Enzymes: The two roles of complex III in plants
Every year land plants assimilate about 120 billion tons of carbon from the atmosphere through photosynthesis (Jung et al., 2011). However, plants also rely on respiration to produce energy, and this puts about half the amount of carbon back into the atmosphere (Gonzalez-Meler et al., 2004). Mitochondria have a central role in cellular respiration in plants and other eukaryotes, harboring the enzymes involved in the citric acid cycle and the respiratory electron transport chain.
The basic functioning of mitochondria is highly conserved across evolution. In plants, however, these organelles perform additional roles linked to photosynthesis (Millar et al., 2011). In particular, under certain conditions they employ enzymes called alternative oxidoreductases, which may help to reduce the formation of reactive oxygen species (Vanlerberghe, 2013).
The mitochondrial electron transport chain is similar in plants, fungi and animals, where it is formed of four enzyme complexes – complex I, II, III and IV – as well as further components such as cytochrome c and the lipid ubiquinone. In plants, alternative oxidoreductases are also involved. The structure and function of the complexes I to IV have been extensively investigated in animals and fungi, but less so in plants. Now, in eLife, Maria Maldonado, Fei Guo and James Letts from the University of California Davis present the first atomic models of the complexes III and IV from plants, giving astonishing insights into how the mitochondrial electron transport chain works in these organisms (Maldonado et al., 2021).
For their investigation, Maldonado et al. isolated mitochondria from etiolated mung bean seedlings; the protein complexes of the electron transport chain were then purified, and their structure was analyzed using a new experimental strategy based on single-particle cryo-electron microscopy combined with computer-based image processing (Kuhlbrandt, 2014). The team used pictures of 190,000 complex I particles, 48,000 complex III2 particles (III2 is the dimer formed by complex III), and 28,000 particles of a supercomplex consisting of complexes III2 and IV. Average structures of all three types of particles were calculated with resolutions in the range of 3.5 Angstroms, which allow side chains of amino acids to become visible. Finally, the amino acid sequences of the protein subunits were fitted into the structures, generating models of the protein complex at atomic resolution. Results of this experimental approach on a large subcomplex of plant complex I have been published before, and they revealed an extra functional module that may be relevant when cellular respiration takes place alongside photosynthesis (Maldonado et al., 2020). This suggests that complex I has additional roles in plants.
Now, the atomic models for plant complex III2 and the supercomplex III2-IV indicate that these share highly conserved features with the corresponding animal and fungi structures. The plant complex IV, for instance, which had never been precisely defined so far, consists of 10 protein subunits that are all homologs of animal and fungi complex IV subunits. Similarly, plant complex III2, which is formed of a pair of 10 subunits, much resembles its animal and fungi counterparts, and performs a similar role in cellular respiration (Figure 1).
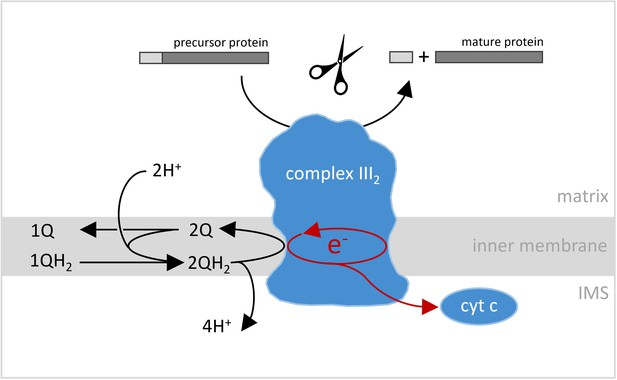
Reactions catalyzed by complex III2 in plant mitochondria.
Complex III2 is an enzyme complex embedded in the inner membrane (light gray band) that separates the matrix (top) and the inter-membrane space (IMS; bottom) in mitochondria. One of the reactions it catalyzes is an oxidoreduction, where two molecules of ubiquinol (QH2) are sequentially converted into ubiquinone (Q), two pairs of protons are released into the mitochondrial intermembrane space (IMS), and two pairs of electrons are transferred onto complex III2. Two of these electrons are sequentially transferred onto cytochrome c (cyt c), while the other two electrons are used to convert one molecule ubiquinone into ubiquinol. The latter step is linked to the uptake of two protons from the mitochondrial matrix. The overall reaction results in protons being translocated across the inner mitochondrial membrane, which is a key process in cellular respiration. However, in plants, complex III2 is also involved in another reaction: it helps mitochondrial preproteins mature by removing the import sequence necessary for their movement into the compartment (top).
Image credit: Shape of the complex III2 is adapted from Maldonado et al., 2021.
However, at the same time, complex III2 is special in plants because it also includes the activity of the mitochondrial processing peptidase (MPP for short), an enzyme which cleaves off the transit sequences that help to import certain preproteins into mitochondria (Braun et al., 1992; Eriksson et al., 1994; Figure 1). For the very first time, structural details of the two MPP subunits of plant complex III2 are presented, showing that they form a large central cavity with a negative surface that is probably essential for preprotein binding. The structural model also includes a catalytic zinc ion at the active site of the subunit which cleaves the preproteins. In addition, compared to similar protein subunits in animal or fungi complex III2, the plant MPP subunits are more stably connected to the remaining complex III2 subunits. Finally, Maldonado et al. offer further structural insights into the functioning of complex IV and supercomplex III2-IV, which provide clues as to the way their enzyme activities take place.
Overall, the bifunctionality of complex III2 in plants may be another example that mitochondria work differently in the context of photosynthesis. Indeed, the way MPP is attached to complex III reflects that the presence of chloroplasts makes it more complicated for proteins to be transported and processed within plant cells. The atomic models revealed by Maldonado et al. will help further genetic and biochemical investigations into the physiology of the mitochondrial electron transport chain of plants.
References
-
The ubiquinol cytochrome c oxidoreductase complex of spinach leaf mitochondria is involved in both respiration and protein processingBiochimica Et Biophysica Acta (BBA) - Bioenergetics 1186:221–231.https://doi.org/10.1016/0005-2728(94)90181-3
-
Organization and regulation of mitochondrial respiration in plantsAnnual Review of Plant Biology 62:79–104.https://doi.org/10.1146/annurev-arplant-042110-103857
-
Alternative oxidase: A mitochondrial respiratory pathway to maintain metabolic and signaling homeostasis during abiotic and biotic stress in plantsInternational Journal of Molecular Sciences 14:6805–6847.https://doi.org/10.3390/ijms14046805
Article and author information
Author details
Publication history
Copyright
© 2021, Braun
This article is distributed under the terms of the Creative Commons Attribution License, which permits unrestricted use and redistribution provided that the original author and source are credited.
Metrics
-
- 1,382
- views
-
- 136
- downloads
-
- 4
- citations
Views, downloads and citations are aggregated across all versions of this paper published by eLife.
Download links
Downloads (link to download the article as PDF)
Open citations (links to open the citations from this article in various online reference manager services)
Cite this article (links to download the citations from this article in formats compatible with various reference manager tools)
Further reading
-
- Microbiology and Infectious Disease
- Plant Biology
Programmed cell death occurring during plant development (dPCD) is a fundamental process integral for plant growth and reproduction. Here, we investigate the connection between developmentally controlled PCD and fungal accommodation in Arabidopsis thaliana roots, focusing on the root cap-specific transcription factor ANAC033/SOMBRERO (SMB) and the senescence-associated nuclease BFN1. Mutations of both dPCD regulators increase colonization by the beneficial fungus Serendipita indica, primarily in the differentiation zone. smb-3 mutants additionally exhibit hypercolonization around the meristematic zone and a delay of S. indica-induced root-growth promotion. This demonstrates that root cap dPCD and rapid post-mortem clearance of cellular corpses represent a physical defense mechanism restricting microbial invasion of the root. Additionally, reporter lines and transcriptional analysis revealed that BFN1 expression is downregulated during S. indica colonization in mature root epidermal cells, suggesting a transcriptional control mechanism that facilitates the accommodation of beneficial microbes in the roots.
-
- Cell Biology
- Plant Biology
Plants distribute many nutrients to chloroplasts during leaf development and maturation. When leaves senesce or experience sugar starvation, the autophagy machinery degrades chloroplast proteins to facilitate efficient nutrient reuse. Here, we report on the intracellular dynamics of an autophagy pathway responsible for piecemeal degradation of chloroplast components. Through live-cell monitoring of chloroplast morphology, we observed the formation of chloroplast budding structures in sugar-starved leaves. These buds were then released and incorporated into the vacuolar lumen as an autophagic cargo termed a Rubisco-containing body. The budding structures did not accumulate in mutants of core autophagy machinery, suggesting that autophagosome creation is required for forming chloroplast buds. Simultaneous tracking of chloroplast morphology and autophagosome development revealed that the isolation membranes of autophagosomes interact closely with part of the chloroplast surface before forming chloroplast buds. Chloroplasts then protrude at the site associated with the isolation membranes, which divide synchronously with autophagosome maturation. This autophagy-related division does not require DYNAMIN-RELATED PROTEIN 5B, which constitutes the division ring for chloroplast proliferation in growing leaves. An unidentified division machinery may thus fragment chloroplasts for degradation in coordination with the development of the chloroplast-associated isolation membrane.