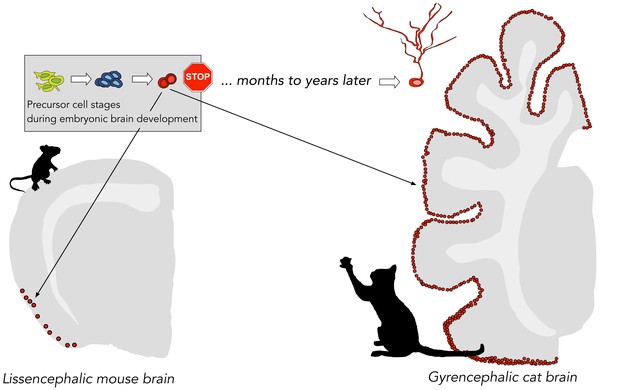
Distribution of immature cortical neurons in different mammals.
A particular type of adult neurogenesis in mammals is thought to take place in the cortex through a reservoir of immature cortical neurons, which are created before birth (green, blue and red cells) and remain dormant (indicated by the stop sign) until their maturation into functional neurons might be activated many years later. La Rosa et al. discovered that mammals with larger brains and denser brain folds (e.g., a cat) have more immature cortical neurons than mammals with smaller brains (e.g., a mouse).
La Rosa et al. suggest that these cells provide a reservoir of undifferentiated neurons, which may represent a mechanism for plasticity that varies among mammalian species. Immature cortical neurons could be adaptable and help maintain cognitive processes throughout life. Indeed, previous studies in adult mice revealed that immature neurons in the piriform cortex showed signs of both immaturity and synaptic plasticity (Klempin et al., 2011). Their further development remains to be proven, but is a fascinating hypothesis and has interesting implications for the role of brain plasticity in health and disease.
Dormant immature neurons could also play a role in adult neurogenesis of the human hippocampus (Kempermann et al., 2018), which shares many similarities in learning and memory with its rodent counterpart, but might show different dynamics in adult neurogenesis. Consequently, adult mammals with large brains – especially humans – might not necessarily lack adult neurogenesis (or have very low levels of it); they may simply delay the maturation of new neurons for decades. In theory, this process could allow quicker adaptive responses than adult neurogenesis, which has to be stimulated at the stem cell level.
The study of La Rosa et al. supports such speculations, inviting further research on the exact nature of these immature neurons: How do they maintain their supposedly dormant state? How do they end this state, if ever? And what is their ultimate fate and function? Maybe they are not as dormant as we are tempted to think?
References
-
Autoradiographic and histological evidence of postnatal hippocampal neurogenesis in ratsThe Journal of Comparative Neurology 124:319–335.https://doi.org/10.1002/cne.901240303
-
Proliferation, neurogenesis and regeneration in the non-mammalian vertebrate brainPhilosophical Transactions of the Royal Society B: Biological Sciences 363:101–122.https://doi.org/10.1098/rstb.2006.2015
Article and author information
Author details
Publication history
- Version of Record published: July 21, 2020 (version 1)
Copyright
© 2020, Kempermann
This article is distributed under the terms of the Creative Commons Attribution License, which permits unrestricted use and redistribution provided that the original author and source are credited.
Metrics
-
- 1,885
- views
-
- 164
- downloads
-
- 4
- citations
Views, downloads and citations are aggregated across all versions of this paper published by eLife.
Download links
Downloads (link to download the article as PDF)
Open citations (links to open the citations from this article in various online reference manager services)
Cite this article (links to download the citations from this article in formats compatible with various reference manager tools)
Further reading
-
- Biochemistry and Chemical Biology
- Neuroscience
Amyloid β (Aβ) peptides accumulating in the brain are proposed to trigger Alzheimer’s disease (AD). However, molecular cascades underlying their toxicity are poorly defined. Here, we explored a novel hypothesis for Aβ42 toxicity that arises from its proven affinity for γ-secretases. We hypothesized that the reported increases in Aβ42, particularly in the endolysosomal compartment, promote the establishment of a product feedback inhibitory mechanism on γ-secretases, and thereby impair downstream signaling events. We conducted kinetic analyses of γ-secretase activity in cell-free systems in the presence of Aβ, as well as cell-based and ex vivo assays in neuronal cell lines, neurons, and brain synaptosomes to assess the impact of Aβ on γ-secretases. We show that human Aβ42 peptides, but neither murine Aβ42 nor human Aβ17–42 (p3), inhibit γ-secretases and trigger accumulation of unprocessed substrates in neurons, including C-terminal fragments (CTFs) of APP, p75, and pan-cadherin. Moreover, Aβ42 treatment dysregulated cellular homeostasis, as shown by the induction of p75-dependent neuronal death in two distinct cellular systems. Our findings raise the possibility that pathological elevations in Aβ42 contribute to cellular toxicity via the γ-secretase inhibition, and provide a novel conceptual framework to address Aβ toxicity in the context of γ-secretase-dependent homeostatic signaling.
-
- Computational and Systems Biology
- Neuroscience
Normal aging leads to myelin alterations in the rhesus monkey dorsolateral prefrontal cortex (dlPFC), which are positively correlated with degree of cognitive impairment. It is hypothesized that remyelination with shorter and thinner myelin sheaths partially compensates for myelin degradation, but computational modeling has not yet explored these two phenomena together systematically. Here, we used a two-pronged modeling approach to determine how age-related myelin changes affect a core cognitive function: spatial working memory. First, we built a multicompartment pyramidal neuron model fit to monkey dlPFC empirical data, with an axon including myelinated segments having paranodes, juxtaparanodes, internodes, and tight junctions. This model was used to quantify conduction velocity (CV) changes and action potential (AP) failures after demyelination and subsequent remyelination. Next, we incorporated the single neuron results into a spiking neural network model of working memory. While complete remyelination nearly recovered axonal transmission and network function to unperturbed levels, our models predict that biologically plausible levels of myelin dystrophy, if uncompensated by other factors, can account for substantial working memory impairment with aging. The present computational study unites empirical data from ultrastructure up to behavior during normal aging, and has broader implications for many demyelinating conditions, such as multiple sclerosis or schizophrenia.